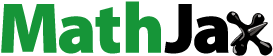
Abstract
Periwinkle (Catharanthus roseus L.) grown in the Mecca region contains more than 100 alkaloids such as vincristine and vinblastine used to treat leukaemia. The members of family Potyviridae, including Catharanthus mosaic virus (CatMV), cause natural infections in periwinkle. The presence of CatMV in collected samples was tested by double antibody sandwich enzyme linked immunosorbent assay and was confirmed by reverse transcription polymerase chain reaction using specific primers. The effect of CatMV infection on vincristine and vinblastine was determined by chromatographic techniques. All the isolated mixtures were evaluated by high-performance liquid chromatography. Vinblastine and vincristine were isolated by the use of a charcoal column. We evaluated the potential use of plant growth-promoting rhizobacterial isolates (Bacillus subtilis and Bacillus pumilus) against virus infection to stimulate resistance induction in host plants.
Introduction
Periwinkle (Catharanthus roseus), also known as Vinca rosea, belongs to Apocynaceae family, which contains active compounds such as alkaloids and tannins with anticancer activities [Citation1,Citation2]. The pharmacologically active compounds (vinacristine and vinblastine) are produced from stems and leaves and are necessary for cancer drugs, while roots have anti-hypertensive activity [Citation3–5].
Vinblastine and vinacristine are traditionally obtained from C. roseus and are an antimicrotubule drug used to treat more than one type of cancer [Citation5]. They are anti-neoplastic medicines and the monoindole alkaloids ajmalicine and serpentine are anti-hypertension drugs [Citation6–12]. Vinblastine sulphate is used commercially for the treatment of neoplasma and is recommended for generalized Hodgkin’s disease and resistant choiocarcenoma. The plant has been used in the treatment of diabetes, hypertension, tuberculosis, laryngitis, sore throat, dyspepsia, malaria and to regulate menstruation [Citation13–15]. Favretto et al. [Citation16] reported a semi-quantitative determination of vincrisitine and vinblastine by injection electrospray ionization mass spectrometric analysis. Scragg [Citation17] developed a new method for the isolation and purification of indole alkaloids from C. roseus by a centrifuged partition chromatography (CPC). Moreover, they determined both ajmalicine and serpentine by HPLC on a Bondapak C18 reverse phase column.
On the other hand, the family Potyviridae includes some viruses infecting periwinkle such as Catharanthus mosaic virus (CatMV) [Citation18,Citation19] and Lettuce mosaic virus (LMV) [Citation20] with a single stranded, positive sense RNA genome and flexuous, filamentous particles. Viral infection leads to a decrease in total photosynthetic pigments, total soluble intracellular protein and total phenol contents and to synthesis of alkaloids (vinblastine and vinacristine) and vindoline of C. roseus, compared to the healthy control seedlings. Due to the needs to protect plants from diseases, reduce crop losses and increase food quality, several plant protection approaches have been investigated such as biological control [Citation21], systemic acquired resistance inducers [Citation22] and plant growth-promoting rhizobacteria (PGPR) [Citation23,Citation24] to manage plant pathogens.
A plant virus disease management strategy depends mainly on the application of insecticides to control insect virus vectors. Two supplementary strategies such as cross-protection [Citation25–27] and PGPR [Citation28] have come within reach to manage viral diseases. The PGPR induce plant growth promotion either directly or indirectly and may exhibit biological activities against plant pathogens. There are still no available reports on the action of PGPR against vinca viruses. Exploring PGPR to manage plant viral diseases has started in the last few years with a few studies [Citation28–32]. In this study, we explored the roles of PGPR and their effect on naturally and artificially infected periwinkle plants in the search of approaches to protect healthy commercial periwinkle orchards throughout the Mecca region.
Materials and methods
Sampling
No special permission to access periwinkle locations was needed. The fields in the Mecca region, subject of this study, did not include endangered or protected species.
To obtain CatMV isolates, we collected samples of periwinkle plants showing mosaic leaf deformation and plant stunting, in September 2016. We collected 240 leaf samples from naturally infected nursery gardens, orchards and landscape plantings of Pacifica, Titan and Sun Storm periwinkle plants. All the samples were collected from eight periwinkle-growing provinces of the West Mecca region, including Taif, Makkah, Jeddah, Bahra, Khulais, Aljummum, Lith and Rabigh provinces.
Diagnosis of the virus isolate by serological assay
Double antibody sandwich enzyme linked immunosorbent assay (DAS-ELISA) was done according to the manufacturer’s instructions using the CatMV specific antiserum (Bioreba AG Christoph Merian-Ring 7, CH-4153 Reinach BL1 Switzerland) as previously described [Citation18,Citation19]. All samples were examined three times for confirmation. The plant samples which gave a positive reaction were used as a source of CatMV infection.
Periwinkle seedlings as systemic hosts were inoculated with infected juice containing CatMV virus. The inoculated periwinkle seedlings were kept in separate cages and were observed for symptom expression 3–4 weeks after inoculation, as a sign of virus infection.
Molecular detection of CatMV by RT-PCR
Total nucleic acid extraction (TNA)
Total nucleic acids (TNAs) were extracted from 100 mg of leaf veins or cortical scrapings ground in 1 mL grinding buffer (4.0 mol/L guanidine thiocyanate, 0.2 mol/L NaOAc pH 5.2, 25 mmol/L ethylenediaminetetraacetic acid (EDTA), 1.0 mol/L KOAc and 2.5% (w/v) PVP-40) and silica-purified according to Foissac et al. [Citation33].
cDNA synthesis
Eight to 10 µL of TNA extracts were mixed with 1 µL of random hexamer primers (Boehringer Mannheim, GbmH) (0.5 µg/µL), denatured at 95 °C for 5 min and quickly chilled on ice. Reverse-transcription was done for 1 h at 39 °C by adding 4 µL M-MLV buffer 5× (50 mmol/L Tris–HCl pH 8.3, 75 mmol/L KCl, 3 mmol/L MgCl2), 2 µL of 10 mmol/L DTT, 0.5 µL of 10 mmol/L deoxyribonucleoside triphosphates (dNTPs) and 200 units of Moloney Murine Leukaemia virus (M-MLV) reverse transcriptase (Bethesda Research Laboratories, USA) in a final volume of 20 µL.
PCR
Detection of CatMV by RT-PCR was conducted using specific primers () as previously described by Conceicao et al. [Citation18]. Briefly, 2.5 µL of reverse-transcribed TNA mixture was amplified with the addition of 2.5 µL of 10 × Taq polymerase buffer (Promega Corporation, USA), with a final concentration of 1.5 mmol/L MgCl2 in a 25 µL total volume. PCR products were analyzed by electrophoresis on 1.2% agarose gel prepared in 1× tris-borate-EDTA (TBE) buffer [Citation34], stained with ethidium bromide and examined on a UV transilluminator.
Table 1. Nucleotide sequences of oligonucleotide primers used for RT-PCR to detect CatMV.
Molecular detection of CatMV by PCR
To investigate the potential viral infection, total DNA was extracted from leaves of 32 infected plants using the cetyl trimethyl ammonium bromide (CTAB) method [Citation34]. To confirm the presence of virus in infected samples, PCR was performed using a pair of degenerate primers specific to the coat protein region of begomovirus.
A typical PCR contained about 100 ng DNA template, Taq 10× buffers (10 mmol/L Tris–HCl, pH 8.8; 50 mmol/L KCl) 25 mmol/L MgCl2, 200 μmol/L of each dNTP, 2 units of Taq DNA polymerase, nuclease-free water and 10 pmol/L of each primer. The PCR thermal profile was pre-PCR denaturation at 94 °C for 120 s followed by 35 cycles of denaturing at 94 °C for 45 s, annealing at 55 °C for 45 s and extension at 72 °C for 45 s, and a final extension at 72 °C for 5 min [Citation35].
PGPR strains and inoculums preparation
The selected PGPR strain B1 (Bacillus subtilis) was isolated from the rhizosphere soil of a healthy tomato plant and strain B2 (Bacillus pumilus) was isolated from the rhizosphere soil of a healthy cucumber plant [Citation36]. The PGPR isolates were identified at the Biotechnology Lab, Agricultural Research Institute, Giza, Egypt. All bacterial isolates were grown in nutrient agar medium. The bacterial cells were transferred to nutrient-broth (NB) medium and incubated for 24 h at 37 °C, yielded and re-suspended in 10 mL sterile distilled water. The concentration of bacteria was attuned to 108 colony-forming units (CFU)/mL by measuring the absorbance at 660 nm. PGPR strains were stored in tryptic soy broth (TSB) adjusted with 15% glycerol at −80 °C until use.
Effectiveness of some PGPR isolates against periwinkle virus
Periwinkle seeds of pacifica Polka Dot cultivar were germinated and developed in plastic pots (30 × 30 cm). Soil was mixed with PGPR suspension (1 × 108 CFU/cm3) at a ratio of 1:50 (v/v) immediately after seed planting (with a handheld sprayer until runoff) and once drenched with 20 mL of the PGPR strain suspensions again when plants reached the true-leaf stage (approximately 14 days from sowing seed).
Periwinkle seedlings were inoculated with CatMV 7 days after initial PGPR drenching soil. The CatMV inoculums were prepared from CatMV infected top periwinkle leaves, which gave a positive ELISA reaction. The periwinkle seedlings were conserved in a greenhouse at 24–27 °C. ELISA test was used in addition to symptom observation to checking for the presence of the virus.
Seedling pots were placed in a completely randomized block design with three seedlings per pot, three pots per replicate and three replicates per treatment, giving a total of 27 seedlings per treatment. Treatments were performed: (T1) control healthy, (T2) healthy with B1, (T3) healthy with B2, (T4) healthy with B1 + B2 (at a rate of 1:1), (T5) control infected by CatMV, (T6) CatMV with B1, (T7) CatMV with B2, (T8) CatMV with (B1 + B2). After 5 weeks from CatMV inoculation, plants of a similar size were selected and examined for growth and physiological parameters.
Evaluation of changes in virus infection treated with viruses and PGPR isolates treatment
One month after inoculation, the percentage of infection and disease severity were determined according to the following scale: 0 = no symptoms, 1 = light mottling and crinkling, 2 = mild mosaic and crinkling, 3 = severe mosaic, crinkling and size reduction; and 4 = malformation. Disease severity (DS) values were calculated using the following formula according to Yang et al. [Citation37]
The youngest developed leaves from both control and treated seedlings of periwinkle cultivars were collected after one month from inoculation for analysis of changes.
Total phenol content
Total phenolic concentration was measured by the Folin–Ciocalteu colourimetric method with methanolic plant extracts and absorbance at 765 nm [Citation38]. The standard curve was prepared using different dilutions of gallic acid in methanol:water (50:50, v/v). The results were expressed in terms of GAE (gallic acid equivalent) mg/g.
Total flavonoid content
Flavonoid concentration was determined in methanolic plant extracts by an aluminium chloride colourimetric method, in which the absorbance was measured at 415 nm [Citation39]. Total flavonoid content was determined based on a standard curve of quercetin at different concentrations in methanol (g/mL).
Alkaloids
Plant extracts (1 mg) were dissolved in dimethyl sulphoxide (DMSO). A set of reference standard solutions of atropine (20, 40, 60, 80 and 100 μg/mL) were prepared. The absorbance of the test and standard solutions were determined against the reagent blank at 470 nm with a UV/Visible spectrophotometer. The total alkaloid content was expressed as aconitine equivalent per gram of extract (AE mg/g) [Citation40].
Evaluation of vinblastine and vincristine in CatMV infected plants treated with PGPR isolates
Preparation of total alkaloids from C. roseus (adsorption on charcoal column)
In preliminary experiments, the following method was chosen as most reliable to obtain total alkaloids. Briefly, 1 kg of C. roseus powdered plant was percolated overnight with methanol (95%, 3 L ×3). The total methanolic extract was filtered and concentrated at 50 °C in vacuum to 500 mL, diluted with water (500 mL), then acidified with 1 N sulphuric acid (pH 2). The combined aqueous acidic extract was kept overnight in a refrigerator, and then filtered from resinous bodies. The clear filtrate was allowed to pass through a glass column packed with 500 (g) charcoal (4 cm × 100 cm) previously activated at 120 °C in an oven for 2 h. Eluates were taken at different intervals and tested for the presence of alkaloids using Mayer’s reagent to ensure that the alkaloidal mixture was completely adsorbed. The column was first washed with distilled water (2 L), then eluted in a gradient manner starting with 30% methanol, followed by 50%, 70% and finally 100% methanol, respectively using 500 mL of each solvent.
Each of the eluted fractions was concentrated separately in vacuum at 50 °C till solvent-free. The aqueous solution was adjusted to pH 6.4 using NH4OH solution (25%) and then extracted with methylene chloride (500 mL ×5). The combined methylene chloride extract was washed with distilled water till alkali-free, dried over anhydrous sodium sulphate and then evaporated in vacuum to dryness to give an alkaloidal fraction eluted by 70% methanol (I) which was found to be very rich in vincristine and vinblastine (fraction I, 1.033 g). The obtained fractions were separately tested to confirm that they contain total alkaloid with high purity [Citation40]. The fractions obtained from the method for preparing total alkaloids were subjected to high performance liquid chromatography (HPLC) analysis using isocratic elution with phosphate buffer solution of pH 6.5, water:acetonitrile (55:45) as the mobile phase.
Statistical analysis
All data were analysed by analysis of variance (ANOVA). All statistical tests were carried out using SPSS software version 26 (IBM SPSS Statistics for Windows, Version 25.0. Armonk, NY: IBM Corp.).
Results and discussion
Detection of CatMV source and propagation
Periwinkle leaves showing evidence of infection with typical symptoms of CatMV, that is, samples of periwinkle plants showing mosaic, leaf deformation and plant stunting, were collected from several nurseries, orchards and landscape plantings of periwinkle plants growing in the Mecca region (). Samples which yielded a positive reaction for CatMV were used as a source of virus infection, and samples which yielded a negative reaction were discarded. The resulting virus isolate was propagated as a pure CatMV source in a proliferation host such as periwinkle (pacifica Polka Dot cultivar). The symptoms of mosaic, leaf deformation and plant stunting that developed were observed on periwinkle leaves. This observation was in agreement with previous studies [Citation18,Citation19,Citation41].
Figure 1. Typical symptoms of natural infection of periwinkle with CatMV (A): mosaic leaf deformation and plant stunting; compared to healthy periwinkle leaves (B).
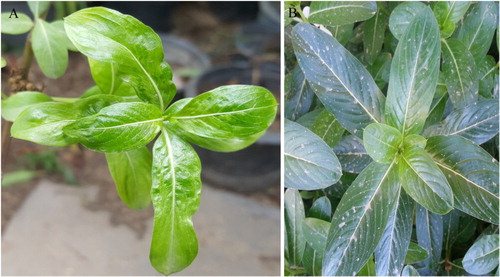
Conceicao et al. [Citation18] and Svanella-Dumas et al. [Citation20] reported that CatMV and LMV were found in C. roseus causing mosaic symptoms, foliar malformation and flower variegation. Elbeshehy et al. [Citation19] performed a study on periwinkle widely grown as an ornamental plant in the Mecca region in the KSA. Different symptoms similar to those induced by viruses (mosaic, vein clearing, mottling, yellowing, flower variegation) prompted surveys in eight different regions of KSA to assess the presence of periwinkle-infecting viruses, that is, CatMV and Catharanthus yellow mosaic virus (CYMV) and the results showed that viruses were present in the surveyed areas, with incidence of infection reaching 15% for CatMV [Citation19]. Mollov et al. [Citation41] reported that the plants showed mosaic symptoms, leaf deformation, premature leaf senescence and vine dieback. Filamentous virus particles with modal lengths of 700–900 nm were observed by transmission electron microscopy (TEM) in partially purified preparations from symptomatic leaves [Citation41].
RT-PCR
We detected the CP gene encoding the CatMV coat protein in infected periwinkle seedlings by RT-PCR. The PCR fragment of the expected size of 650 bp was amplified (). No bands were obtained from the samples from healthy plants, similar to previous reports [Citation18,Citation19,Citation41].
PGPR application and CatMV symptoms
The plants under study were observed for symptom propagation and severity one month after inoculation. All the results of the PGPR treatments showed effective repression of the viral symptoms caused by CatMV compared to the infected controls over two repeated experiments. Moreover, the periwinkle leaves treated with the mixture of B1 + B2 showed fewer symptoms than the leaves treated with the B1 strain or the B2 strain individually (data not shown). Induced systemic resistance (ISR) has been documented as a promising means for managing plant diseases due to the efficiency of induced resistance against various pathogens occurring under authentic crop field conditions. PGPR are among the different groups of plant-associated bacteria that can stimulate natural plant defences against bacterial and fungal plant pathogens [Citation42–44]. However, only a small number of studies have explored their activity against viral plant pathogens [Citation45,Citation46], excluding CatMV.
Virus concentration and DS
The development of CatMV infection in periwinkle plants was confirmed by ELISA and was associated with increased infection rate and disease severity. The plants grown in soil drenched with PGPR showed a significant reduction in CatMV-related parameters compared to the infected control plants in all replicated greenhouse assays (). That is, the infected control showed average disease severity that was higher than any of the other treatments. Treatment with the mixture of B1 + B2 or B2 alone resulted in disease suppression of 81.48% and 88.89%, respectively, which was significantly higher than that in plants treated with B1 alone (59.26%). also shows the ELISA values as a measurement of the CatMV concentration in young leaves of periwinkle seedlings at 21 days after inoculation. The indicated ELISA values for the leaf samples collected from each PGPR treatment were significantly lower than these of the infected plants. The addition of B2 and B1 + B2 in the soil yielded significantly lower ELISA values of CatMV compared with the B1 treatment.
Table 2. Effects of PGPR strains B1, B2 and B1 + B2 on ELISA values, disease severity percentage, and percentage of infections caused by CatMV in periwinkle seedlings under greenhouse conditions.
Decreasing of virus symptoms by PGPR application has been formerly reported [Citation23]. According to Akbari et al. [Citation47], PGPR can potentially induce ISR against CatMV to reduce symptoms or reduce viral accumulation in infected periwinkle plants. Based on the obtained data, the two PGPR isolates were able to reduce disease symptoms and severity caused by CatMV virus infection, which indicates the presence of ISR. Inoculation with a mixture of the B1 + B2 strains or individually provided satisfactory disease suppression.
Total phenol, flavonoid and alkaloid contents
The total phenol and flavonoid contents reached 495.13, 470.84 and 441.22 mg/g fresh weight () and 47.81, 45.22 and 41.34 mg/g fresh weight (), respectively, in healthy seedlings grown in soil with B2, B1 + B2 and B1, respectively, compared with healthy controls (300.19 mg/g and 37.87 mg/g fresh weight). In contrast, the infected seedlings grown with different PGPR (B2, B1 + B2 and B1) reached total phenol content of 463.22, 431.72 and 299.54 mg/g fresh weight and flavonoid content of 44.60, 39.85 and 34.79 mg/g fresh weight, respectively, which were higher than those in the infected control seedlings (). The cultivars grown in soil with the PGPR strain B2 showed a highly significant increase in phenolics and flavonoids in healthy and infected periwinkle seedlings, which accumulated phenolics and flavonoids less than the seedlings infected by virus and more than the control seedlings ().
Figure 3. Effects of PGPR strain treatments on total phenol content (a), alkaloid content (b) and flavonoid content (c) of periwinkle seedlings infected with CatMV under greenhouse conditions.
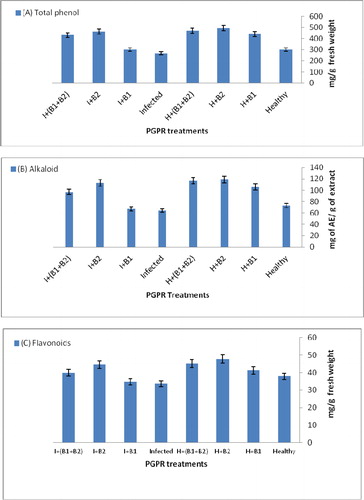
The data in show that the application of PGPR led to an increase in the alkaloid content of periwinkle leaf cultivars (pacifica Polka Dot) compared to the infected control leaves (73.16 and 64.13 mg of AE/g of extract, respectively). The application of PGPR (B2, B1 + B2 and B1) increased the alkaloid content in healthy and infected seedlings treated with PGPR compared to healthy and infected control seedlings (118.41, 116.85 and 105.75 mg AE/g in healthy treated seedlings compared to 112.93, 96.73 and 67.16 mg AE/g of extract, respectively).
On the other hand, Moreno et al. [Citation14] reported that more than 100 alkaloids found in periwinkle, of which vincristine and vinblastine are the most notable for the treatment of diseases such as leukaemia. That is why we studied the effect of CatMV infection on the antineoplastic alkaloids in periwinkle leaves and the effect of using PGPR isolates against virus infection to stimulate resistance induction in host plants. Our findings demonstrate the possibility of using selected Bacillus spp. strains to induce systemic resistance for control of CatMV infection.
The phenolics, flavonoids and alkaloids are important secondary metabolites present in plants and can be antioxidant agents. Plant secondary metabolites play an important role in defence mechanisms against abiotic stress and against phytopathogens based on their toxicity and repellence to microbes [Citation48–51]. PGPRs can induce the synthesis of secondary metabolites in plants, including terpenoids, phenolics, alkaloids and essential oils mostly consisting of monoterpenes, sesquiterpenes and phenylpropanoids [Citation52,Citation53].
Vinblastine and vincristine in CatMV infected plants treated with PGPR isolates
Vinblastine and vincristine were isolated, in a pure form, from C. roseus cultivated in Mecca regions, by several chromatographic protocols. Modified methods for the preparation of total alkaloids were used [Citation40]. The isolated mixture was evaluated by HPLC analysis. The antineoplastic alkaloids, vinblastine and vincristine, were isolated by the use of a charcoal column.
HPLC was used for the preparation of the antineoplastic alkaloids from C. roseus powdered plant. The highest percentage of total alkaloids was obtained by this method and the purest mixture was obtained. The maximum quantitative yield of vinblastine- and vincristine-rich fraction obtained using qualitative and quantitative HPLC analysis is shown in . HPLC studies of different fractions obtained from the method used in the preparation of total alkaloids showed that the two target alkaloids (viz. vinblastine and vincristine) were found together in a fraction as its main constituents ().
Table 3. Quantitative determination of alkaloidal fractions (vinblastine and vincristine-rich fraction) of C. roseus inoculated by CatMV and treated with PGPR strains (B1, B2 and B1 + B2).
When the fractions obtained from the method for preparing total alkaloids were subjected to HPLC analyses using isocratic elution with phosphate buffer solution of pH 6.5, water:acetonitrile (55:45) as the mobile phase, there was a difference in the retention times of the target alkaloids, viz. vinblastine and vincristine, of 3.771 min. The HPLC analysis of the total alkaloids prepared by this method indicated that the fraction eluted from the charcoal column by 70% methanol (K) gave a relatively pure fraction containing the target alkaloids vinblastine and vincristine with only six other alkaloids [Citation6,Citation40].
Conclusions
In this study, two PGPR strains (B1 and B2) were examined for their potential activity against virus infection of periwinkle seedlings. Individual treatment by B2 or in combination with B1 showed inhibiting activity against virus symptoms, decreased virus concentration and decreased disease severity. Evaluation of the total phenol, total flavonoid and alkaloid contents revealed that PGPR application had a positive effect on plants challenged with CatMV. Using a charcoal column is a very simple, easy, cheap and reliable technique for isolation of a highly purified form of the total alkaloids especially vinblastine and vincristine and proved to be very reproducible particularly for the production scale. The results obtained from HPLC analysis were promising. The obtained results suggest the potential of the two PGPR isolates to protect periwinkle seedlings against CatMV, through stimulating plants to produce secondary metabolites and activating ISR against virus infection.
Acknowledgements
The authors thank Dr. Othman A. Alghamdi, Head of Biological Sciences Department, Faculty of Science, University of Jeddah, Jeddah, Saudi Arabia, for providing scientific and moral support for this research.
Disclosure statement
No potential conflict of interest was reported by the authors.
Correction Statement
This article has been republished with minor changes. These changes do not impact the academic content of the article.
Additional information
Funding
References
- Jordan MA, Thrower D, Wilson L. Mechanism of inhibition of cell proliferation by Vinca alkaloids. Cancer Res. 1991;51(8):2212–2222.
- Jaleel CA, Gopi R, Lakshmanan GMA, et al. Triadimefon induced changes in the antioxidant metabolism and ajmalicine production in Catharanthus roseus (L.) G. Don. Plant Sci. 2006;171(2):271–276.
- Cowley RC, Bennett FC. Vinca rosea. Aust J Pharm. 1928;9:61.
- Pillay PP, Nair CPM, Santi Kumari TN. Lochnera rosea as a potential source of hypotensive and other remedies. Bull Res Inst Univ Kerala. 1959;1:51–54.
- Kumar A, Patil D, Rajamohanan PR, et al. Isolation, purification and characterization of vinblastine and vincristine from endophytic fungus Fusarium oxysporum isolated from Catharanthus roseus. PLoS ONE. 2013;8(9):e71805.
- Zhao J, Verpoorte R. Manipulating indole alkaloid production by Catharanthus roseus cell cultures in bioreactors: from biochemical processing to metabolic engineering. Phytochem Rev. 2007;6(2–3):435–457.
- Bashir M, Hafeez M, Perveen N, et al. A rapid procedure for the isolation of Catharanthine, vindoline and vinblastine . Planta Med. 1983;47(4):246–247.
- Atta-Ur-Rahman Fatima J. Isolation and structure of rosicine from C. roseus. Tetrahedron Lett. 1984;25(52):6051–6054.
- Atta-Ur-Rahman IA, Bashir M. Isolation of rhazinal from the leaves of C. roseus. J Nat Products. 1984;47 2) :389.
- Atta-Ur-Rahman IA. Chaudry MI. Isolation and 13C-NMR studies on cathovaline on alkaloid from leaves of C. roseus. Planta Med. 1985;5:447–448.
- Atta-ur-Rahman, Alam M, Ali I, Habib-ur-Rahman, Haq I. Leurosinone: a new binary indol alkaloid from C. roseus. J Chem Soc Perkin Trans I. 1988;8:2175–2178.
- Auriola S, Naaranlahti T, Kostiainen R, et al. Identification of indol alkaloids of C. roseus with liquid chromatography/mass spectrometry using collision-induced dissociation with the thermospray ion repeller. Biol Mass Spectrom. 1990;19(7):400–404.
- Kanika B, Shweta S, Dharmendra KP. Phytochemical and pharmaceutical panorama of Catharanthus roseus. Indo Am J Pharm Sci. 2016;3(3):288–293.
- Moreno PRH, van der Heijden R, Verpoorte R. Effect of terpenoid precursor feeding and elicitation on formation of indole alkaloids in cell suspension cultures of C. roseus. Plant Cell Rep. 1993;12(12):702–705.
- Lee S-Y, Choi P-S, Chung H-J, et al. Comparison of adventitious shoot formation in petiole explant cultures of 20 cultivars of C. roseus. J Plant Biotechnol. 2003;5(1):59–61.
- Favretto D, Piovan A, Filippini R, et al. Monitoring the production yields of vincristine and vinblastine in C. roseus from somatic embryogenesis. Semiquantitative determination by flow-injection electrospray ionization mass spectrometry. Rapid Commun Mass Spectrom. 2001;15(5):364–369.
- Scragg AH. Alkaloids accumulation in C. roseus suspension cultures. Methods Mol Bio. 1999;111:393–402.
- Conceicao MS, Da Silva RF, Reis MS, et al. Characterization of a new potyvirus causing mosaic and flower variegation in Catharanthus roseus in Brazil. Sci Agric. 2011;68(6):687–690.
- Elbeshehy EKF, Hassan SM, Al-Zahrani AY, et al. Elbeaino viruses infecting periwinkle (Catharanthus roseus L.) in Western Saudi Arabia. Phytopathol Mediterr. 2017;56(3):479–485.
- Svanella-Dumas L, Verdin E, Faure C, et al. Adaptation of Lettuce mosaic virus to Catharanthus roseus involves mutations in the central domain of the VPg. MPMI. 2014;27(5):491–497.
- Conway KE, Maness NE, Motes JE. Integration of biological and chemical controls for Rhizoctonia aerial blight and root rot of rosemary. Plant Dis. 1997;81(7):795–798.
- Kessman H, Staub T, Hofmann C, et al. Induction of systemic acquired disease resistance in plants by chemicals. Annu Rev Phytopathol. 1994;32:439–459.
- Kloepper JW, Leong J, Teintze M, et al. Enhanced plant growth by siderophores produced by plant growth-promoting rhizobacteria. Nature. 1980;286(5776):885–886.
- Kloepper JW, Schroth MN, Miller TD. Effects of rhizosphere colonization by plant growth promoting rhizobacteria on potato plant development and yield. Phytopathology. 1980;70(11):1078–1082.
- Fitchen JH, Beachy RN. Genetically engineered protection against viruses in transgenic plants. Annu Rev Microbiol. 1993;47(1):739–763.
- Lomonossoff GP. Pathogen-derived resistance to plant viruses. Annu Rev Phytopathol. 1995;33(1):323–343.
- Antoun H, Kloepper JW. Plant growth promoting rhizobacteria (PGPR) In: Brenner S, Miller JM, editors. Encyclopedia of genetics. New York (NY): Academic Press; 2001. p 1477–1480.
- Jetiyanon K, Fowler WD, Kloepper JW. Broad-spectrum protection against several pathogens by PGPR mixtures under field conditions in Thailand. Plant Dis. 2003;87(11):1390–1394.
- Al-Zahrani HSM, Elbeshehy EKF, Aldhebiani AY. Effect of Cucumber mosaic virus (CMV) infection on antineoplastic alkaloids from periwinkle (Catharanthus roseus L.) cultured in the Mecca region and resistance induction by plant-growth promoting rhizobacteria (PGPR). Biotechnol Biotechnol Equip. 2017;32(1):49–75.
- Harish S, Kavino M, Kumar N, et al. Induction of defense-related proteins by mixtures of plant growth promoting endophytic bacteria against Banana bunchy top virus. Biol Control. 2009;51(1):16–25.
- Wang S, Wu H, Zhan J, et al. The role of synergistic action and molecular mechanism in the effect of genetically engineered strain Bacillus subtilis OKBHF in enhancing tomato growth and Cucumber mosaic virus resistance. Bio Control. 2011;56:113–121.
- Elbeshehy EKF, Youssef SA, Elazzazy AM. Resistance induction in pumpkin Cucurbita maxima L. against watermelon mosaic potyvirus by plant growth-promoting rhizobacteria. Biocontrol Sci Technol. 2015;25(5):525–542.
- Foissac X, Svanella-Dumas L, Gentit P, et al. Polyvalent detection of fruit tree Tricho, Capillo and Foveavirus by nested RT-PCR using degenerated and inosine containing primers (DOP RT-PCR). Acta Hortic. 2001;550:37–43.
- Manen J-F, Sinitsyna O, Aeschbach L, et al. A fully automatable enzymatic method for DNA extraction from plant tissues. BMC Plant Biol. 2005;5(1):23.
- Marwal A, Sahu A, Sharma P, et al. Molecular characterizations of two begomoviruses infecting Vinca rosea and Raphanus sativus in India. Virol Sin. 2013;28(1):53–56.
- Youssef SA, Shaban WI. Management of tomato fusarium wilt by plant growth promoting rhizobacteria. 4th Conference of Sustainable Agricultural Development Fayoum University. Fayoum City, Egypt. 2008 October 20.
- Yang X, Liangyi K, Tien P. Resistance of tomato infected with Cucumber mosaic virus satellite RNA to potato spindle tuber viroid. Ann Appl Biol. 1996;129(3):543–551.
- McDonald S, Prenzler PD, Antolovich M, et al. Phenolic content and antioxidant activity of olive extracts. Food Chem. 2001;73(1):73–84.
- Chang CC, Yang MH, Wen HM, et al. Estimation of total flavonoid content in propolis by two complementary colorimetric methods. J Food Drug Anal. 2001;10:178–182.
- Shams KA, Nazif NM, Abdel Azim NS, et al. Isolation and characterization of antineoplastic alkaloids from Catharanthus roseus L. Don. cultivated in Egypt. Afr J Tradit Complement Altern Med. 2009;6(2):118–122.
- Mollov D, Guaragna MA, Lockhart B, et al. First report of Catharanthus mosaic virus in Mandevilla in the United States. Plant Disease. 2015;99(1):165.
- Van Loon LC, Glick GR. Increased plant fitness by rhizobacteria. In: Sandermann H, editor. Molecular ecotoxicology of plants. Vol. 170. Berlin: Springer-Verlag; 2004. p. 177–205.
- Liang J, Rong-Xiang TR, Hao Z, et al. Induction of resistance in cucumber against seedling damping-off by plant growth-promoting rhizobacteria (PGPR) Bacillus megaterium strain L8. Afr J Biotechnol. 2011;10:6920–6927.
- Tamm L, Thürig B, Fliessbach A, et al. Elicitors and soil management to induce resistance against fungal plant diseases. NJAS – Wagen J Life Sci. 2011;58(3–4):131–137.
- Dashti NH, Ali NY, Cherian VM, et al. Application of plant growth-promoting rhizobacteria (PGPR) in combination with a mild strain of Cucumber mosaic virus (CMV) associated with viral satellite RNAs to enhance growth and protection against a virulent strain of CMV in tomato. Canad J Plant Pathol. 2012;34(2):177–186.
- Kavino M, Harish S, Kumar N, et al. Induction of systemic resistance in banana (Musa spp.) against Banana bunchy top virus (BBTV) by combining chitin with root-colonizing Pseudomonas fluorescens strain CHA0. Eur J Plant Pathol. 2008;120(4):353–362.
- Akbari PA, Ghalavand AM, ModaresSanavy M, et al. Comparison of different nutritional levels and the effect of plant growth promoting rhizobacteria (PGPR) on the grain yield and quality of sunflower. Aus J Crop Sci. 2011;5(12):1570–1576.
- Razali N, Razab R, Junit SM, et al. Radical scavenging and reducing properties of extracts of cashew shoots (Anacardium occidentale). Food Chem. 2008;111(1):38–44.
- Prasad R, Kamal S, Sharma PK, et al. Root endophyte Piriformospora indica DSM 11827 alters plant morphology, enhances biomass and antioxidant activity of medicinal plant Bacopa monniera. J Basic Microbiol. 2013;53(12):1016–1024.
- Singh N, Yadav A, Varma A. Effect of plant growth promoting activity of rhizobacteria on Cluster bean (Cyamopsis tetragonoloba L.) plant growth and biochemical constituents. Int J Curr Microbiol Appl Sci. 2015;4(5):1071–1082.
- Priyanka T, Sujata MA. Study on potential phytopharmaceuticals assets in Catharanthus roseus L. (Alba). Int J Life Sci Biotechnol Pharma Res. 2016;5(1):1–6.
- Ceccarelli N, Curadi M, Martelloni L, et al.. Mycorrhizal colonization impacts on phenolic content and antioxidant properties of artichoke leaves and flower heads two years after field transplant. Plant Soil. 2010;335(1–2):311–323.
- Anitha Sri S. Pharmacological activity of vinca alkaloids. Res Rev: J Pharmacog Phytochem. 2016;4(3):27–34.