Abstract
The aim of the present study was to identify key genes and molecular biological processes that are responsive to CO2 enrichment in tomato (Solanum lycopersicum L.). Tomato plants were exposed to CO2 concentrations of either 350–500 μmol/mol (ambient) or 750–850 μmol/mol, and genes that were differentially expressed (DEGs) in the two groups were identified. Gene ontology (GO) and Kyoto Encyclopedia of Genes and Genomes (KEGG) pathway enrichment analysis were then used to identify DEGs involved in photosynthesis and sugar synthesis. As a result, a total of 208 DEGs, including 179 up-regulated and 29 down-regulated genes, were identified. GO functional annotation revealed that the DEGs were mainly enriched in the processes of photosystem I, photosystem II, photosynthesis phosphate ion binding, and electron carrier activity, whereas the KEGG pathway analysis indicated that the DEGs were mainly enriched in 21 signalling pathways, including plant hormone signal transduction, RNA polymerase, ribosomes and photosynthesis. A total of 62 DEGs, including Solyc12g032970.1, Solyc12g032960.1, Solyc07g043480.1 and Solyc02g086650.2, were associated with photosynthesis and sugar synthesis. The present study provides a basis for investigating the effects of elevated CO2 on the molecular processes of tomato, which will, in turn, provide a theoretical basis for tomato breeding programs.
Introduction
The effects of rising atmospheric CO2 concentrations have attracted increasing attention, since these increases are considered the most prominent cause of global climate change, and an increasing number of studies have reported that atmospheric CO2 levels significantly affect global agriculture and food security [Citation1–3]. Briefly, these studies have reported that CO2 enrichment stimulates the photosynthesis and carbohydrate accumulation of plants, which could potentially increase plant yield and concentrations of starch, sugars, ascorbic acid and organic acids [Citation4, Citation5]. Actually, for different plant species, the capacity to tolerate elevated atmospheric CO2 relies on a series of physiological and biochemical processes.
In response to long-term CO2 enrichment, tomato (Solanum lycopersicum L.), which is an important annual vegetable crop, has been reported to exhibit foliar deformation and discoloration [Citation6]. Carbon dioxide enrichment has also been reported to influence the growth, yield and gas exchange of tomato [Citation7]. For example, elevated CO2 levels might also alleviate heat stress by modulating antioxidant defence systems [Citation8], and Jayawardena et al. [Citation9] reported that the combination of elevated CO2 levels and chronic warming caused dramatic increases in leaf angle, which is correlated with reduced plant growth. However, the molecular mechanisms of the responses of tomato plants to elevated CO2 levels remain poorly understood.
With the development of microarrays and next-generation sequencing, transcriptome analysis has been widely used to investigate the molecular mechanisms underlying the responses of plants to a variety of abiotic stressors. For example, Min et al. [Citation10] used transcriptional analysis and found that DkNAC genes, which are potentially important regulators of persimmon astringency, responded differentially to CO2 treatment in persimmon, whereas Ma et al. [Citation11] identified candidate sugar metabolism genes that were differentially expressed under different CO2 concentrations in goji berry. Similarly, the identification of key genes, transcriptional factors, and metabolic pathways would help elucidate the molecular mechanisms underlying the responses of tomato to CO2 enrichment.
Accordingly, the aim of the present study was to identify genes that were differentially expressed (DEGs) in tomato plants grown under ambient and elevated CO2 levels and to investigate the functions of those genes using Gene Ontology (GO) and Kyoto Encyclopedia of Genes and Genomes (KEGG) analyses.
Materials and methods
Plant materials and experimental treatments
The tomato cultivars of ‘Hong Zhixing’ were planted under short-day conditions (8 h illumination, 16 h darkness) in an enclosed solar greenhouse [Citation12]. The plants were divided into control and CO2 enrichment groups and were exposed to CO2 concentrations of 350–500 (ambient) or 750–850 μmol/mol, respectively [Citation13]. For the CO2 enrichment group, CO2 was applied from 8:00 to 10:00 in the morning, when the flowers of the first ear of tomato were in bloom (depending on weather). For the control group, the conditions were the same as those used for the CO2 enrichment, except that no CO2 was applied. When the tomato plants began to bear fruit, three plants with similar growth patterns were selected from each group when the morning light was best and plant activity was high, and three highly metabolized leaves, each weighing about 0.15 g, were collected from the selected plants, frozen immediately in liquid nitrogen, and stored at −80 °C until RNA extraction.
RNA extraction and sequencing
Total RNA extraction was conducted using an RNeasy Plus Universal Mini Kit (Qiagen, Germany), according to the manufacturer’s instructions. The quality and quantity of the extracted RNA were determined using an Agilent Bioanalyzer 2100 (Agilent Technologies, Santa Clara, CA, USA). Transcriptome sequencing analysis was performed by Beijing Baimichael Biotechnology Co., Ltd., using an Illumina HiSeq 2500 high-throughput sequencing platform (Biomarker Technologies Co., Ltd., Beijing, China). The resulting raw data were filtered to remove adapter sequences, empty reads, and low-quality reads.
Bioinformatics analysis
Based on TopHat2, the reads obtained from the transcriptome sequencing procedure were sequenced with a tomato reference genome, and DEGs were identified using EBSeq software [Citation14], with a false discovery rate (FDR) threshold of <0.05 and fold change (FC) threshold of ≥2. GO and KEGG analyses of the DEGs were then performed using DAVID [Citation15], with a count threshold of ≥2 and p-value threshold of <0.05.
Validation of DEGs
To validate the identified DEGs, three genes (Solyc01g017310.1.1, Solyc10g052740.1.1 and Solyc01g007350.2.1) were randomly selected for quantitative real-time polymerase chain reaction (qRT-PCR) analysis, using actin as the internal control gene. Tomato leaves were exposed to different CO2 concentrations, and total RNA was extracted from the leaves using the PrimeScript RT reagent kit (Perfect Real Time; TaKaRa). Next, qRT-PCR analysis was performed using SYBR Premix Ex Taq II (Tli RNaseH Plus; TaKaRa), according to the manufacturer’s instructions and using the following primers: Solyc01g017310.1.1 CCATTTGGTAGGGCTACTAG (F) and ATCTACTCCAGCGTTTACAA (R); Solyc10g052740.1.1 GCGAGCATCTGGAATAACT (F) and CGGATAGTGATTCAGCATAGA (R); Solyc01g007350.2.1 GATCAGGATCTCTGGATGG (F) and ACTCTCATCAACTAGCACTA (R); actin GATGGTGGGTATGGGTCAAA (F) and AGGGGCTTCAGTTAGGAGGA (R). Relative expression levels were calculated using the 2−ΔΔCt method.
Results and discussion
Quality of RNA-Seq results
After data cleaning and quality checking, a total of 28.70 Gb clean high-quality data remained, among which, the clean data of each sample reached 6.11 Gb, and the percentage of Q30 bases was ≥90.02%. We then assessed the sequencing quality of the detected reads and constructed four cDNA libraries: FQD1, FQD2, FQF1, and FQF2. As illustrated in , the GC content of the four libraries ranged from 42.94 to 43.36%, which suggested that the content of G, C, A and T bases in the libraries were similar and that no base separation had occurred, thereby indicating that the sequencing process was relatively stable. Meanwhile, 87.13, 87.35, 86.79 and 87.25% of the reads in the four libraries were mapped to the reference genome, respectively, which indicated that the alignment efficiency was high and that the reference genome selection was reliable. Together, these results indicated that the transcriptome was successfully sequenced and could be subject to further bioinformatics analyses.
Table 1. Quality assessment of the sequencing results of each library.
Differentially expressed genes (DEGs)
To investigate the effect of CO2 enrichment on the global transcription of tomato plants, the present study identified DEGs among the transcriptomes of tomato plants grown under different CO2 concentrations. A total of 208 genes were significantly differentially expressed, of which 179 and 29 DEGs were up- and down-regulated, respectively (). Furthermore, when 2 < FC ≤ 10, 177 DEGs were identified, including 153 up-regulated and 24 down-regulated genes; when 10 < FC ≤ 50, 29 DEGs were identified, including 25 up-regulated and four down-regulated genes; and when FC > 50, only Solyc09g011150.1 and Solyc11g030380.1 were identified as DEGs and were up- and down-regulated, respectively.
Table 2. Screening of differentially expressed genes (DEGs).
Putative DEG functions
To identify the putative functions of the 208 DEGs, GO functional annotation was performed, during which the transcripts are separated into three categories: biological process (BP), cellular component (CC) and molecular function (MF). As shown in , a total of 130 DEGs were successfully assigned to 126 items of BP, 35 items of CC and 81 items of MF. Moreover, the most significantly enriched GO terms were photosystem I, photosystem II, photosynthesis phosphate ion binding, and electron carrier activity. Meanwhile, KEGG pathway enrichment analysis indicated that the DEGs were mainly enriched in 21 signalling pathways, which included zeatin biosynthesis, plant hormone signal transduction, oxidative phosphorylation, RNA polymerase, pyrimidine metabolism, ribosomes, photosynthesis and plant–pathogen interaction ().
Photosynthesis- and sugar synthesis-related DEGs
Based on the GO function and KEGG pathway enrichment analyses, the DEGs related to photosynthesis and sugar synthesis were screened. As shown in , the DEGs enriched in photosystem I were upregulated and included Solyc12g032970.1, Solyc12g032960.1, Solyc10g017900.1, Solyc10g052740.1, Solyc01g017310.1, Solyc01g007350.2, Solyc01g017320.1, Solyc06g009950.1 and Solyc09g015290.1. Similarly, other upregulated DEGs, such as Solyc01g048590.1, Solyc01g007500.2, Solyc09g055950.1, Solyc12g027550.1, Solyc12g038080.1 and Solyc01g007500.2, were involved in photosystem I. Furthermore, Solyc01g007530.2, Solyc01g007540.2, Solyc07g032450.1, Solyc01g007310.2, Solyc08g061900.1 and Solyc02g011800.1 were found to be important in the electron transfer of cytochrome b6f and NADPH dehydrogenase activity.
Table 3. Identification of key genes related to photosynthesis and sugar synthesis.
In addition, the analysis of 32 DEGs from the plastid genome revealed that Solyc01g007280.2, Solyc01g007450.1, Solyc01g007480.1, Solyc01g007580.1, Solyc01g007610.2 and Solyc01g007620.2 were involved in ribosome synthesis, whereas Solyc01g007560.2, Solyc10g031550.1, Solyc10g031560.1 and Solyc12g096910.1 were involved in the regulation the of RNA polymerase activity in chloroplasts. In addition, Solyc01g006540.2 was involved in iron ion binding and REDOX process; whereas Solyc01g007640.2 was involved in ATP binding, and the proteins encoded by both of these genes were located in the chloroplast stroma.
With regard to sugar synthesis, Solyc07g043480.1 was found to regulate UDP-glucosyl transferase, and Solyc02g086650.2 was found to regulate 6-phosphate glucose transporter activity.
DEG validation
In order to verify the accuracy of the RNA-Seq results, the expression levels of three randomly selected DEGs were analyzed using qRT-PCR. As shown in , the results were in accordance with the transcriptome data. The expression levels of Solyc01g017310.1.1, Solyc10g052740.1.1 and Solyc01g007350.2.1 were increased by CO2 enrichment (p < 0.01).
Figure 3. Quantitative real-time PCR validation of RNA-Seq results. Three genes were randomly selected: Solyc01g017310.1.1 (A), Solyc10g052740.1.1 (B) and Solyc01g007350.2.1 (C). Actin was used as the internal control gene.
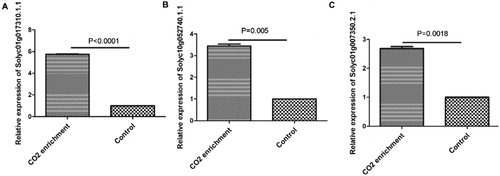
As atmospheric CO2 concentrations continue to increase, it is becoming more important to understand plant adaptations to rapidly changing climate conditions, especially in regards to food security. Several previous studies have investigated the short-term effects of high CO2 concentrations on the gene expression of food crops, such as grape [Citation16, Citation17], maize [Citation18] and rice [Citation19]. However, even though high CO2 concentrations have been reported to affect the growth and development of tomato plants [Citation6, Citation20] no studies have investigated the effects of high CO2 concentrations on the molecular-level processes of tomato until now.
In the present study, 208 DEGs were identified by comparing the transcriptomes of tomato plants grown under ambient (350–500 μmol/mol) and elevated (750–850 μmol/mol) levels of CO2. GO function and KEGG pathway enrichment analyses then revealed that the DEGs were mainly enriched in photosystem I, photosystem II, photosynthesis phosphate ion binding, and electron carrier activity, as well in plant hormone signal transduction, oxidative phosphorylation, RNA polymerase, and pyrimidine metabolism. DEGs involved in photosynthesis and sugar synthesis were also identified to understand the effects of elevated CO2 on the growth of tomato plants.
Previous studies have reported that, in the absence of CO2 in the atmosphere of greenhouses in winter, the addition of CO2 can increase growth rate, fruit setting rate, fruit yield and disease resistance of tomato plants [Citation2]. Moreover, Pan et al. [Citation20] reported that the elevated CO2 improved photosynthesis under high temperature by attenuating the functional limitations to energy fluxes, electron transport and redox homeostasis in tomato leaves. Together, these studies support the conclusion that high CO2 levels can promote photosynthesis in tomato plants.
Indeed, in line with previous studies, the present study identified 62 DEGs that were related to photosynthesis. Briefly, nine DEGs (e.g. Solyc12g032970.1 and Solyc12g032960.1), six DEGs (e.g. Solyc01g048590.1 and Solyc01g007500.2), three DEGs (e.g. Solyc01g007530.2 and Solyc01g007540.2), three DEGs (e.g. Solyc01g007310.2 and Solyc08g061900.1), and one DEG (Solyc11g007200.1) were involved in the electron transport system of photosystem I, electron transport system of photosystem II, electron transfer of cytochrome b6f, NADPH dehydrogenase activity, and biosynthesis of flavonoids, respectively. More importantly, all the DEGs were upregulated, which indicates that the genes play positive roles in photosynthesis.
Interestingly, both Solyc01g007330.2 and Solyc01g017320.1 were found to be involved in magnesium ion binding. Because Mg2+ is an important metal ion in the chlorophyll porphyrin ring [Citation21, Citation22] and because Mg2+ deficiency can impede chlorophyll functioning and prevent normal photosynthesis, it is possible that these genes regulate photosynthesis by regulating electron transfer processes.
The plant hormone auxin is essential for plant growth and development and controls both organ development and overall plant architecture [Citation23], among which, the gibberellin with two unrelated microRNA-controlled modules could coordinate the induction and flowering development of tomatoes [Citation24]. Moreover, Pan et al. [Citation25] reported that ethylene biosynthesis and signalling play critical roles in the heat stress responses of tomato plants grown under elevated CO2 levels. Indeed, the present study revealed that Solyc09g057580.2 and Solyc10g046870.1 were enriched in the gibberellin and ethylene mediated signalling pathways, respectively, and both were up-regulated, which indicates that CO2 enrichment promotes the nutritional growth of tomato and suggests that CO2 enrichment increases tomato yield.
The present study also revealed that Solyc07g043480.1, Solyc02g086650.2 and Solyc01g073640.2 might be involved in the regulation of UDP-glucosyl transferase, glucose-6-phosphate translocator, and glucose/ribitol dehydrogenase activities. In fact, sugar actually functions as a substrate for plant growth and also affects sugar sensing, thereby mediating changes in gene expression. Liu et al. [Citation26] reported that long-term exposure to elevated CO2 levels reduced the activity of fruit enzymes involved in sucrose synthesis and metabolism. Under high CO2 concentrations, rates of sucrose cycling might increase, owing to the enhanced provision of sucrose to leaf acid invertases [Citation27].
In tomato, CO2 enrichment significantly increases the contents of health-promoting compounds, including lycopene, and flavor-related parameters, such as sugar content, titratable acidity, and sugar:acid ratio [Citation28]. Moreover, a meta-analysis revealed that elevated atmospheric CO2 enhances the fructose, glucose, and total soluble sugar contents of tomato [Citation29]. The present study found that glucose metabolism-related genes were up-regulated, which indicated that photosynthetic products would be used for other metabolic pathways, thereby providing more energy for tomato growth and development.
Conclusions
The present study is the first to investigate the responses of tomato plants to long-term elevated CO2 levels using RNA-Seq. The present study identified a total of 208 DEGs that were mainly enriched in photosynthesis and sugar synthesis. These findings could improve the current understanding of the effects of elevated CO2 levels on the photosynthetic carbon assimilation mechanism, plant–pathogen interactions and molecular processes of tomato, which will, in turn, provide a theoretical basis for tomato breeding programs.
Acknowledgments
We would like to thank members of the Professor Li lab for helpful discussions and technical assistance.
Disclosure statement
No potential conflict of interest was reported by the authors.
Additional information
Funding
References
- Ainsworth EA, Long SP. What have we learned from 15 years of free‐air CO2 enrichment (FACE)? A meta‐analytic review of the responses of photosynthesis, canopy properties and plant production to rising CO2. New Phytol. 2004;165(2):351–372.
- Becklin KM, Walker SM, Way DA, et al. CO2 studies remain key to understanding a future world. New Phytol. 2017;214(1):34–40.
- Zhao H-Y, Jun-Qin G, Cun-Jie Z, et al. Climate change impacts and adaptation strategies in Northwest China. Adv Clim Change Res. 2014;5(1):7–16.
- Sun P, Mantri N, Lou H, et al. Effects of elevated CO2 and temperature on yield and fruit quality of strawberry (Fragaria × ananassa Duch.) at two levels of nitrogen application. PLoS One. 2012;7(7):e41000.
- Wei Z, Du T, Li X, et al. Interactive effects of elevated CO2 and N fertilization on yield and quality of tomato grown under reduced irrigation regimes. Front Plant Sci. 2018;9:328.
- Tripp KE, Peet MM, Pharr DM, et al. CO2-enhanced yield and foliar deformation among tomato genotypes in elevated CO2 environments. Plant Physiol. 1991;96(3):713–719.
- Yelle S, Beeson RC, Trudel MJ, et al. Duration of CO2 enrichment influences growth, yield, and gas exchange of two tomato species. J Am Soc Hortic Sci. 1990;115(1):52–57.
- Li X, Ahammed G, Zhang Y, et al. Carbon dioxide enrichment alleviates heat stress by improving cellular redox homeostasis through an ABA‐independent process in tomato plants. Plant Biol J. 2015;17(1):81–89.
- Jayawardena DM, Heckathorn SA, Bista DR, et al. Elevated carbon dioxide plus chronic warming causes dramatic increases in leaf angle in tomato, which correlates with reduced plant growth. Plant Cell Environ. 2019;42:1247–1256.
- Min T, Wang M-M, Wang H, et al. Isolation and expression of NAC genes during persimmon fruit postharvest astringency removal. Int J Mol Sci. 2015;16(1):1894–1906.
- Ma Y, Reddy VR, Devi MJ, et al. De novo characterization of the Goji berry (Lycium barbarum L.) fruit transcriptome and analysis of candidate genes involved in sugar metabolism under different CO2 concentrations. Tree Physiol. 2019.
- Chen Z-F, Kang X-P, Nie H-M, et al. Introduction of exogenous glycolate catabolic pathway can strongly enhances photosynthesis and biomass yield of cucumber grown in a low-CO2 environment. Front Plant Sci. 2019;10:702.
- Guo H, Sun Y, Ren Q, et al. Elevated CO2 reduces the resistance and tolerance of tomato plants to Helicoverpa armigera by suppressing the JA signaling pathway. PLoS One. 2012;7(7):e41426.
- Leng N, Dawson JA, Thomson JA, et al. EBSeq: an empirical Bayes hierarchical model for inference in RNA-seq experiments. Bioinformatics. 2013;29(8):1035–1043.
- Sherman BT, Lempicki RA. Systematic and integrative analysis of large gene lists using DAVID bioinformatics resources. Nat Protoc. 2009;4:44–57.
- Becatti E, Chkaiban L, Tonutti P, et al. Short-term postharvest carbon dioxide treatments induce selective molecular and metabolic changes in grape berries. J Agric Food Chem. 2010;58(13):8012–8020.
- Rosales R, Romero I, Fernandez-Caballero C, et al. Low temperature and short-term high-CO2 treatment in postharvest storage of table grapes at two maturity stages: effects on transcriptome profiling. Front Plant Sci. 2016;7:1020.
- Kolbe AR, Studer AJ, Cornejo OE, et al. Insights from transcriptome profiling on the non-photosynthetic and stomatal signaling response of maize carbonic anhydrase mutants to low CO2. BMC Genom. 2019;20(1):138.
- Chen T, Wu H, Wu J, et al. Absence of OsβCA1 causes a CO2 deficit and affects leaf photosynthesis and the stomatal response to CO2 in rice. Plant J. 2017;90(2):344–357.
- Pan C, Ahammed GJ, Li X, et al. Elevated CO2 improves photosynthesis under high temperature by attenuating the functional limitations to energy fluxes, electron transport and redox homeostasis in tomato leaves. Front Plant Sci. 2018;9:1739.
- Fiedor L, Kania A, Myśliwa-Kurdziel B, et al. Understanding chlorophylls: central magnesium ion and phytyl as structural determinants. Biochim Biophys Acta (BBA)-Bioenergetics. 2008;1777(12):1491–1500.
- Sirijovski N, Olsson U, Lundqvist J, et al. ATPase activity associated with the magnesium chelatase H-subunit of the chlorophyll biosynthetic pathway is an artefact. Biochem J. 2008;410(3):631–631.
- Blakeslee JJ, Spatola Rossi T, Kriechbaumer V. Auxin biosynthesis: spatial regulation and adaptation to stress. J Exp Bot. 2019;70(19):5041–5049.
- Silva GF, Silva EM, Correa JP, et al. Tomato floral induction and flower development are orchestrated by the interplay between gibberellin and two unrelated micro RNA‐controlled modules. New Phytol. 2019;221(3):1328–1344.
- Pan C, Zhang H, Ma Q, et al. Role of ethylene biosynthesis and signaling in elevated CO2-induced heat stress response in tomato. Planta 2019;250:563–572.
- Yujing L, Yannan Z, Bing C. Effects of hight atmospheric CO2 concentrations on activities of sucrose metabolism-related enzymes in lycium barbarum fruit. J Northwest For Univ. 2016;31:44–47.
- Moore B, Cheng SH, Sims D, et al. The biochemical and molecular basis for photosynthetic acclimation to elevated atmospheric CO2. Plant Cell Environ. 1999;22:567–582.
- Zhang Z, Liu L, Zhang M, et al. Effect of carbon dioxide enrichment on health-promoting compounds and organoleptic properties of tomato fruits grown in greenhouse. Food Chem. 2014;153:157–163.
- Dong J, Gruda N, Lam SK, et al. Effects of elevated CO2 on nutritional quality of vegetables – a review. Front Plant Sci. 2018;9:924.