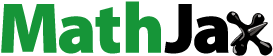
Abstract
WRKY transcription factors are involved in complex signalling processes that occur during stress responses. However, in contrast to the exploration of such mechanisms in model plants, far less research has focussed on the WRKY family in cotton. We previously identified GbWRKY1 in cotton (Gossypium barbadense), the expression of which is induced upon infection by Verticillium dahliae. Our current results indicate that this gene with three introns, has a group of cis-acting elements in its promoter that are associated with plant growth and development as well as responses to environmental stresses. Here, we used virus-induced gene silencing to characterize the role of GbWRKY1. Silencing in cotton simultaneously up-regulated salicylic acid (SA)-mediated responses and inhibited jasmonate (JA)-inducible and ethylene (ET)-inducible defences, resulting in an increased susceptibility to V. dahliae. However, when compared with wild-type Arabidopsis, transgenic plants exhibited more resistance to infection with V. dahliae. In the latter genotype, expression was increased for pathogen-related PR4 and ACS2, two genes involved in the JA pathway and ET biosynthesis, while expression of PR5, involved in the SA pathway, decreased after V. dahliae infection. Moreover, POD activity and lignin content related to ethylene synthesis in transgenic Arabidopsis were higher than those in wild-type Arabidopsis after V. dahliae infection. Collectively, these findings suggest that GbWRKY1 is regulated by signalling pathways for SA, JA and ET, and mainly involved in resistance to V. dahliae attack by ET signalling pathway the response. This highlights the importance of WRKYs in regulating pathogen-induced responses and plant development.
Introduction
Cotton (Gossypium spp.) is one of the most economically important crops worldwide and an excellent source of fibre and oil. Its growth is severely inhibited under various biotic-stress and abiotic-stress conditions, such as pathogen attacks, insect herbivory, wounding and extreme temperatures. Verticillium wilt, caused by Verticillium dahliae Kleb., is one of the most serious diseases in cotton. Timely transcriptional regulation of defence-related genes is crucial for effective responses to pathogens [Citation1]. Transcription factors (TFs) are central to this process because they activate or restrain the transcription of downstream defence-related genes [Citation2]. Those TFs include members of the WRKY family, which are involved in disease resistance [Citation3,Citation4]. Since the first member was isolated in Ipomoea batatas, several more have been identified, including 74 members in Arabidopsis thaliana and 109 members in Oryza sativa [Citation5,Citation6]. Most of the WRKY TFs in cotton have been identified in Upland varieties [Citation7–11]. Except for GbWRKY1, the roles of WRKY transcription in conferring tolerance or modulating the response to biotic stresses have not been characterized for G. barbadense [Citation12].
The WRKY proteins recognize the W-box sequence that exists in the promoter of pathogen-related (PR) genes [Citation13]. Therefore, WRKY TFs might play an important role in regulating defence responses to pathogen attack and they may also be part of a complex hormone-signalling network. For example, constitutive overexpression of WRKY70 results in an increased resistance to pathogens and the constitutive expression of salicylic acid (SA)-induced PR genes [Citation14]. Similarly, constitutive overexpression of WRKY18 promotes the expression of SA-related PR1 and enhances resistance to Pseudomonas syringae, while its co-expression with WRKY40 or WRKY60 results in increased growth of the bacterial pathogen [Citation15,Citation16]. In cotton, Ethylene biosynthesis and response genes have been identified from resistant cotton cultivars (G. barbadense cv. ‘7124’ or ‘Pima 90’) through suppression subtractive hybridization (SSH), which plays a crucial role in the defence against the invasion of pathogens [Citation17]. It has been reported that WRKY transcription factors are involved in the invasion of pathogens by participating in ethylene signalling. For example, GhWRKY15 can improve tolerance to fungal pathogens in transgenic tobacco plants by participating in SA-signalling and ethylene (ET)-signalling pathways [Citation9]. In Nicotiana benthamiana, overexpression of GhWRKY40 alters plant responses to wounding and infection of the bacterium Ralstonia solanacearum by involving complex hormone-signalling network [Citation8]. Taken together, these reports demonstrate that WRKY TFs have key roles in hormone-signalling and abiotic-stress signalling networks.
Continued investigations with WRKYs can provide new insight that might assist with creating plants that are better able to resist stress. However, a large number of related studies mainly focus on model plants such as A. thaliana, but there is far less information about the function of WRKY protein in cotton [Citation8]. In our previous study, we isolated and characterized GbWRKY1, a Group I WRKY gene from G. barbadense. Its expression was up-regulated when cotton plants were inoculated with V. dahliae. We also noted that the genes related to the synthesis of 1-aminocyclopropane-1-carboxylic acid (ACC) and methyl jasmonate (MeJA) were down-regulated in plants treated with SA [Citation12]. In the research presented here, we utilized a virus-induced gene silencing (VIGS) approach to characterized four classes of genes involved in SA, JA and ET signalling. We also examined how overexpression of GbWRKY1 can be used to alter the response of A. thaliana plants to infection with V. dahliae. Our findings suggest that SA, JA and ET act as important factors in making cotton resistant to V. dahliae and overexpression of GbWRKY1 can enhance disease resistance in Arabidopsis. Moreover, a change in the disease response in those overexpressing plants might be related to multiple defence signalling pathways, such as those for SA, JA and ET.
Materials and methods
Plant materials
Seeds of G. barbadense cv. Pima90-53, a cultivar that is highly resistant to V. dahliae, were used to produce cotton plants for sequence analysis. To obtain transgenic receptor materials, we also used seeds and seedlings of A. thaliana ecotype Columbia (wild-type ‘Col’, WT).
Cloning the GbWRKY1 genomic sequence and its promoter
Genomic DNA was isolated from cotton seedling leaves by a modified CTAB method [Citation18]. One pair of primers (F1: 5′-ATGGGTGAAAGCCAAGAGCAGAAG-3′ and R1: 5′-GCGGTCACTGCATTCTGCATTCTTG-3′) was designed and synthesized based on the full-length GbWRKY1 cDNA, which was used to amplify the GbWRKY1 sequence with genomic DNA as the template. The full-length GbWRKY1 was used for locating gene sequence positions in the published cotton genomic sequence (http://www.phytozome.net). The primer pair F2 (5′-AACACTCTTTCCCTCCTTCTGCCC-3′) and R2 (5′-GATGGCTTCTGCTCTTGGCTTTC-3′) was designed for amplifying the promoter sequence, which was then analyzed by the Plant-CARE database programme (http://bioinformatics.psb.ugent.be/webtools/plantcare/html).
Virus-induced gene silencing (VIGS) in cotton followed by pathogen inoculation
The tobacco rattle virus (TRV) vectors and Agrobacterium tumefaciens for VIGS were prepared as described previously [Citation19]. Inserts to generate TRV:GbWRKY1 and the positive control TRV:GbCLA1 (Cloroplastos Alterados 1) were amplified from the cDNA of G. barbadense cv. Pima90-53. The PCR fragments were digested with BamHI and KpnI and then ligated into the TRV:00 plasmid. Constructs were transformed into A. tumefaciens strain GV3101. Those TRV vectors were agro-infiltrated [Citation20] into the cotyledons of 5-day-old ‘Pima90-53’ seedlings, which were then grown in a controlled environment chamber (25 °C; 16 h photoperiod). We observed a leaf bleaching phenotype 2 weeks after the TRV:GbCLA1 plants were infiltrated. Leaves were collected from TRV:GbWRKY1 and the TRV:00 plants, frozen in liquid nitrogen and stored at −80 °C prior to RNA isolation. Some of those plants were also inoculated with V. dahliae before a disease index (DI) was scored for at least 16 plants of TRV:GbWRKY1 and TRV:00 plants. All experiments were conducted at least three times. The plant DI was calculated by the following formula:
(1)
(1)
where n denotes the numerical disease rating according to a scale ranging from 0 (no symptoms) to 4 (severe; 75–100% chlorotic leaves), based on the method described by Xu et al. [Citation21]. Values for DI reflected the infection status of an entire population rather than individual plants.
RNA isolation, reverse transcription-PCR (RT-PCR), and quantitative real-time PCR (qPCR)
Total RNA was extracted from the cotton leaves and cDNA synthesis was conducted with UEIris II RT-PCR system for First-Strand cDNA Synthesis. For RT-PCR, an aliquot of the reverse-transcription product was used as a template, Ubiquitin7 (DQ116441) from cotton was used as an internal control and the following primers were designed and synthesized: F3 (5′-GACTCTACTCAATCCCCACCAGCCT-3′) and R3 (5′-CCGCACCTTAGCCGACTACAACATC-3′). The control GbUB7 and genes involved in SA, JA and ET signalling pathways were analyzed by qRT-PCR with SYBR Green Mix (Takara) and the Roche LightCycler 2.0 (Roche, Germany). Those genes included GbPR5, GbPR1, GbNPR1, GbPR4, GbAOS, GbMYC2, GbJaz1, GbACS, GbAMS. All primers were synthesized as described previously [Citation22]. The qRT-PCR data obtained from three replicate experiments were evaluated by the 2−ΔΔCT method [Citation23].
Production of transgenic Arabidopsis plants
The open reading frame of GbWRKY1 was cloned into overexpression vector pCamE after PCR amplification with primer pair F4 (5′-GGATCCATGGGTGAAAGCCAAGAGCAGAAG-3′; BamHI restriction site) and R4 (5′-GGTACCGCGGTCACTGCATTCTGCATTCTTG-3′; KpnI restriction site). Binary vector pCamE-GbWRKY1 was then inserted into A. tumefaciens strain GV3101 and used to transform WT Arabidopsis plants by the floral-dip method [Citation24].
Molecular characterization of putative transgenic plants
Transgenic Arabidopsis lines were screened on a quarter-strength Murashige and Skoog (MS) medium containing 40 mg L−1 of hygromycin. After PCR amplification and DNA sequencing, positive expression of GbWRKY1 in those transgenics was verified via RT-PCR, which was performed with cDNA from the transformed and WT plants. The following specific primers were used for PCR amplification: F5 (5′-TTCTCAATTACTGGTCGGTGC-3′) and R5 (5′-GACAATCAATGAAGCAGGTTGG-3′).
Southern blotting was performed according to standard procedures [Citation25]. Genomic DNA samples (20 μg) were digested for 24 h at 37 °C with HindIII enzymes. After fractionation by 1.0% agarose gel electrophoresis, the samples were transferred to a Hybond Nylon membrane (Amersham Pharmacia, USA) by capillary blotting. A gene-specific probe (495 bp) from the partial coding region of GbWRKY1 cDNA was prepared using Primers F5 and R5. Standard procedures were followed for probe-labelling and Southern blot analysis, including the use of a DIG DNA Labeling and Detection Kit II (Roche, Germany).
Assessment of growth performance by roots from Arabidopsis plants
Seeds from WT and transgenic Arabidopsis plants were surface-sterilized for 15 min in 10% (v/v) bleach and a 0.01% (v/v) Triton X-100 solution, then washed briefly in 70% (v/v) ethanol and rinsed four or five times in sterile distilled water. After cold treatment (4 °C) for 2 days, the seeds were individually pipetted out in a single row at the top of 10-cm2 Petri dishes containing 75 mL of 1/2 MS agar. The resultant seedlings were grown at 24 °C under a 16-h light/8-h dark regime and a light intensity of approximately 65 μmol m−2 s−2.
Examination of parameters associated with disease resistance
Previously vernalized Arabidopsis seeds from the WT and transgenic lines were germinated on Hoagland solution-saturated vermiculite in a growth chamber (24 °C, 16-h photoperiod and 80% relative humidity). After 3 weeks, the roots from those seedlings were damaged with iron wire. A fresh spore suspension (1.9 × 107 conidia mL−1) of highly aggressive V. dahliae strain T5 (5 mL) was injected into a plastic bowl containing Arabidopsis plants, while control seedlings were mock-treated with sterile water only. All plants were irrigated regularly with Hoagland’s nutrient solution and received standard care. Disease development was monitored for up to 4 weeks post-inoculation. The calculation method of plant DI is the same as above. Parameters used for assessing disease severity included the extent of leaf chlorosis, inflorescence heights and fresh weights of the aboveground portions.
Expression analysis of defence-related genes in transgenic and WT lines of Arabidopsis
Expression of genes in the SA, JA and ET signalling pathways was analyzed by qPCR protocols, using the following primers: for AtPR4, U01880 (5′-GCAGTAACTGTGAGAATAGT-3′ and 5′-GCTGCCCAATGGCTCATTGC-3′); for AtPR5, NM_106161 (5′-ATGTGAGCCTCGTAGATGGTTAC-3′ and 5′-GATCCATGACCTTAAGCATGTG-3′); for EF1a, At5g60390 (5′-TGAGCACGCTCTTCTTGCTTTCA-3′ and 5′-GGTGGTGGCATCCATCTTGTTACA-3′); and for AtACS2, LG01480 (5′-GGATGGTTTAGGATTTGCTTTG-3′ and 5′-GCACTCTTGTTCTGGATTACCTG-3′) [Citation26].
Microscopic observation of lignin and determination of peroxidase (POD) content in transgenic and WT lines of Arabidopsis after inoculation with V. dahliae
The stem base of WT and transgenic Arabidopsis were sliced after 15 days of inoculation with V. dahliae. The stem sections were immersed in 2% phloroglucinol solution for 10 min and afterwards – in 18% HCl solution for 5 min. The results of lignin staining were observed under optical microscope. The activities of POD was measured by the guaiacol oxidation method. The reaction system: 3 mL 10 mmol/L phosphate buffer (pH 7.0), 1 mL 20 mmol/L guaiacol, 1 mL 40 mmol/L H2O2, 2 mL enzyme extract.
Results and discussion
Sequence analysis of GbWRKY1
We amplified the sequence for GbWRKY1 from the genomic DNA of G. barbadense and produced a 1938-bp fragment. When its genomic and cDNA sequences were compared, we noted three introns with lengths of 87, 92 and 289 bp (). Each had the typical characteristics of a plant intron with high AT contents (67.1% for Intron 1, 67.7% for Intron 2 and 66.7% for Intron 3), plus conserved 5′-GT and AG-3′ splicing sites. Comparisons among GbWRKY1, GhWRKY3, AtWRKY3 and VvWRKY2 revealed that the number and position of introns were well-conserved. Moreover, each of their third intron is an R-type intron that is located in the WRKY domain and inserted between AG and G (the codon of arginine) [Citation27]. Although they are located in the C-terminal WRKY domain, their N-terminal WRKY domains lack introns. This suggests that the conserved intron splicing site in the WRKY domain has an important role in transcription or evolution.
Analysis of partial putative cis-acting elements in the GbWRKY1 promoter
A 1762-bp fragment of the GbWRKY1 promoter contained several pathogen-/elicitor-related elements, e.g. RAV1AAT, ARE, W-box, CGTCA-motif, ERE and TGACG-motif; abiotic stress-responsive elements such as HSE, TC-rich repeats, WRKY71OS, WBOXNTERF3 and MYCATERD1; tissue-specific and development-related elements, including CAT-box, CCGTCC-box, GARE-motif and GCN4_motif; and light-responsive elements such as GA-motif and AE-box (). These results suggested that, based on the cis-elements found in its promoter region, the WRKY gene is related to disease resistance.
Table 1. Putative cis-acting elements occurring in the promoter of GbWRKY1.
GbWRKY1 influences the level of disease resistance in cotton by altering SA-mediated and JA-mediated defence signalling
We applied VIGS protocols to improve our understanding of how GbWRKY1 functions in the defence response by ‘Pima90-53’ cotton. Two weeks after Agrobacterium infiltration, the TRV:GbCLA1 plants showed a leaf bleaching phenotype (). At this point, transcripts of GbWRKY1 were significantly reduced in TRV:GbWRKY1 plants when compared with TRV:00 plants as demonstrated through RT-PCR analysis (). This indicated that the gene was effectively silenced. Upon inoculation with defoliating V. dahliae strain T5, leaves of the TRV:GbWRKY1 plants showed more wilting and etiolation when compared with the control (). The higher proportion of diseased plants and greater DI values indicated that the lack of GbWRKY1 expression compromised the resistance to V. dahliae (). Expression of GbNPR1, GbPR1 and GbPR5, three important genes involved in the SA-signalling pathway, was analyzed by qPCR; transcripts of all three were up-regulated after GbWRKY1 was silenced (). To explore whether activation of the SA pathway in TRV:GbWRKY1 plants influences JA signal transduction, we analyzed the expression of genes that function in JA biosynthesis and signal transduction. Our qRT-PCR results indicated that expression of GbAOS (for JA biosynthesis) and GbJAZ1, GbMYC2 and GbPR4 (for JA signal transduction) was inhibited in TRV:GbWRKY1 plants (). Moreover, expression of two genes for ET biosynthesis, GbACS and GbAMS, was down-regulated after GbWRKY1 was silenced ().
Figure 2. Silencing of GbWRKY1 in cotton by TRV-mediated VIGS. (A) Seedlings of G. barbadense ‘Pima90-53’, hand-infiltrated with Agrobacterium carrying VIGS-vector control – TRV:00 (left) or TRV:GbCLA1 (right). (B) Disease symptoms induced in empty-vector control (TRV:00) or GbWRKY1-silenced (TRV:GbWRKY1) cotton plants after inoculation with V. dahliae strain T5 10 days post-inoculation and RT-PCR analysis of GbWRKY1 after infiltration. (C and D) Ratings for diseased plants and DI values for control (TRV:00) and GbWRKY1-silenced (TRV:GbWRKY1) cotton plants, respectively, after inoculation with conidial suspensions. Error bar represents standard deviation of 3 biological replicates (n ≥ 16).
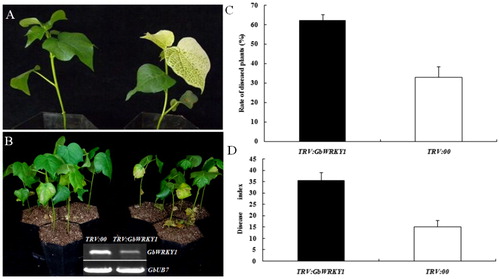
Figure 3. Detection of genes related to signalling pathways for SA (A), JA (B) and ET (C) in TRV:00 and TRV:GbWRKY1 plants. Note. Error bar represents standard deviation for 3 independent experiments; three technical replicates were used in qRT-PCR analysis.
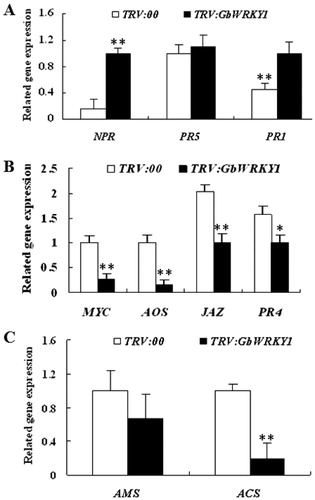
Phytohormones are implicated in complex signalling pathways and are crucial for regulating developmental processes and plant responses to a variety of environmental stresses [Citation28]. The three key signalling molecules for disease-induced defences are SA, JA and ET [Citation29]. Furthermore, the SA and JA signalling pathways are mutually antagonistic and the latter usually acts with ET to induce resistance against necrotrophic pathogens [Citation30]. We previously found that GbWRKY1 is involved in SA and JA/ET crosstalk to regulate plant defences against V. dahliae [Citation12]. The results obtained from this study found that silencing of GbWRKY1 in cotton through VIGS simultaneously up-regulated SA-mediated responses and inhibited JA- and ET-inducible defences, resulting in increased susceptibility to that pathogen. It is consistent with the previous research results.
Molecular analysis of GbWRKY1 and evaluation of Verticillium resistance in transgenic Arabidopsis plants
From the shoots of Arabidopsis that survived on the hygromycin selection medium, we chose seven well-developed plantlets and transferred them to a vermiculite substrate. Their DNA samples were used in PCR analysis to detect GbWRKY1. Among all of the T3 transgenic Arabidopsis plants produced here, five lines gave the expected product sizes (). Genomic DNA from four randomly selected PCR-verified plants was analyzed by Southern blots after digestion with HindIII, which did not cut the GbWRKY1 cassette. While Line 5 exhibited two bands, each of the other four had only one band that suggested the transgene had a single locus (). The number of insertions and their sizes differed among plants, confirming the independent origin of those transgenic lines. Expression of GbWRKY1 in the positive transformants was examined through RT-PCR analysis. Its transcripts were detected only in the transgenic lines (), indicating that GbWRKY1 was correctly expressed.
Figure 4. Molecular analysis of GbWRKY1-transformed Arabidopsis and non-transformed plants. (A) Schematic representation of recombinant plasmid PCamE35S-GbWRKY1-hptII. R and L indicate right and left borders of T-DNA. (B) PCR-screening of GbWRKY1. PCR product (495 bp) was amplified from transgenic plants and plasmid (P), but not from WT. M, DNA marker DL2000. (C) Southern blot analysis of GbWRKY1 in transgenic lines and WT. Genomic DNA (20 μg) was digested with HindIII and hybridized against DIG-labeled GbWRKY1 probe. M, DNA Molecular Weight Marker II (Roche). Transgenic lines L1, L2, L4 and L5 of T3 progeny were initially tested. (D) RT-PCR analysis of GbWRKY1 expression in transgenic Arabidopsis lines L1, L2 and L4.
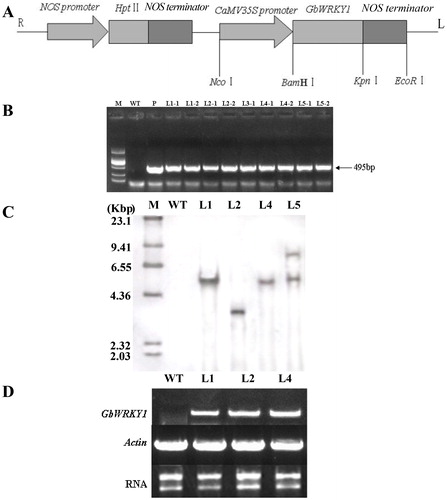
Disease resistance was tested on T3 progeny from the three transgenic lines and the WT Arabidopsis. When inoculated with spore suspensions of V. dahliae, three-week-old transgenic plants proved to be more resistant than the WT plants to infection (). The survey results about disease index and rate of diseased plants showed the same results (). To illustrate the level of damage caused by this pathogen, we scored the presence of lesions, recorded inflorescence heights and plant fresh weights. These data were consistent with our finds (). For the WT, inflorescences were 47.5% shorter from the inoculated plants than from the mock control and fresh weights were 44.1% lower for the former type. In contrast, the inflorescences from the three inoculated transgenic lines were only 24.12–26.81% shorter than the control while their fresh weights were just 26.86–30.29% lower. Based on the extent of development for these symptoms, we could conclude that transgenic Arabidopsis had significantly improved resistance to V. dahliae.
Figure 5. Determination of resistance by transgenic Arabidopsis to V. dahliae. (A) Transgenic lines and non-transformed plants showed obvious differences 2 weeks after inoculation with mock control (H2O only) or spore suspensions (1.9 × 107 conidia mL−1). (B and C) Ratings for diseased plants and DI values for WT Arabidopsis and transgenic Arabidopsis.
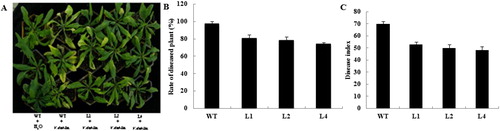
Table 2. Level of resistance by Arabidopsis wild-type ‘Col’ and GbWRKY1-transgenic lines to Verticillium dahliae.
Effects of GbWRKY1 overexpression on the expression of PR genes and genes related to ethylene biosynthesis
We previously reported that expression of GbWRKY1 is enhanced to varying degrees by MeJA, ACC and V. dahliae infection and suppressed under SA treatment [Citation12]. To assess more thoroughly the possible mechanisms underlying the improvement in resistance to that pathogen by transgenic plants, we examined the transcript levels of PR genes and monitored the activity of an enzyme involved in SA/JA signalling pathways and ET biosynthesis relative to the expression of EF1a (). Following infection with V. dahliae, expression of PR4 and ACS2 was higher in transgenic lines than in the WT plants, peaking in those genotypes at 72 h and 24 h, respectively (). However, transcription of PR5 was inhibited in the transgenic plants when compared with the WT ().
Figure 6. Expression of defence-related PR4 (A), PR5 (B) and ET biosynthesis-related ACS2 (C) after infection with V. dahliae. Total RNA was isolated at indicated time points from transgenic Arabidopsis and WT plants. EF1a was used as internal standard for purposes of normalization.
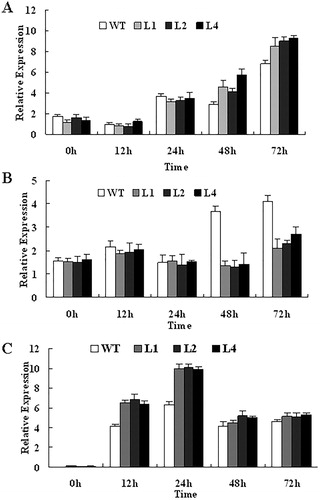
The WRKY DNA-binding proteins can regulate the transcription of several defence-related genes, including PR genes, by binding to the W-box in their promoters [Citation31]. Thus, PR4 and PR5 are often used as molecular markers for the JA-signalling and SA-signalling pathways, respectively [Citation22]. We noted that the GbWRKY1-expressing Arabidopsis plants were more resistant than the WT to V. dahliae infection. Moreover, infection of transgenic plants increased the expression of PR4, which is often used as a molecular marker for JA. Concurrently, expression of PR5, a marker for SA, was down-regulated during V. dahliae infection to a greater extent in the transgenics. Ethylene is a plant stress hormone, and acetyl-CoA synthetase (ACS) is believed to be the committed and generally, rate-limiting enzyme in ET biosynthesis [Citation32,Citation33]. When plants are infected by pathogens, ethylene production increases dramatically, which is involved in plant stress response process [Citation34]. In Arabidopsis, ACS2 and ACS6 are phosphorylated and stabilized by MPK3 and MPK6, two pathogen-responsive MAPKs [Citation35]. We also found here that expression of ACS2 increased in GbWRKY1 transgenic plants after Verticillium infection. All of these findings indicate that GbWRKY1 plays a role in resistance to fungal pathogens via SA-dependent and JA/ET-dependent defence pathways.
Effects of GbWRKY1 overexpression on the plant growth, POD and lignin content
To investigate the effects of constitutive overexpression of GbWRKY1 on plant growth, we monitored the phenotype of both WT plants and transgenic Arabidopsis lines. On Days 3, 6, 9 and 12 after germination, the roots were longer and stronger from the transgenics than from the WT (). These results indicated that GbWRKY1 promotes root development and overall plant growth, thereby enhancing disease resistance. We further observed the effect of constitutive overexpression of GbWRKY1 on POD and lignin content in WT and transgenic Arabidopsis lines. The POD activity increased significantly after infection by V. dahliae in WT and transgenic Arabidopsis and reached its peak at 24 h. However, the POD activity of transgenic Arabidopsis was significantly higher than that of WT Arabidopsis (). Similarly, the lignin content of transgenic Arabidopsis was significantly higher than that of wild type ().
Figure 7. Phenotypes of root growth, POD content and staining of lignin for transgenic and WT Arabidopsis. (A) Phenotypes of root growth for transgenic and WT Arabidopsis on Day 9. (B) Heights of transgenic and WT Arabidopsis plants during first 12 d of growth. (C) POD content of WT and transgenic Arabidopsis plants after infection with V. dahliae. (D, E, F and G) Staining of lignin in WT (D), L1 (E), L2 (F) and L4 (G) transgenic Arabidopsis’s stem after inoculation with V. dahliae (15 days).
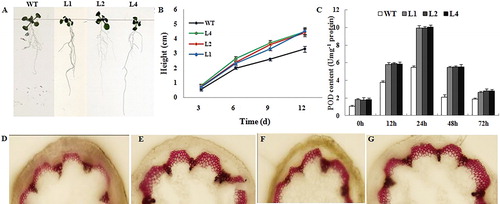
Lignin biosynthesis is one of the resistance reactions induced by pathogens. In particular, the expansion of pathogenic bacteria in plant fibro-vascular system was blocked by the synthesis of lignin, which was regulated by ET. In this study, we found that the lignin content of transgenic Arabidopsis was significantly higher than that of wild type after infection by V. dahliae, which may be related to the increase of ethylene synthesis. In addition, POD is an important oxidase in plants and one of the key enzymes in lignin synthesis. It also participates in the biosynthesis of ethylene. The POD activity of transgenic Arabidopsis increased significantly after infection by V. dahliae, which may have caused increasing ethylene biosynthesis and lignin synthesis. The more lignin may be the primary cause of the stronger roots and the resistance in transgenic Arabidopsis. This suggested that the increased disease resistance by Arabidopsis plants overexpressing GbWRKY1 was partially dependent upon the expression of genes related to ET synthesis.
Conclusions
In this study, the level of resistance to V. dahliae was distinctly different between GbWRKY1-expressing transgenic Arabidopsis plants and the WT. When this is combined with the results from our VIGS analysis, we can propose that SA, JA and ET are important factors in the development of disease resistance by cotton. Our data provide important information about the molecular processes of disease resistance in that crop and will facilitate further studies to breed cotton plants with improved resistance to Verticillium wilt.
Authors’ contributions
Shuling Zhang and Jianfeng Liu conceived and designed the research. Shuling Zhang prepared the materials, designed and performed the research and wrote the original manuscript. Lizhu Wu and Jun Yang participated in preparing the materials. Zhiying Ma revised thoroughly and finalized the manuscript.
Disclosure statement
The authors declare that they have no conflict of interest.
Additional information
Funding
References
- Park JM, Paek KH. Recognition and response in plant-pathogen interactions. J Plant Biol. 2007;50(2):132–138.
- Pape S, Thurow C, Gatz C. The Arabidopsis PR-1 promoter contains multiple integration sites for the coactivator NPR1 and the repressor SNI1. Plant Physiol. 2010;154(4):1805–1818.
- Sureshkumar V, Dutta B, Kumar V, et al. RiceMetaSysB: a database of blast and bacterial blight responsive genes in rice and its utilization in identifying key blast-resistant WRKY genes. Database (Oxford). 2019;2019:baz015 [13 p.]. doi:10.1093/database/baz015
- Ye M, Glauser G, Lou Y, et al. Molecular dissection of early defense signaling underlying volatile-mediated defense regulation and herbivore resistance in rice. Plant Cell. 2019;31(3):687–698.
- Ross CA, Liu Y, Shen QJ. The WRKY gene family in rice (Oryza sativa). J Integr Plant Biol. 2007;49(6):827–842.
- Rushton PJ, Somssich IE, Ringler P, et al. WRKY transcription factors. Trends Plant Sci. 2010;15(5):247–258.
- Shi W, Hao L, Li J, et al. The Gossypium hirsutum WRKY gene GhWRKY39-1 promotes pathogen infection defense responses and mediates salt stress tolerance in transgenic Nicotiana benthamiana. Plant Cell Rep. 2014;33(3):483–498.
- Wang XL, Yan Y, Li YZ, et al. GhWRKY40, a multiple stress-responsive cotton WRKY gene, plays an important role in the wounding response and enhances susceptibility to Ralstonia solanacearum infection in transgenic Nicotiana benthamiana. PLoS One. 2014;9:e93577 [12 p.]. doi:10.1371/journal.pone.0093577
- Yu FF, Huaxia YF, Lu WJ, et al. GhWRKY15, a member of the WRKY transcription factor family identified from cotton (Gossypium hirsutum L.), is involved in disease resistance and plant development. BMC Plant Biol. 2012;12(1):144–161.
- Yan Y, Jia H, Wang F, et al. Overexpression of GhWRKY27a reduces tolerance to drought stress and resistance to Rhizoctonia solani infection in transgenic Nicotiana benthamiana. Front Physiol. 2015;6:265–280.
- Liu X, Song Y, Xing F, et al. GhWRKY25, a group I WRKY gene from cotton, confers differential tolerance to abiotic and biotic stresses in transgenic Nicotiana benthamiana. Protoplasma. 2016;253(5):1265–1281.
- Zhang SL, Wang XF, Zhang Y, et al. GbWRKY1, a novel cotton (Gossypium barbadense) WRKY gene isolated from a full-length cDNA library, is induced by infection with Verticillium dahliae. Ind J Biochem Biophys. 2012;49:405–413.
- Liu Q, Li X, Yan S, et al. OsWRKY67 positively regulates blast and bacteria blight resistance by direct activation of PR genes in rice. BMC Plant Biol. 2018;18(1):257–269.
- Li J, Brader G, Palva ET. The WRKY70 transcription factor: a node of convergence for jasmonate-mediated and salicylate-mediated signals in plant defense. Plant Cell. 2004;16(2):319–331.
- Xu X, Chen C, Fan B, et al. Physical and functional interactions between pathogen-induced Arabidopsis WRKY18, WRKY40, and WRKY60 transcription factors. Plant Cell. 2006;18(5):1310–1326.
- Chen H, Lai Z, Shi J, et al. Roles of Arabidopsis WRKY18, WRKY40 and WRKY60 transcription factors in plant responses to abscisic acid and abiotic stress. BMC Plant Biol. 2010;10(1):281–295.
- Xu L, Zhu L, Tu L, et al. Differential gene expression in cotton defence response to Verticillium dahliae by SSH. J. Phytopathol. 2011;159(9):606–615.
- Doyle JJ, Doyle JL. A rapid DNA isolation procedure for small quantities of fresh leaf tissue. Phytochem Bull. 1987;19:11–15.
- Fradin EF, Zhang Z, Juarez Ayala JC, et al. Genetic dissection of Verticillium wilt resistance mediated by tomato Ve1. Plant Physiol. 2009;150(1):320–332.
- Gao X, Wheeler T, Li Z, et al. Silencing GhNDR1 and GhMKK2 compromises cotton resistance to Verticillium wilt. Plant J. 2011;66(2):293–305.
- Xu F, Yang L, Zhang J, et al. Prevalence of the defoliating pathotype of Verticillium dahliae on cotton in central China and virulence on selected cotton cultivars. J. Phytopathol. 2012;160(7-8):369–376.
- Gao WH, Sun LQ, Liu LL, et al. Proteomic and virus-induced gene silencing (VIGS) analyses reveal that gossypol, brassinosteroids and jasmonic acid contribute to the resistance of cotton to Verticillium dahliae. Mol Cell Proteomics. 2013;12(12):3690–3703.
- Livak KJ, Schmittgen TD. Analysis of relative gene expression data using real-time quantitative PCR and the 2(-Delta Delta C(T)) Method. Methods. 2001;25(4):402–408.
- Clough SJ, Bent AF. Floral dip: a simplified method for Agrobacterium-mediated transformation of Arabidopsis thaliana. Plant J. 1998;16(6):735–743.
- Sambrook J, Fritsch EF, Maniatis T. Molecular cloning: a laboratory manual. Cold Spring Harbor (NY): Cold Spring Harbor Laboratory Press; 1989.
- Li GJ, Meng XZ, Wang RG, et al. Dual-level regulation of ACC synthase activity by MPK3/MPK6 cascade and its downstream WRKY transcription factor during ethylene induction in Arabidopsis. PLOS Genet. 2012;8(6):e1002767. doi:10.1371/journal.pgen.1002767
- Wu KL, Guo ZJ, Wang HH, et al. The WRKY family of transcription factors in rice and Arabidopsis and their origins. DNA Res. 2005;12(1):9–26.
- Rajendra B, Jones JD. Role of plant hormones in plant defence responses. Plant Mol Biol. 2009;69:473–488.
- Caarls L, Van der Does D, Hickman R, et al. Assessing the role of ethylene response factor transcriptional repressors in salicylic acid-mediated suppression of jasmonic acid-responsive genes. Plant Cell Physiol. 2017;58(2):266–278.
- Caarls L, Pieterse CM, Van Wees SC. How salicylic acid takes transcriptional control over jasmonic acid signaling. Front Plant Sci. 2015;6:170–180.
- Pandey B, Grover A, Sharma P. Molecular dynamics simulations revealed structural differences among WRKY domain-DNA interaction in barley (Hordeum vulgare). BMC Genomics. 2018;19(1):114–132.
- Wang KLC, Li H, Ecker JR. Ethylene biosynthesis and signaling networks. Plant Cell. 2002;14(suppl 1):S131–S151.
- Van Loon LC, Geraats BPJ, Linthorst H. Ethylene as a modulator of disease resistance in plants. Trends Plant Sci. 2006;11(4):184–191.
- Jiang J, Ma S, Ye N, et al. WRKY transcription factors in plant responses to stresses. J Integr Plant Biol. 2017;59(2):86–101.
- Mao G, Meng X, Liu Y, et al. Phosphorylation of a WRKY transcription factor by two pathogen-responsive MAPKs drives phytoalexin biosynthesis in Arabidopsis. Plant Cell. 2011;23(4):1639–1653.