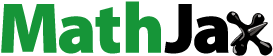
Abstract
Cucumber (Cucumis sativus L.) damping-off disease caused by Phytophthora melonis is a limiting factor affecting cucurbit production. This study assessed a collection of 38 domestic and exotic commercial cucumber genotypes for damping-off resistance at transplanting and 50% flowering. Plants were classified using a four-score scale, the average damping-off percentage ranging from 7.92% (resistant) to 88.01% [highly susceptible (HS)]. Most of the genotypes were susceptible and HS to P. melonis. These genotypes were analyzed with 15 inter-simple sequence repeat (ISSR) markers. A total of 317 bands were produced, of which 297 bands (93.69%) were polymorphic. The primer ISSR29 possessed the highest resolving power, polymorphic information content and marker index value, which could be the most informative primer for distinguishing cucumber genotypes. Cluster analysis and principal component analysis were carried out and there was a probable connection between cucumber genotypes and resistance level against P. melonis, indicating possible application for ISSR in studying disease resistance of cucumber and producing cultivars that resist damping-off. The expression of three candidate genes probably involved in the defence against P. melonis was quantified by quantitative real-time polymerase chain reaction in five cucumber genotypes showing different susceptibility to the pathogen. CsWRKY20, CsLecRK6.1 and LOX1 genes were differentially expressed depending on genotype in early stages of infection. LOX1 was the most expressed gene across experiments and the one that best discriminated between susceptible and resistant genotypes. These results are a valuable resource for future functional genomics studies to unravel the molecular mechanisms of C. sativus/P. melonis interaction.
Introduction
Cucumber (Cucumis sativus L.; 2n = 2x = 14) breeding for improving yield potential, quality, disease and pest resistance is underway [Citation1]. Cucumber is one of the most important vegetable crops worldwide and ranks the fourth in global vegetable production, showing a narrow genetic base despite its considerable morphological variability [Citation2,Citation3].
The hemibiotrophic oomycete Phytophthora melonis is one of the most destructive pathogens of cucumber causing damping-off and severe yield losses [Citation4,Citation5]. The disease is characterized by root and root collar rot, stem lesions, foliar blight and fruit rot in greenhouses [Citation6–8]. Although, the use of disease-resistant genotypes is a key to environmentally friendly and economically sustainable disease control in modern crop production, the employment of genetic resistance to minimize yield losses induced by P. melons remains largely unexplored in cucumber. To date, no resistant cucumber cultivar has been developed so far and few reports are available with regard to Phytophthora damping-off [Citation9–11]. Therefore, identifying resistant genotypes and improving the genetic resistance of cucumber to P. melonis is an effective approach toward development of damping-off resistance in cucumber.
To identify and improve cucumber cultivars with desirable characteristics, breeders need suitable knowledge of cucumber germplasm genetic diversity. Crop genetic diversity is a fundamentally important resource for improving yield stability. Many methods have been employed to assess genetic diversity in cucumber, ranging from morphological traits to molecular markers. Molecular markers are a valuable tool for genotype characterization and estimation of genetic diversity. To date, genetic diversity in cucumber has been evaluated with polymerase chain reaction (PCR)-based DNA marker systems, such as random amplified polymorphic DNA (RAPD) [Citation12], simple sequence repeats (SSRs) [Citation13,Citation14], expressed sequence tag-SSRs (EST-SSRs) [Citation15] and inter simple sequence repeat (ISSR) markers [Citation16,Citation17]. Among these markers, ISSR is an efficient and reproducible marker to assess genetic diversity because it is a simple and quick method, has low cost, high polymorphism and more importantly, does not require prior knowledge of DNA sequences [Citation18].
Plants have developed diverse constitutive defence mechanisms against pathogens, and understanding of the interactions between plants and pathogens has been a major area of investigation for many years. Lipoxygenases (LOXs) are strongly induced when plants respond to infection by fungal, bacterial or viral pathogens [Citation19]. Recently some WRKY transcription factors and lectin receptor kinase were found to be involved in protection mechanisms [Citation20,Citation21]. In several plant species, strong and rapid induction of WRKY genes has been reported upon pathogen infection. In cucumber, CsWRKY20 may be involved in disease resistance against P. melonis [Citation22]. The CsLecRK6.1 gene among all 25 identified CsLecRKs genes in cucumber was especially induced by P. capsici and P. melonis in JSH (resistant cultivar) [Citation5]. Despite these results, the regulatory roles of most of the identified WRKY TFs and LecRKs genes in defence response are still largely unknown.
The objectives of the study were screened to identify damping-off resistant lines/hybrids in cucumber genotypes to P. melonis under greenhouse conditions and estimate genetic variation of resistant and susceptible cucumber genotypes using ISSR markers. In addition, attention was focused on the expression of three genes, LOX1, CsWRKY and CsLecRK6.1, in response to P. melonis infection in five hybrid genotypes with different responses to P. melonis, in order to understand some of the adaptation and defence mechanisms which provide cucumber resistance to damping-off in the early stages of infection.
Materials and methods
Plant material and screening to P. melonis
Thirty-eight cucumber genotypes, including domestic and exotic hybrids, and inbreeding lines from different seed companies (), were examined for genetic diversity and resistance to damping-off disease caused by P. melonis at two growth stages: seedling (45-day-old seedlings) and maturing (50% flowering) stages. The study was carried out in a greenhouse and the laboratory of the Agricultural and Natural Resource Research and Education Centre, Isfahan, Iran, in 2018. These genotypes had not been previously screened for resistance to P. melonis. The genotypes ‘Sina’ and ‘Bosco’, being resistant and HS to P. melonis (P. drechsleri) in previous assays, respectively [Citation7,Citation23], were included. The experiments were arranged in a completely randomized design in six replications with 10 plants of each genotype per replication for seedling stage in a single seedling nursery tray for every genotype filled with substrate of oven-sterilized mixture of sand-peat moss in equal parts. For 50% flowering stage, three replications in 5 kg plastic pots filled with sterilized substrate of soil, sand and peat moss (1:1:1), each of which consisted of two plants in every pot without any inoculation. When plants had the first pair of true leaves fully expanded, they were removed from the tray cells and transplanted into plastic pots. The experiments were repeated twice ().
Table 1. Characteristic of cucumber genotypes including abbreviation, breeding company and reaction to damping-off disease at seedling and 50% flowering stages.
The cucumber plants at seedling and 50% flowering stages were inoculated with P. melonis isolate, as described by Nasr Esfahani et al. [Citation7,Citation24]. The pathogen, P. melonis was isolated from naturally infected cucumber plants exhibiting post-emergence damping-off and root rot symptoms and identified as P. melonis (MH924841). Koch’s postulates were conducted by re-isolation of the inoculated fungi.
Data analysis of screening test
Twenty days after inoculation, the percent of damping-off in each replication was calculated using the following formula given by Singh et al. [Citation25]. The status of the cucumber genotypes was determined and categorized into five groups based on their percentage of disease incidence (PDI): (1) 0% highly resistant (HR); (2) 0.1–10%, resistant (R); (3) 10.1–25%, moderately resistant (MR); (4) 25.1–50%, susceptible (S); (5) 50.1–100%, highly susceptible (HS) [Citation6,Citation26,Citation27]. The data were converted with Asin √x + 0.5 for homogeneity. Analysis of variance was performed using SAS (ver. 9.4, SAS Institute, Cary, NC) and means compared with least significant difference (LSD).
Genetic diversity analysis
Genomic DNA extraction and PCR amplification using ISSR primers
To increase the efficiency in the analyses, leaves from three randomly chosen plants per genotype were bulked into a single sample. Genomic DNA was isolated from about 1 g of young leaf tissue from each bulk using Cetyl Trimethyl Ammonium Bromide (CTAB)- based method with some modifications [Citation28]. The quality of extracted DNA was examined by 1% agarose gel electrophoresis and NanoDrop technique (IMPLEN, NP80). The final DNA concentration of each template stock was adjusted to 20 ng·µL−1. The PCR reaction was conducted using 15 ISSR primers ().
Table 2. Details and parameters of genetic variation generated by ISSR markers.
The PCR amplification was performed in a 20-µL volume containing approximately 20–50 ng template DNA, 10 pmol of each primer, and 10 µL 2× Master mix Red (Ampliqon, Odense, Denmark). Reactions were carried out using the Eppendorf Gradient Mastercycler (Eppendorf, Hamburg, Germany) with an initial denaturation step of 10 min at 94 °C, followed by 45 cycles at 94 °C for 45 s to 1 min (depending on primer sequence, ) and 72 °C for 120 s, and a final extension at 72 °C for 10 min. The PCR-amplified fragments were separated by vertical electrophoresis in 12% (w/v) polyacrylamide gel in 0.5× TBE buffer, visualized by silver nitrate staining and photographed [Citation29,Citation30].
DNA bands were treated as dominant markers and scored in terms of a binary code as presence (1) or absence (0). The binary data were used to calculate the levels of polymorphism by separating the polymorphic bands from the total scored bands. The utility of the ISSR primer was measured by polymorphism information content (PIC), marker index (MI) and resolving power (RP).
Data were analyzed using the Similarity for Qualitative Data (SIMQUAL) routine to generate a Jacquard similarity coefficient. The similarity coefficient was used to construct a dendrogram with the Unweighted Pair Group Method with Arithmetic Average (UPGMA) algorithm using the Numerical Taxonomy and Multivariate Analysis System (NTSYS), version 2.1 [Citation31]. Principal Component Analysis (PCA) in NTSYS-PC 2.1 was used to analyze genetic relationships among 38 genotypes of cucumber without any missing ISSR data in a two-dimensional mode and determine the optimal number of clusters in the study.
Quantification of involving related genes
RNA extraction and cDNA synthesis
One resistant genotype ‘Ramezz’, two MR genotypes ‘Baby’ and ‘Orzu’ and two HS genotypes ‘Bosco’ and ‘Extrem’ were re-inoculated by P. melonis and maintained as described above. Total RNA was isolated 72 h after inoculation from collar tissues of cucumber genotypes using AccuZolTM kit (Bioneer, Korea) according to the manufacturer’s protocol. The extracted total RNA treated with DNase I (Thermo Scientific) to eliminate the presence of residual genomic DNA. The quantity and purity of total RNA was evaluated using a Nanodrop NP80 spectrophotometer (Implen Germany). The cDNA of the cucumber genotypes inoculated with P. melonis and the control ones with no inoculations was synthesized from 5 μg of total RNA using the cDNA Synthesis commercial kit (YTA, Yekta Tajhiz Azma, Iran) according to the manufacturer’s instructions.
Primer design and relative quantification of gene expression
WRKY transcription factor 20 (CsWRKY20), receptor-like kinase 6.1 (CsLecRK6.1) and LOX1 gene sequences of cucumber were downloaded from the NCBI site (NCBI, http://www.ncbi.nlm.nih.gov/). Gene-specific primers were designed using Oligo7 software [Citation32]. The primer sequences and their amplicon characteristics for each gene are presented in .
Table 3. Oligonucleotide primer sequences and real-time PCR amplification parameters.
RT-qPCR
The mRNA expression levels of selected probes were analyzed by quantitative real-time PCR (qPCR) using SYBR Green PCR Master Mix (2X) (Biofact, South Korea) according to the manufacturer’s protocols. The RT-qPCR was performed with preliminary hold at 95 °C for 15 min, 45 cycles of 95 °C for 15 s, annealing temperature depending on the primer Tm for 25 s and 72 °C for 40 s (). The experiment was performed with three biological replicates for each sample and two technical replicates. Primer efficiencies (E) for all primers and Ct values were determined using LinRegPCR software version 2012.3 [Citation33] and relative gene expression was calculated by Pfaffl formula [Citation34]:
In this study, the cucumber actin gene (XM_004147305.2) was used as the internal reference for the normalization of expression data [Citation30,Citation35,Citation36].
Statistical analysis
The experiment was conducted in a completely random design with three replications and data analysis was performed using the StepOne™ Software v2.3. The significances of data were evaluated by analysis of variance (ANOVA) using SPSS ver. 18 [Citation37] followed by Fisher's LSD test (p < 0.01).
Results and discussion
Screening cucumber genotypes for resistance/susceptibility to damping-off
There was a significant difference in percent damping-off among genotypes at the seedling and 50% flowering stages. Controls had no infection or damping-off. At transplanting, the screening of cucumber genotypes against P. melonis identified one resistant genotype, ‘Ramezz’ and one MR genotype, ‘Baby’ (; ). At 50% flowering, ‘Ramezz’ exhibited resistance and the genotypes ‘Baby’, ‘Orzu’ and ‘Sina’ displayed moderate resistance (; ). At neither stage were any genotypes immune. Highly infected genotypes tended to be associated with a high mean score of 50–100% PDI and the least infected genotype had the lowest scores of 0–10%. According to screening under greenhouse conditions, the majority of cucumber genotypes were susceptible and HS to P. melonis, which corroborates previous results obtained in screening germplasm collections of other Cucurbitaceae hosts for resistance to Phytophthora species [Citation11,Citation38].
Figure 1. Wilting variation of cucumber genotypes at seedling (A and B) and maturing stages, before (C) and after (D) inoculation with P. melonis. Note: R: resistant; MR: moderately resistant, HS: highly susceptible.
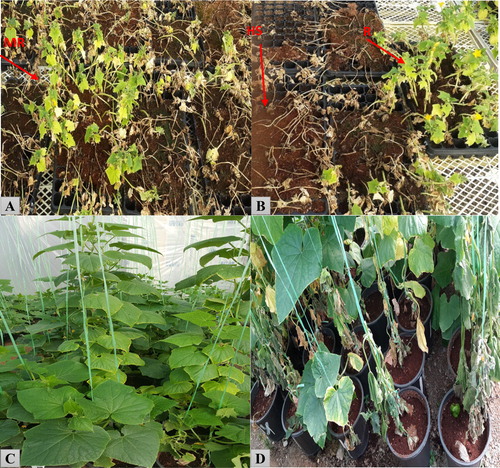
The correlation between percent damping-off at seedling stage and 50% flowering was highly significant (r = 0.96). Cluster analysis of the combination of percent damping-off at seedling and 50% flowering stages separated the genotypes into four distinct groups, which matched the results of the LSD (). The genotype ‘Ramezz’ was the most resistant to this isolate in all growing stages, and may be a potentially useful source of resistance to damping-off and can be used in damping-off management programs.
Figure 2. Ward’s clustering dendrogram of cucumber genotypes based on resistance to P. melonis. Note: Cluster A and B consisted of highly susceptible genotypes (PDI = 5), Cluster C included susceptible genotypes (PDI = 4), Cluster D consisted of resistant and moderately resistant genotypes (PDI = 2 and 3).
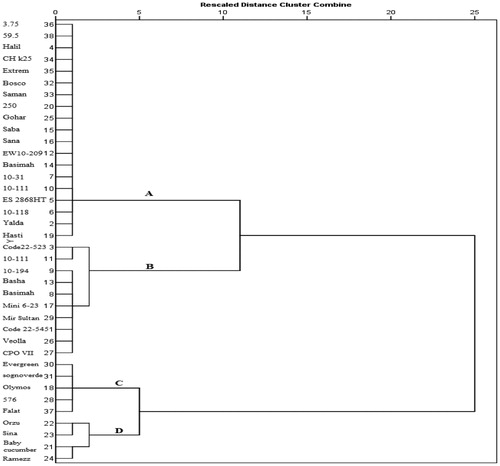
ISSR analysis
Of 30 ISSR primers, 15 ISSR primers could amplify all 38 cucumber genotypes (). The total number of bands amplified varied between 18 and 24 with the average of 21.13 bands per primer (). Sikdar et al. [Citation12] found an average of 17.37 bands per ISSR primer. Punetha et al. [Citation16] and Singh et al. (Citation17] observed numbers of alleles of 8.66 and 9.5, respectively. In this study, the average number of polymorphic bands generated by ISSR was higher (19.80/primer) compared to those in Punetha et al. [Citation16], who reported an average of eight polymorphic bands/primer in 13 genotypes of cucumber. Singh et al. [Citation17] found an average of 8.17 bands per primer among 11 genotypes of cucumber. The higher average number of polymorphic bands could be attributed to the greater number of cucumber genotypes used in this study (). The ISSR primers with dinucleotide motifs (AC)n, (CT)n and trinucleotide motifs (CAC)n produced a high level of polymorphism ().
The utility of ISSR primers was measured by PIC, MI and RP. The mean PIC for all 15 ISSR primers was 0.39 with values ranging from 0.34 for the ISSR primers 9, 11 and 22, to 0.45 for the ISSR primer 29. Botstein et al. [Citation39] reported that PIC value >0.5 is considered as a highly informative marker, while 0.25 < PIC < 0.5 is just an informative marker and PIC <0.25 is a slightly informative marker. As a result, the PIC scores are considered to have high discriminating power and are informative to separate genotypes. Singh et al. [Citation17] reported that the PIC values in cucumber ranged from 0.19 and 0.34. Punetha et al. [Citation16] evaluated 13 cucumber genotypes, using ISSR markers and the highest and lowest PIC valuesw were 0.90 and 0.31, respectively. To determine the overall usefulness of the system markers used, the MI was calculated for each ISSR primer (). The highest MI was observed with the ISSR primer 29 and the lowest in the ISSR primers 9 and 22. The RP indicates the discriminatory potential of the primer chosen. The highest RP value was observed with the primer ISSR29 and the lowest with the primer ISSR11. The significant, positive, correlation between PIC and MI values (r2 = 0.96, p < 0.01), indicated that the information obtained for cucumber with ISSR markers is robust. High RP values, with positive correlations with PIC and MI, indicate high descriptive potential of diversity between cucumber genotypes. Although the RP value is less than the one reported by Payel et al. [Citation40], it is higher than the one reported by Dar et al. [Citation41], who analyzed 104 genotypes of cucumber using 23 SSR primer pairs. ISSR29 was most informative among the 15 primers because of its higher PIC, MI and RP values. This marker was the best marker to separate different cucumber genotypes.
Clustering and principal component analysis
The dendrogram provided the best resolution of relationships between genotypes, which could be separated into five main clusters with varying genetic similarity coefficients (). The grouping of the genotypes in the clustering analysis was further compared with their disease resistance responses. The clustering pattern of the cucumber genotypes based on the ISSR markers was partly in agreement with the groupings based on disease resistance responses and indicated only one cluster containing resistant and MR genotypes. All four genotypes grouped in cluster A were HS to damping-off based on greenhouse screening. Cluster B comprised four genotypes, of which ‘Ramezz’ was resistant and ‘Baby’, ‘Orzu’ and ‘Sina’ were MR to damping-off. Cluster C consisted of 18 HS and five susceptible genotypes. Cluster D included three HS genotypes. The last cluster was comprised of three HS genotypes. When the similarity index between clusters increases, it indicates the presence of common parents among genotypes. Each of the clusters could be divided into several subgroups.
Figure 3. Grouping of cucumber genotypes and lines using the UPGMA method based on Jaccard’s similarity coefficient of 15 ISSR molecular markers. Note: Major clusters are marked on the right side of the dendrogram. The numbers represent cucumber genotypes listed in .
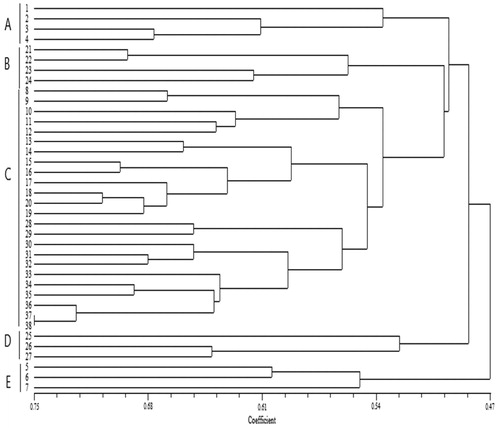
Genetic similarity in this study implied a high level of genetic variation between genotypes. This result is comparable with the results of Mohammed et al. [Citation42], who reported that genetic similarity coefficients ranged between 0.15 and 0.62 in 10 cucurbit species using 13 RAPD primers. Dar et al. [Citation41] stated that Jaccard’s similarity coefficient ranged from 0.07 to 0.89 for 104 genotypes of cucumber using 23 SSR primer pairs. Considering the percent of total genetic diversity, the results may not be statistically appropriate for PCA but genetically they indicate the desirable sampling of ISSR markers of the 38 cucumber genotypes. The PCA grouped genotypes in a two-dimensional space with a clustering pattern similar to the dendrogram with some discrepancies (). Combining the variety information, in group B/cluster II, the genotypes were resistant, and MR to damping-off, whereas the other groups/clusters showed no resistance. This indicated that there might exist a connection between cucumber genotype and resistance level against damping-off. The present findings confirmed previous studies demonstrating association between molecular marker clusters and disease resistance response [Citation13,Citation30,Citation43].
RT-qPCR analysis of gene expression
Complementary experiments showed that LOX1, CsWRKY20 and CsLecRK6 genes were highly expressed in the infected resistant genotypes of cucumber in response to P. melonis infection 72 h after inoculation (). Although the relative expression of these genes in ‘Baby’ and ‘Orzu’ (moderate resistance) was significant, the expression level was lower than that in ‘Ramezz’. The transcriptional levels of these genes were 8.42-, 5.61- and 5.45-fold higher in the resistant ‘Ramezz’ than in the control ones, respectively ().
Figure 5. Patterns of differential gene expression levels of (A) CsLecRK6.1, (B) CsWRKY20 and (C) LOX1genes in resistant (‘Ramezz’), moderately resistant (‘Baby’ and ‘Orzu’) and highly susceptible (‘Bosco’ and ‘Extrem’) genotypes of cucmber at 72 hpi after inoculation with P. melonis. (D) Radar plots of relative gene expression showed that CsLecRK6.1, CsWRKY20 and LOX1 genes were highly expressed in the infected resistant cucumber in response to P. melonis infection. Note: To determine the RE ratio, transcript level in control plants for each cucumber genotype was chosen as a baseline and genotypes were compared to it.
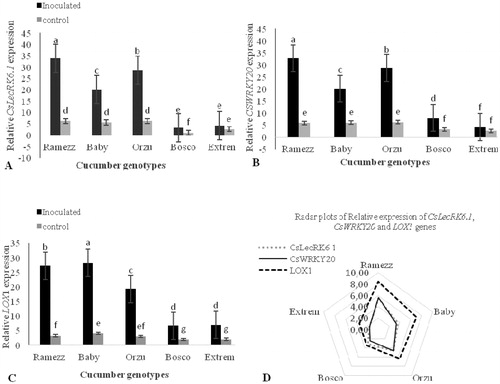
LOX1 was revealed to be the most expressed gene, whose expression increased from the most highly resistant to the most susceptible genotype. In MR ‘Baby’ and ‘Orzu’, LOX1 transcript levels were approximately 7.05-fold and 6.39-fold greater than in their control plants, respectively. Lipoxygenase, encoded by the LOX1 gene, is the first enzyme in the biosynthesis pathway of jasmonate (JA) [Citation44]. JA is known to play major roles in the regulation of the plant immune system. Pathogen-induced LOX transcript accumulation has been reported in cucumber after inoculation with F. oxysporum CS-20 [Citation45]. Although, this interaction is not the same, we found a similar pattern in our experiment, in which LOX1 showed activation after inoculation with P. melonis.
The expression of CsWRKY20 and CsLecRK6.1 gene from C. sativus was significantly induced after infection by P. melonis in the resistant and MR genotypes in this study. Comparatively, with resistant and MR genotypes, the HS ‘Extrem’ demonstrated no significant change in the transcriptional activity of CsWRKY20 and CsLecRK6.1 genes relatively to the control group, which demonstrated worse adaptability to biotic stress. According to these results, ‘Ramezz’ can be regarded as one of the most resistant cucumber genotypes here (; ). There is a large amount of evidence that suggests a critical role of WRKY transctiption factors (TFs) in regulating defence-related genes and increasing the resistance to pathogens in several plant species [Citation46]. Our findings are in accordance with previous studies which have demonstrated the association of WRKY TFs in the resistance response against oomycete phytopathogens [Citation22,Citation47]. Therefore, this is not surprizing considering the fact that CsWRKY20 in cucumber might have a similar role during the interaction between cucumber and P. melonis. Further research is needed to clarify the role of the CsWRKY20 gene in cucumber signalling in response to P. melonis infection.
Multiple studies have revealed the significant and outstanding role of LecRKs in plant innate immunity on several aspects, which makes them fascinating as potential resistance components in plants [Citation20,Citation21]. Thus, the expression information of the CsLecRK6.1 gene will be useful for further investigating the function of this gene under various biotic stress conditions.
There were significant negative correlations between the expression levels of these genes in the studied genotypes and the PDI (i.e. higher expression of these genes in cucumber genotypes led to a lower PDI when challenged with P. melonis).
Conclusions
Considering the adverse impact of damping-off disease or root and crown rot on the successful production of cucumber, it is necessary to develop new resistance sources to minimize yield loss. The screening test provided information in identifying resistant and MR cucumber genotypes to damping-off, which would be useful for disease management. It provided insights into genetic diversity and estimation of genetic variation among resistant and susceptible cucumber hybrids and inbreeding lines. We believe that ISSR markers could be useful in studying cucumber resistance, thereby, improving cucumber breeding and conservation. In this study, in the resistant and MR genotypes, higher expression of LOX1, CsLecRK6.1 and CsWRKY20 genes is the possible reason for resistance. Although, this is a preliminary study on C. sativus/P. melonis interactions, it could be useful to understand the defence mechanisms of cucumber. This study is part of an ongoing Agricultural Research, Education and Extension Organization (AREEO) breeding program to introduce resistance to P. melonis in C. sativus. Increasing genome information and identification of candidate germplasm for novel sources of damping-off resistance may contribute to the development of strategies to control damping-off disease in cucumber, which may include early selection of resistant genotypes.
Acknowledgments
The authors thank Plant Protection Research Division, Isfahan Center for Agricultural and Natural Resources Research and Education, Isfahan, Iran for providing laboratory facilities.
Disclosure statement
The authors declare that the research was conducted in absence of any commercial or financial relationships that could be construed as a potential conflict of interest.
References
- Fukino N, Yoshioka Y, Kubo N, et al. Development of 101 novel SSR markers and construction of an SSR-based genetic linkage map in cucumber (Cucumis sativus L.). Breed Sci. 2008;58(4):475–483.
- Staub J, Chung SM, Fazio G. Conformity and genetic relatedness estimation in crop species having a narrow genetic base: the case of cucumber (Cucumis sativus L.). Plant Breed. 2005;124(1):44–53.
- FAOSAT. Crops. Rome (Italy): Food and Agriculture Organisation of the United Nations. 2017. Available from: http://www.fao.org/faostat/en/#data/QC
- Erwin DC, Ribeiro OK. 1996. Phytophthora disease worldwide. St. Paul (MN): APS Press.
- Wu T, Wang R, Xu X, et al. Cucumis sativus L-type lectin receptor kinase (CsLecRK) gene family response to Phytophthora melonis, Phytophthora capsici and water immersion in disease resistant and susceptible cucumber cultivars. Gene. 2014;549(2):214–222.
- McGrath MT. 2001. Vegetable MD online: Phytophthora blight of cucurbits. Cooperative extension. Ithaca (NY): Cornell University [cited August 2006]. Available from: http://vegetablemdonline.ppath.cornell.edu/factsheets/Cucurbit_Phytoph2.htm
- Nasr Esfahani M, Chatraee M, Shafizadeh S, et al. Evaluation of resistance of cucurbit and cucumber cultivars to Phytophthora drechsleri in Greenhouse. Seed Plant Improv J. 2012;28:407–417.
- Hatami N, Aminaee MM, Zohdi H, Tanideh T. Damping-off disease in greenhouse cucumber in Iran. Arch Phytopathol Plant Protect. 2013;46(7):796–802.
- Mansoori B, Banihashemi Z. Evaluating cucurbit seedling resistance to Phytophthora drechsleri. Plant Dis. 1982;66(1):373–376.
- Colle M, Straley EN, Makela SB, et al. Screening the cucumber plant introduction collection for young fruit resistance to Phytophthora capsici. HortScience. 2014;49(3):244–249.
- Nazavari K, Jamali F, Bayat F, et al. Evaluation of resistance to seedling damping-off caused by Phytophthora drechsleri in cucumber cultivars under greenhouse conditions. Biol Forum. 2016;8:54–60.
- Sikdar B, Bhattacharya M, Mukherjee A, et al. Genetic diversity in important members of Cucurbitaceae using isozyme, RAPD and ISSR markers. Biol Plant. 2010;54(1):135–140.
- Innark P, Ratanachan T, Khanobdee C, et al. Evaluation of genetic diversity in cucumber (Cucumis sativus L.) germplasm using agro-economic traits and microsatellite markers. Sci Hortic. 2013;162:278–284.
- Valcárcel JV, Pérez-de-Castro A, Díez MJ, et al. Molecular characterization of the cucumber (Cucumis sativus L.) accessions of the COMAV’s genebank. Span J Agric Res. 2018;16(1):e0701.
- Pandey S, Ansari WA, Pandey M, et al. Genetic diversity of cucumber estimated by morpho-physiological and EST-SSR markers. Physiol Mol Biol Plants. 2018;24(1):135–146.
- Punetha S, Singh DK, Singh NK. Genetic diversity assessment of cucumber (Cucumis sativus L.) genotypes using molecular markers. Electron J Plan Breed. 2017;8(3):986–991.
- Singh DK, Rajani T, Singh NK, et al. Genetic diversity cucumber using inter simple sequence repeats (ISSR). Transcriptomics. 2016;4:129.
- Reddy MP, Sarla N, Siddiq EA. Inter simple sequence repeat (ISSR) polymorphism and its application in plant breeding. Euphytica. 2002;128(1):9–17.
- Porta H, Rocha-Sosa M. Plant lipoxygenases. Physiological and molecular features. Plant Physiol. 2002;130(1):15–21.
- Wang Y, Bouwmeester K. L-type lectin receptor kinases: new forces in plant immunity. PLoS Pathog. 2017;13(8):e1006433.
- Zhao T, Wang J, Zhang B, et al. Genome-wide analysis of lectin receptor-like kinases in tomato (Solanum lycopersicum) and its association with the infection of tomato yellow leaf curl virus. Plant Mol Biol Rep. 2018;36(3):429–438.
- Xu X, Wang R, Chao J, et al. The expression patterns of Cucumis sativus WRKY (CsWRKY) family under the condition of inoculation with Phytophthora melonis in disease resistant and susceptible cucumber cultivars. Can J Plant Sci. 2015;95(6):1121–1131.
- Ghaderi F, Askari S, Abdollahi M. Isolation and identification of Phytophthora species on greenhouse cucumber in Southern Kohgiluyeh and Boyerahmad province and evaluation of relative resistance of greenhouse cucumber cultivars to them. J Plant Prod. 2011;18(3):31–45.
- Nasr Esfahani M, Nasehi A, Rahmanshirazi P, et al. Susceptibility assessment of bell pepper genotypes to crown and root rot disease. Arch Phytopathol Plant Protect. 2014;47(8):944–953.
- Singh V, Gupta RP, Singh AK. Screening of chickpea for resistance to Fusarium wilt. Field Crops Res. 1987;61(3):415.
- Nasr Esfahani M, Ansari Pour B. The variable differences in onion cultivars to pink root rot disease in Iran. J Gen Plant Pathol. 2008;74(1):46–244.
- Nasr Esfahani M. Genetic and virulence variation in Fusarium oxysporum f. sp. cepae causing root and basal rot of common onion in Iran. J Phytopathol. 2018;166(7–8):572–580.
- Stewart CN, Jr, Via LE. A rapid CTAB DNA isolation technique useful for RAPD fingerprinting and other PCR applications. Biotechniques. 1993;14(5):748–749.
- Wang D, Shi J, Carlson SR, et al. A low-cost, high-throughput polyacrylamide gel electrophoresis system for genotyping with microsateilite DNA markers. Crop Sci. 2003;43(5):1828–1832.
- Moghadam GA, Rezayatmanda Z, Nasr Esfahania M, et al. Genetic defense analysis of tomatoes in response to early blight disease, Alternaria alternate. Plant Physiol Biochem. 2019;142:500–509.
- Rohlf FJ. 2002. NTSYS-pc. Numerical taxonomy and multivariate analysis system, Version 2.1. New York (NY): Applied Biostatistics.
- Rychlik W. OLIGO 7 primer analysis software. In: Yuryev A, editor. PCR primer design. Totowa: Humana Press; 2007.
- Ruijter JM, Ramakers C, Hoogaars WM, et al. Amplification efficiency: linking baseline and bias in the analysis of quantitative PCR data. Nucleic Acids Res. 2009;37(6):e45.
- Pfaffl MW. A new mathematical model for relative quantification in real-time RT-PCR. Nucleic Acids Res. 2001;29(9):e45.
- Wan HJ, Zhao ZG, Qian CT, et al. Selection of appropriate reference genes for gene expression studies by quantitative real-time polymerase chain reaction in cucumber. Anal Biochem. 2010;399(2):257–261.
- Castellano M, Pallas V, Gomez G. A pathogenic long noncoding RNA redesigns the epigenetic landscape of the infected cells by subverting host histone deacetylase 6 activity. New Phytol. 2016;211(4):1311–1322.
- Levesque R. 2007. SPSS programming and data management. A Guide for SPSS and SAS Users. 4th ed. Chicago, IL: SPSS Inc.
- Tian D, Babadoost M. Host range of Phytophthora capsici from pumpkin and pathogenicity of isolates. Plant Dis. 2004;88(5):485–489.
- Botstein D, White RL, Skolnick M, et al. Construction of a genetic linkage map in man using restriction fragment length polymorphism. Am J Hum Genet. 1980;32(3):314–331.
- Payel D, Mala P, Sunita S. Inter-genus variation analysis in few members of Cucurbitaceae based on ISSR markers. Biotechnol Equip. 2015;29(5):882–886.
- Dar AA, Mahajan R, Lay P, et al. Genetic diversity and population structure of Cucumis sativus L. by using SSR markers. 3 Biotech. 2017;7:307.
- Mohammed IA, Gumaa AGN, Kamal MN, et al. Genetic diversity among some Cucurbits species determined by random amplified polymorphic DNA RAPD marker. Int J Plant Res. 2012;2(4):131–137.
- Wang Z, Zhang Y, Dai F, et al. Genetic diversity among mulberry genotypes from seven countries. Physiol Mol Biol Plants. 2017;23(2):421–427.
- Wasternack C, Hause B. Jasmonates: biosynthesis, perception, signal transduction and action in plant stress response, growth and development. An update to the 2007 review in Annals of Botany. Ann Bot. 2013;111(6):1021–1058.
- Pu X, Xie B, Li P, et al. Analysis of the defense-related mechanism in cucumber seedlings in relation to root colonization by nonpathogenic Fusarium oxysporum CS-20. FEMS Microbiol Lett. 2014;355(2):142–151.
- Alves MS, Dadalto SP, Goncalves AB, et al. Transcription factors functional protein-protein interactions in plant defense responses. Proteomes 2014;2(1):85–106.
- Cui J, Xu P, Meng J, et al. Transcriptome signatures of tomato leaf induced by Phytophthora infestans and functional identification of transcription factor SpWRKY3. Theor Appl Genet. 2018;131(4):787–800.