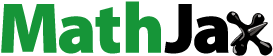
Abstract
The design and synthesis of biopolymer nano- and micro-formulations are a new trend with growing priority in scientific research and development in the fields of biomedicine, bioorganic/medicinal chemistry, pharmaceutics, agrochemistry and food industry. This incorporates a vast variety of improved and newly-developed analytical and physico-chemical techniques for green and efficient synthesis. The scope of the present review was to outline the recent advances in the methods for design of novel chitosan micro- and nano-carriers and transporters. Special emphasis is laid on their functionalities and capacity for encapsulation of natural bioactive compounds and controlled in vitro/in vivo release in various biological/physiological media. Expectations for the application of chitosan formulations and chitosan-based hybrid systems are progressively increasing as the knowledge regarding their physical, chemical and biological properties constantly expands. Thus, this review proposes insights on the objective assessment of the capacity, applicability and versatility of newly-designed chitosan-based hybrid systems. A detailed integrative approach, which incorporates the innovative scientific achievements based on complex novel, precise and reliable analytical procedures and methods for qualitative and quantitative morphological, structural, spectral, chemical and biochemical analyses of the bio-precursors and the designed chitosan-carrier micro/nano-hybrid systems, is applied. Sustainable knowledge on the mechanism and methods of natural bioactive substances encapsulation and in vitro/in vivo release is reviewed and discussed.
Introduction
Natural products have served as the source and inspiration for a large fraction of the current pharmacopoeia and continue to provide a diverse and unique source of bioactive compounds for drug discovery[Citation1–4].
Chitosan – a cationic natural polymer, first isolated in 1811, has begun its biomedical “rebirth” since the first decade of the twenty first century. Recent prominent scientific studies provide insights into its unique features and remarkable physical, chemical and biological properties such as biocompatibility, biodegradability, antimicrobial, antifungal, analgesic and antitumor activities. These features define its promising current and future applications in human and veterinary medicine, pharmaceutics, agro- and food industry [Citation5]. Being an excellent excipient, chitosan currently has enormous potential for the preparation of pharmaceutical dosage forms due to its polyelectrolyte properties, gel-forming capability and high adsorption capacity [Citation6]. Novel chitosan gels, suspensions, micro- and nano-particles [Citation7–12], spheres, capsules [Citation13–19] and drops have been recently investigated as potential controlled release carriers of synthetic drugs, proteins, enzymes and natural bioactive substances [Citation20].
Concerning these biologically active compounds, it should be emphasized that modern scientific research has been significantly focused on the exploration of various natural polyphenols, flavonoids, saponins, tannins, etc. [Citation21,Citation22], with diverse bioactivities including antioxidant, anti-inflammatory, anti-cancer, anti-microbial, anti-diabetic capabilities [Citation23], as well as on their applicability and efficiency for nutraceutical, therapeutic and pharmacological purposes. Scientific literature outlines that the basic challenges facing the practical application of these bioactive compounds arise from their high sensitivity to a diverse range of conditions and factors (temperature, oxygen, light, pH, oxidative enzymes, moisture content, etc.), associated with difficulties in the preservation of their stability and valuable biomedical properties. Thus, nano- and micro-encapsulation supports have emerged as reliable techniques to avoid the unwanted degradation of natural bioactive compounds [Citation24].
Another scientific area where chitosan formulations have been widely applied is the field of advanced cell technologies and therapeutics. Two-dimensional and three-dimensional chitosan scaffolds with various cell types cultured on them have been applied in cell-based regenerative therapy and tissue engineering. [Citation25,Citation26]. Increasing scientific knowledge and expectations for the unique potential for applicability of chitosan-based systems, however, face a number of constraints related to the lack of long-term stability, low solubility and sometimes unsatisfactory mechanical strength: challenges that have to be overcome and solved by modern science.
This review outlines the recent advances in the design methods of novel chitosan micro- and nano-carriers and transporters, with emphasis on their functionalities and capacity for encapsulation of natural bioactive compounds and controlled in vitro/in vivo release in various biological/physiological media.
Chitosan: molecular structure, nature and properties
Chitin and chitosan are linear polysaccharides consisting of β-(1→4) linked residues of N-acetyl-2-amino-2-deoxy-D-glucose (AcAG) and 2-amino-2-deoxy-D-glucose (AG). Due to the low content of 2-amino-2-deoxy-D-glucose units, chitin is insoluble in acidic aqueous media. The higher fraction of 2-amino-2-deoxy-D-glucose residues in chitosan determines the polymer solubility in acidic aqueous solutions [Citation25]. The deacetylation process of chitin to chitosan, which could be accomplished either by chemical, or enzymatic hydrolyses, generally involves removal of acetyl (CH3C = O-) groups resulting in the formation of amino (NH2-) groups, i.e. the formation of copolymers of N-acetylglucosamines and glucosamines () [Citation27–32].
Physico-chemical and structural analyses of chitosan nano-/micro-formulations
The majority of the literature reports on the physico-chemical properties and the structure of chitosan-based formulations employ various combinations of analytical methods, such as ultraviolet–visible (UV-VIS) spectrophotometry, fluorescence spectroscopy, Fourier-transform infrared spectroscopy (FTIR), nuclear magnetic resonance spectroscopy (NMR), conductometric and potentiometric titration, differential scanning calorimetry (DSC), average Mw and/or Mw distribution viscosimetry, light scattering, X-ray diffraction, thermogravimetric analysis (TGA), scanning electron microscopy (SEM), electron paramagnetic resonance (EPR), etc [Citation9,Citation10,Citation16,Citation30,Citation32–40]. Hussain et al. [Citation13] determined the degree of deacetylation (DDA) of alkali-modified essential oil-loaded chitosan by FTIR, potentiometric titration and elemental analysis. A direct relationship between the duration of the alkali treatment and DDA was established. SEM analyses indicated that DDA and crosslinking control the surface smoothness of the obtained microcapsules [Citation13]. The particle size, size distribution and zeta potential of biodegradable chitosan nanoformulations for encapsulation of strawberry extract polyphenols were measured by dynamic light scattering technique (DLS), and the morphology and size of the prepared nanoparticles were studied by SEM. UV-spectrophotometry and FTIR analyses were conducted to investigate the bonding between chitosan functional groups and polyphenol molecules [Citation41]. Harris et al. [Citation14] obtained chitosan hydrochloride nanoparticles and microspheres and proved that they could be adequate vehicles for the encapsulation of natural antioxidants due to maintainance of the antioxidant activity of Ilex paraguariensis extract polyphenols. The nanoparticles prepared by ionic gelation and the microspheres prepared by spray-drying, were characterized in terms of morphology, zeta potential analyses and in vitro release studies [Citation14]. The SEM image of a cross-sectional fracture of chitosan microbeads loaded with thyme (Thymus serpyllum L.) polyphenols showed the presence of polyphenol crystals [Citation42]. Porosity measurements confirmed that, upon encapsulation, polyphenols filled cavities of the matrix, therefore causing reduction in microbeads porosity [Citation42]. The complex structural analysis incorporating transmission electron microscope (TEM), atomic force microscopy (AFM), FTIR and thermogravimetric analysis (TGA)-differential scanning calorimetry (DSC) indicated that tea polyphenol-Zn complex was well incorporated into β-chitosan nanoparticles [Citation7]. The formation of epigallocatechin gallate-sulfobutyl ether-β-cyclodextrin sodium inclusion complexes with chitosan hydrochloride nanoparticles was confirmed by steady-state fluorescence, FTIR and NMR spectroscopy [Citation43]. The photomicrographs of chitosan microparticles synthesized by Cabral et al. [Citation8], by means of a spray-drying method, indicated that the microparticles were homogeneously spherical with a wrinkled surface, while the extent of surface smoothness increased directly with the increase of the quantity of the encapsulated jabutikaba peel extract polyphenols [Citation8]. Newly-synthesized water soluble quercetin loaded chitosan hydrochloride and carboxymethyl chitosan nanoparticles were optimized to small particle size, high zeta potential and high encapsulation efficiency [Citation44]. Their morphology was observed by SEM and quercetin encapsulation efficiency was confirmed based on molecular interactions indicated by FTIR and X-ray diffraction analyses [Citation44]. According to SEM analyses heat-denatured zein/carboxymethyl chitosan complex nanoparticles containing propolis appeared spherical and exhibited smooth surfaces; the carboxymethyl chitosan coating reduced the particle size – an observation that corresponded to DLS data [Citation45]. The comparative FTIR analyses confirmed that the complex nanoparticles were formed through hydrogen bonding, electrostatic and hydrophobic interactions [Citation45]. The real-time fabrication of dextran/chitosan multilayer of microcapsules for polyphenol co-delivery was monitored by quartz crystal microbalance with dissipation monitoring, and the morphology of the nanostructured polymeric capsules was characterized by SEM, whereas UV-spectroscopy and HPLC were applied for assessment of the encapsulation efficiency [Citation15]. The conjugation of resveratrol, genistein and curcumin with two types of chitosan was studied at physiological conditions, using fluorescence spectroscopy, FTIR, TEM and docking studies [Citation46]. Structural analysis indicated the presence of hydrophilic, hydrophobic and H-bonding between polyphenols and chitosan, which induced major alterations of chitosan morphology as established by the morphology studies [Citation46].
Chitosan characteristic parameters
The extent of deacetylation determines two basic and significant interdependent characteristics of chitosan, namely the degree of deacetylation (DD) and the degree of acetylation (DA), that govern important physicochemical, biochemical and biological properties of chitosan related to acid–base characteristics, electrostatic interactions, solubility, biodegradability, self-aggregation, sorption properties, chelation capacity, molecular conformation, etc., which are critical for the effectiveness of the biopolymer for various medical, pharmaceutical, biotechnological and agroindustrial applications [Citation13,Citation33]. DD and DA are measures of the fraction of AG or AcAG monomer residues, respectively, in percentile units [Citation33,Citation47]:
(1)
(1)
(2)
(2)
The DD exhibited by chitosan can be controlled during a relatively aggressive alkaline hydrolysis process applied to chitin, through a combination of exposure duration and temperature [Citation48,Citation49]. Foster et al. [Citation49] studied the variation in the physiochemical, mechanical and biological properties for a range of commercial chitosans within a narrow DD range (72–85%), with a view to chitosan potential in the regeneration of nerve tissue [Citation49]. The lowest chitosan DD values cited in literature are variable but usually within the range of 40–60%. However, the majority of commercial chitosan samples have average DD values (70–90%) [Citation13,Citation30]. The process of preparation of highly deacetylated chitosans (DD > 95%), which are appropriate for specific biological applications, often results in partial depolymerization of the polyssacharide and is associated with increased economic cost [Citation13,Citation50]. The solubility of chitosan is usually investigated in weakly acidic solutions of diluted inorganic (H3PO4, H2SO4) and organic (HCOOH, CH3COOH, C2H2(OH)2(COOH)2 tartaric acid, C2H4(OH)(COOH)3 citric acid) acids [Citation31,Citation50]. Due to the presence of primary –NH2 groups, chitosan is positively charged. The magnitude of the charge density, however, depends on the pH, DD and ionic strength. In acidic conditions, the –NH2 groups (pKa ∼ 6.5) are partially protonated (), which in turn provokes repulsion between positively charged polymer chains, thereby allowing diffusion of water molecules and subsequent solvation of the macromolecules [Citation30]. Sogias et al. [Citation51] showed that chemical disruption of chitosan crystallinity by partial re-acetylation or physical disruption caused by the addition of urea and guanidine hydrochloride broadens the pH-solubility range for this biopolymer [Citation51].
According to Tachaboonyakiat et al. [Citation52], the main cause of the water insolubility of phosphorylated chitosan is inter- and intra-molecular crosslinking. Fu and Xiao [Citation53]developed a facile physical approach to make chitosan soluble in acid-free water by modulating the solution concentration and varying the precipitants.
The capacity of chitosan for systemic bioabsorption, bioadsorption and diffusion is also dependent on its molecular weight (Mw). Scientific studies outlined that oligomers display greater affinity for sorption, whereas larger Mw chitosans were excreted without being sorbed [Citation48,Citation50]. Besides, the cytotoxic effects of these biopolymers were also dependent on the Mw [Citation54,Citation55]. According to the investigations of Chae et al. [Citation54], chitosan oligosaccharides (MW < 10 kDa) showed negligible cytotoxic effect on Caco-2 cells and were characterized with the highest plasma concentration. Due to the semicrystallinity of chitosan, it displays polymorphism which depends on its physical state [Citation48]. Recent scientific investigations [Citation13,Citation30,Citation33,Citation47–54] outlined strong and complex interdependence between various chitosan functionality parameters and intrinsic/extrinsic factors, which is schematically illustrated in .
Physico-chemical methods for chitosan modification
The abundant amine and hydroxyl groups present in chitosan monomers offer a unique opportunity for attaching targeting ligands or imaging agents. The scientific literature over the last 5 years abounds with studies on numerous newly-designed derivatives of chitosan tailored to improve the physicochemical properties of various chitosan formulations: size, shape, charge, density, solubility [Citation55], so as to enhance their biological activity in view of biocompatibility, antibacterial, antifungal, antioxidant activity, biodegradability, etc. Chitosan-based biodegradable materials, including micro- and nano-particles, gels, composites and films, have grown to become a key choice in agriculture due to their proven antimicrobial and plant-growth promoting activities [Citation56–59]. Recent research findings are subjected to the diverse biomedical and pharmaceutical applications of chitosan-based formulations for drug-delivery, gene delivery, cell encapsulation, tissue engineering, wound healing, bioimaging, antibacterial food packaging, etc. [Citation60,Citation61]. The variety of modification procedures [Citation28] and modifing agents applied in scientific investigations is highly specific depending on the intended future application of the chitosan composites () [Citation32,Citation52,Citation55,Citation62].
Table 1. Modified chitosan derivatives applied in various biomedical, agricultural and environmental fields (recent scientific literature review 2016-2019).
Diversity of chitosan formulations applied for encapsulation of natural bioactive compounds
Nano-/micro-particles, nano-/micro-spheres, microbeads, nano-/micro-capsules, hydrogels etc. are basic representatives of the variety of multiple unit chitosan-based formulations recently applied as potential drug carrier systems in the segment of novel drug delivery [Citation17].
The main disadvantages of chitosan-based matrices regarding the release of the incorporated bioactive substances are uncontrollable diffusion profile, passive drug release over time, poor control of external or internal stimuli [Citation77]. Efforts are directed towards overcoming these limitations and improving the physico-chemical, biochemical and biomedicinal properties of chitosan carriers by modification and optimization of existing methodologies and the development of innovative synthesis techniques. In this respect, a number of scientific studies during the last decade outlined that chitosan nano- and micro-formulations tested for different application routes, i.e. oral, ocular, nasal, vaginal, buccal, parenteral and intravesical, possess some unique characteristics over other delivery systems. These advantages include slow and controlled release of biologically active compounds, in situ gelling, mucoadhesion, hydrophilic behavior, enhanced transfection and permeation, improved drug encapsulation efficiency, bioavailability and controlled release of the target compound at specific active sites [Citation17,Citation77–80]. Mohapatra et al. [Citation77] developed a novel drug delivery platform based on biocompatible chitosan microbeads cross-linked with glyoxal and embedded with Fe3O4 magnetic nanoparticles, which was responsive to electric stimulation and exhibited statistically significant drug release efficiency . According to Aslan et al. [Citation55], the ionic cross-linking of chitosan is advantageous due to the facility of the method and the possibility for its performance under mild conditions without the use of organic solvents. Chitosan nanoparticles were synthesized by ionotropic gelation with tripolyphosphate, and their applicability for the incorporation of low molecular drugs, proteins, DNA/siRNA was examined. Novel chitosan-based polyelectrolyte nanocapsules were prepared and their improved encapsulation efficiency toward curcumin was established. The oral administration of these formulations induced significant decrease in hyperglycemia after 7 days [Citation81]. Encapsulation in chitosan nanoparticles improved the stability of tea polyphenols by preventing oxidation or degradation in the gastrointestinal tract was [Citation82]. The study of Wang et al. [Citation83] demonstrated the superior benefits of using cellulose nanocrystal as a macro-ion crosslinking agent over sodium tripolyphosphate, for the synthesis of chitosan microcapsules applied for improving the encapsulation efficiency and stability of blueberry anthocyanin extracts. Their results showed that CNC incorporation into chitosan functioned not only as a macro-ion crosslinking agent on the positively charged amino groups in chitosan, but also as a filler for the chitosan matrix, thus generating more rigid and stable microcapsules. The results revealed that the stable rigid complex microcapsules produced by the innovative crosslinking method were characterized with improved encapsulation efficiency and enhanced the stability of the encapsulated anthocyanins [Citation83]. The broad-spectrum imidazolinone herbicide imazaquin was encapsulated in starch and chitosan beads reinforced with alginate [Citation80]. The microformulations characterized with high porosity demonstrated improved matrix strength and prevented leakage of the encapsulated herbicide, which was due to interactions between the biopolymer functional groups and imazaquin molecules [Citation80]. A systematic classification of varous recently developed chitosan nano- and micro-formulations for the encapsulation of natural bioactive substances with emphasis on the preparation procedures is presented in .
Table 2. Multiplicity of chitosan nano- and micro-formulations applied for the encapsulation of natural biologically active compounds.
Methods and mechanism of bioactive compounds encapsulation
There are a number of techniques recently used for the encapsulation of natural and synthetic biologically active compounds on chitosan carriers, e.g. ionotropic gelation, emulsion phase separation, cross-linking with various agents, emulsion solvent diffusion, coacervation, etc. () [Citation55,Citation77,Citation82,Citation88,Citation89].
Figure 4. Generalized procedure for chitosan nano-/micro-particles synthesis and encapsulation. Adapted from [Citation18,Citation78,Citation88,Citation90–96].
![Figure 4. Generalized procedure for chitosan nano-/micro-particles synthesis and encapsulation. Adapted from [Citation18,Citation78,Citation88,Citation90–96].](/cms/asset/f10a840a-9826-4453-8efb-09755964ef4e/tbeq_a_1731333_f0004_c.jpg)
Other methods of chitosan micro- and nano-particles production are based on the coalescence phenomenon, collision of dispersed emulsion phases, formation of reversed micelles, opposite charged poly-ions complexation, etc. [Citation12,Citation14,Citation50] (Reviewed in [Citation77]). The design of new bioactive compound carriers together with the accurate mathematical modeling of the mass transport phenomena [Citation97] allows prediction of their behavior during shelf-life, product preparation or consumption, as well as controlling the release of the bioactive compounds [Citation91]. A systematic summary of the recently applied methods for encapsulation of natural bioactive compounds by various chitosan carriers, with emphasis on the analytical methodologies, the most significant functional characteristics of the microformulations and the mechanism of encapsulation/release, is presented in .
Table 3. Recent methods for bioactive compounds encapsulation on chitosan formulations.
In vitro/in vivo release mechanisms from chitosan nano-/micro-formulations
Drug release from biopolymer carriers is influenced by a number of factors including the composition and specific characteristics of the biologically active compound, the polymer, and additives, their ratio, physical and/or chemical interactions among the components, the synthesis and encapsulation methods. According to the mechanism by which a drug “escapes” a carrier, in vitro/in vivo drug release can be conditionally classified into five categories: diffusion-, degradation-, chemical bonds cleavage-, solvent- and stimuli-controlled release [Citation103]. The basic release mechanism principles and their advantageous characteristics described in recent scientific studies are summarized in .
Table 4. Mechanisms of release of natural bioactive compounds from chitosan formulations.
The main goals of the design of innovative chitosan-based formulations are achievement of two types of controlled bioactive substance release: temporal and distribution control. The temporal control ensures delivery over an extended or specific time period, whereas distribution control ensures targeted release of the bioalogically active compound to precise active sites [Citation110]. Besides, the search and need for a new generation of biopolymer hybrid systems has been provoked by the necessity of achieving active substance release subjected to desired kinetic model/s, eliminating the so-called undesirable “burst effect” and providing prolonged and controlled release [Citation78]. To accomplish these aims and to overcome the obstacles associated with bioactive compounds encapsulation and release, the basic initial stages required during the development of novel chitosan-based carriers are the investigations and assessment of the encapsulation efficiency, loading and release efficiency of the substance/carrier matrices. shows detailed comparative analyses of the values of these parameters for a number of new hybrid systems comprising of nano-/micro-sized chitosan formulations and natural bioactive substances.
Figure 5. Encapsulation and release efficiency of chitosan micro/nano-formulations loaded with natural biologically active compounds. Graphically presented data adapted from [Citation7–10,Citation14,Citation15,Citation34,Citation39,Citation41,Citation42,Citation44–46,Citation84,Citation85,Citation89,Citation99,Citation100,Citation111–118].
![Figure 5. Encapsulation and release efficiency of chitosan micro/nano-formulations loaded with natural biologically active compounds. Graphically presented data adapted from [Citation7–10,Citation14,Citation15,Citation34,Citation39,Citation41,Citation42,Citation44–46,Citation84,Citation85,Citation89,Citation99,Citation100,Citation111–118].](/cms/asset/79aae287-e939-437e-87e8-bb20ad640038/tbeq_a_1731333_f0005_c.jpg)
International/European regulatory aspects and future perspectives
Although recent studies indicated that a number of newly-developed chitosan formulations meet the criteria for good biomaterials characterized with unique antibacterial activity, non-allergic response, oxygen permeability, biodegradation to relatively neutral oligomers, unique ability to accelerate healing, functional flexibility, etc. [Citation118], chitosan-based carriers have not been approved yet by the American Food and Drug Association (FDA) in the field of medical applications [Citation48]. In this respect, The American Society of Testing Materials (ASTM F04 division IV) is making efforts to establish standard guidelines for chitosan salts suitable for use in biomedical or pharmaceutical applications, or both, including, but not limited to, tissue-engineered medical products (TEMPS). This guide addresses key parameters relevant for the functionality, characterization and purity of chitosan salts [Citation48,Citation119]. A derivative of chitosan, chitosan hydrochloride, was included in the European Pharmacopoeia (EP) in 2002. The corresponding EP monograph includes tests for heavy metal contamination but does not address bioburden, sterility and bacterial endotoxins (Reviewed in [Citation120]). Taking into account that purity, which is quantified as the remaining ashes, proteins, insolubles and also the bio-burden (microbes, yeasts and moulds, endotoxins, etc.), is vital particularly for high value products, a more detailed characterization is needed [Citation48]. Currently, chitosan has been approved for wound dressing applications and cartilage repairing formulation. However, chitosan approval by the FDA is not a general definition as GRAS (generally recognized as safe), but FDA and other regulatory agencies evaluate and approve materials with respect to their specific applications [Citation27]. Chitosan has been approved as functional food in some Asian countries [Citation48], recognized as safe (GRAS) and approved for dietary use in Italy and Finland. In 2008 a specific monograph was introduced in the European Pharmacopeia and in 2011 in the US National formulary [Citation27]. Based on these facts and regulatory aspects, it could be concluded that the application of chitosan-based carriers for the development of drug formulations is still limited [Citation27]. Thus, the innovative and future studies subjected to the biopolymers biomedical and pharmaceutical applicability have to include detailed and precise clinical investigations assessing probable health risks, side effects, biocompatibility and health benefits associated with the application of chitosan formulations.
With approximately 1011 tons of chitin produced per year from waste crustacean carapaces, chitosan is a cost effective biomaterial [Citation49,Citation50]. Despite the fact that chitosan-based formulations sustain immense biological activities in plants, these materials have not yet been widely adopted as sustainable agricultural remedies due to the lack of reliable knowledge and research on their bioactivity, modes of action towards pathogenic microorganism, plant protection and growth [Citation59].
Conclusions
To satisfy the anticipated claims of chitosan-based formulations and their applicability in the field of bioorganic chemistry, encompassing medical (human and veterinary), pharmaceutical and agricultural scientific research, it is imperative to line up all the possible advantages and strengths, as well as to overcome the established and expected disadvantages and weaknesses associated with the scope of achieving sustainable knowledge, research and development.
Authors’ contributions
Z.Y. developed the review conception and design, performed the acquisition, critical analysis and interpretation of data, prepared the manuscript and revised it critically for important intellectual content; D.I., M.T. and N.N. contributed specific information to the manuscript. All authors read and approved the final manuscript.
Disclosure statement
No potential conflict of interest was reported by the authors.
Additional information
Funding
References
- Serce S, Ercisli S, Sengul M, et al. Antioxidant activities and fatty acid composition of wild grown myrtle (Myrtus communis L.) fruits. Phcog Mag. 2010;6(21):9–12.
- Ozdemir F, Nadeem HS, Akdogan A, et al. Effect of altitude, shooting period, and tea grade on the catechins, caffeine, theaflavin, and thearubigin of Turkish black tea. Turk J Agric For. 2018;42:334–340.
- Senkal BC, Uskutoglu T, Cesur C, et al. Determination of essential oil components, mineral matter, and heavy metal content of Salvia virgata Jacq. grown in culture conditions. Turk J Agric For. 2019;43:395–404.
- Kingston DG. Modern natural products drug discovery and its relevance to biodiversity conservation. J Nat Prod. 2011;74(3):496–511.
- Chien RC, Yen MT, Mau JL. Antimicrobial and antitumor activities of chitosan from shiitake stipes, compared to commercial chitosan from crab shells. Carbohydr Polym. 2016;138:259–264.
- Domínguez-Delgado CL, Fuentes-Prado E, Escobar Chávez JJ, et al. Chitosan and Pluronic® F-127: pharmaceutical applications. In: Mishra M, editor. Encyclopedia of biomedical polymers and polymeric biomaterials. New York (NY): Taylor and Francis; 2016. p. 1513–1535.
- Zhang H, Zhao Y. Preparation, characterization and evaluation of tea polyphenole Zn complex loaded β-chitosan nanoparticles. Food Hydrocolloids. 2015;48:260–273.
- Cabral BRP, de Oliveira PM, Gelfuso GM, et al. Improving stability of antioxidant compounds from Plinia cauliflora (jabuticaba) fruit peel extract by encapsulation in chitosan microparticles. J. Food Eng. 2018;238:195–201.
- Baspinar Y, Üstündas M, Bayraktar O, et al. Curcumin and piperine loaded zein-chitosan nanoparticles: Development and in-vitro characterization. Saudi Pharm J. 2018;26(3):323–334.
- Hosseini SF, Zandi M, Rezaei M, et al. Two-step method for encapsulation of oregano essential oil in chitosan nanoparticles: preparation, characterization and in vitro release study. Carbohydr Polym. 2013;95(1):50–56.
- Carlan IC, Estevinho BN, Rocha F. Study of microencapsulation and controlled release of modified chitosan microparticles containing vitamin B12. Powder Technol. 2017;318:162–169.
- Martin-Saldaña S, Chevalier MT, Iglesias MJ, et al. Salicylic acid loaded chitosan microparticles applied to lettuce seedlings: Recycling shrimp fishing industry waste. Carbohyd Polym. 2018;200:321–331.
- Hussain MR, Iman M, Maji TK. Determination of degree of deacetylation of chitosan and their effect on the release behavior of essential oil from chitosan and chitosan-gelatin complex microcapsules. Int J of Adv Eng Appl. 2013;2(4):4–12.
- Harris R, Lecumberri E, Mateos-Aparicio I, et al. Chitosan nanoparticles and microspheres for the encapsulation of natural antioxidants extracted from Ilex paraguariensis. Carbohydr Polym. 2011;84(2):803–806.
- Paini M, Aliakbarian B, Casazza AA, et al. Chitosan/dextran multilayer microcapsules for polyphenol co-delivery. Materials Sci. Eng. C.2015;46:374–380.
- Saranya TS, Rajan VK, Biswas R, et al. Synthesis, characterisation and biomedical applications of curcumin conjugated chitosan microspheres. Int J Biol Macromol. 2018;110:227–233.
- Patel KS, Patel MB. Preparation and evaluation of chitosan microspheres containing nicorandil. Int J Pharma Investig. 2014;4(1):32–37.
- Sharma M, Pk C, Kumar Dev S. Formulation and in-vitro/in-vivo evaluation of alginate-chitosan microspheres of glipizide by ionic gelation method. Asian J Pharm Clin Res. 2017;10(7):385–390.
- Cui X, Guan X, Zhong S, et al. Multi-stimuli responsive smart chitosan-based microcapsules for targeted drug delivery and triggered drug release. Ultrason Sonochem. 2017;38:145–153.
- Chatterjee S, Tran HN, Godfred OB, et al. Supersorption capacity of anionic dye by newer chitosan hydrogel capsules via green surfactant exchange method. ACS Sustainable Chem Eng. 2018;6(3):3604–3614.
- Simeonov E, Yaneva Z, Chilev C. Kinetics of green solid-liquid extraction of useful compounds from plant materials - kinetics coefficients and modelling. Green Process Synth. 2018;7(1):68–73.
- Yaneva Z, Georgieva NV, Bekirska LL, Lavrova S. Drug mass transfer mechanism, thermodynamics, and in vitro release kinetics of antioxidant-encapsulated zeolite microparticles as a drug carrier system. CABEQ. 2018;32(3):281–298.
- Simeonov E, Yaneva Z, Chilev C. Investigation of the mechanism and kinetics of extraction from plant materials. Bulgarian Chem Commun. 2017;49(2):399–409.
- Hussain SA, Hameed A, Nazir Y, et al. Microencapsulation and the characterization of polyherbal formulation (PHF) rich in natural polyphenolic compounds. Nutrients. 2018;10(7):843–867.
- Intini C, Elviri L, Cabral J, et al. 3D-printed chitosan-based scaffolds: an in vitro study of human skin cell growth and an in-vivo wound healing evaluation in experimental diabetes in rats. Carbohydr Polym. 2018;199:593–602.
- Rodríguez-Vázquez M, Vega-Ruiz B, Ramos-Zúñiga R, et al. Chitosan and its potential use as a scaffold for tissue engineering in regenerative medicine. Biomed Res Int. 2015;2015:1–15.
- Bellich B, D’Agostino I, Semeraro S, et al. “The Good, the Bad and the Ugly” of chitosans. Mar Drugs. 2016;14(99):1–31.
- Mittala H, Raya SS, Kaith BS, et al. Recent progress in the structural modification of chitosan for applications in diversified biomedical fields. Eur Polym J. 2018;109:402–434.
- de Moura CM, de Moura JM, Soares NM, et al. Evaluation of molar weight and deacetylation degree of chitosan during chitin deacetylation reaction: Used to produce biofilm. Chem Eng Process. 2011;50(4):351–355.
- Rangel-Vazquez NA, Felix FR. Computational chemistry applied in the analyses of chitosan/polyvinylpyrrolidone/mimosa tenuiflora. 1st ed. New York (NY): Science Publishing Group; 2014. ISBN: 978-1-940366-00-5.
- Akakuru OU, Louis H, Amos PI, et al. The chemistry of chitin and chitosan justifying their nanomedical utilities. Biochem Pharmacol (Los Angel). 2018;7:1–6.
- Sharif R, Mujtaba M, Rahman MU, et al. The multifunctional role of chitosan in horticultural crops. Molecules. 2018;23(4):872–892.
- Cunha RA, Soares TA, Rusu VH, et al. The Molecular Structure and Conformational Dynamics of Chitosan Polymers: An Integrated Perspective from Experiments and Computational Simulations. In: Karunaratne DN, editor. The complex world of polysaccharides. London, UK: InTech; 2012. Chapter 9.
- Yang R, Liu Y, Gao Y, et al. Nano-encapsulation of epigallocatechin gallate in the ferritin-chitosan double shells: Simulated digestion and absorption evaluation. Food Res Int. 2018;108:1–7.
- Liˇsková J, Douglas TEL, Beranová J, et al. Chitosan hydrogels enriched with polyphenols: antibacterial activity, cell adhesion and growth and mineralization. Carbohydr Polym. 2015;129:135–142.
- Casanova F, Estevinho BN, Santos L. Preliminary studies of rosmarinic acid microencapsulation with chitosan and modified chitosan for topical delivery. Powder Technol. 2016;297:44–49.
- Ye J, Wang S, Lan W, et al. Preparation and properties of polylactic acid-tea polyphenol-chitosan composite membranes. Int J Biol Macromol. 2018;117:632–639.
- Liu Y, Wang S, Lan W, et al. Development of ultrasound treated polyvinyl alcohol/tea polyphenol composite films and their physicochemical properties. Ultrason Sonochem. 2019;51:386–394.
- Kumaraswamy RV, Kumari S, Choudhary RC, et al. Salicylic acid functionalized chitosan nanoparticle: a sustainable biostimulant for plant. Int J Biol Macromol. 2019;123:59–69.
- Karamalakova Y, Nikolova G, Adhikari M, et al. Oxidative-protective effects of Tinospora cordifolia extract on plasma and spleen cells after experimental ochratoxicosis. Comp Clin Pathol. 2018;27(6):1487–1495.
- Pulicharla R, Marques C, Das RK, et al. Encapsulation and release studies of strawberry polyphenols in biodegradable chitosan nanoformulation. Int J Biol Macromol. 2016;88:171–178.
- Trifković KT, Milašinović NZ, Djordjević VB, et al. Chitosan microbeads for encapsulation of thyme (Thymus serpyllum L.) polyphenols. Carbohydr Polym. 2014;111:901–907.
- Liu F, Antoniou J, Li Y, et al. Chitosan/sulfobutylether-b-cyclodextrin nanoparticles as a potential approach for tea polyphenol encapsulation. Food Hydrocolloids. 2016;57:291–300.
- Yan L, Wang R, Wang H, et al. Formulation and characterization of chitosan hydrochloride and carboxymethyl chitosan encapsulated quercetin nanoparticles for controlled applications in foods system and simulated gastrointestinal condition. Food Hydrocolloids. 2018;84:450–457.
- Zhang H, Fu Y, Niu F, et al. Enhanced antioxidant activity and in vitro release of propolis by acid induced aggregation using heat-denatured zein and carboxymethyl chitosan. Food Hydrocolloids. 2018;81:104–112.
- Chanphai P, Tajmir-Riahi HA. Probing the binding of resveratrol, genistein and curcumin with chitosan nanoparticles. J Mol Liq. 2017;243:108–114.
- Czechowska-Biskup R, Jarosińska D, Rokita B, et al. Determination of degree of deacetylation of chitosan – comparison of methods. Prog Chem Appl Chitin Deriv. 2012;17:5–20.
- Aranaz I, Mengíbar M, Harris R, et al. Functional characterization of chitin and chitosan. Current Chem Biol. 2009;3:203–230.
- Foster LJR, Ho S, Hook J, et al. Chitosan as a biomaterial: influence of degree of deacetylation on its physiochemical, material and biological properties. PLoS One. 2015;10(8):e0135153.
- Domínguez-Delgado CL, Rodríguez-Cruz IM, Fuentes-Prado E, et al. Drug carrier systems using chitosan for non parenteral routes. In: Gowder SJT, editor. Pharmacology and therapeutics. London, UK: InTech; 2014. Chapter 10.
- Sogias IA, Khutoryanskiy VV, Williams AC. Exploring the factors affecting the solubility of chitosan in water. Macromol Chem Phys. 2010;211(4):426–433.
- Tachaboonyakiat W, Netswasdi N, Srakaew V, et al. Elimination of inter- and intramolecular crosslinks of phosphorylated chitosan by sodium salt formation. Polym J. 2010;42(2):148–156.
- Fu Y, Xiao C. A facile physical approach to make chitosan soluble in acid-free water. Int J Biol Macromol. 2017;103:575–580.
- Chae SY, Jang MK, Nah JW. Influence of molecular weight on oral absorption of water soluble chitosans. J Control Release. 2005;102(2):383–394.
- Aslan B, Han HD, Lopez-Berestein G, et al. Chitosan nanoparticles. In: Bhushan B, editor. Encyclopedia of nanotechnology. Dordrecht: Springer; 2016.
- Shishir MRI, Xie L, Sun C, et al. Advances in micro and nano-encapsulation of bioactive compounds using biopolymer and lipid-based transporters. Trends Food Sci Technol. 2018;78:34–60.
- Perez JJ, Francois NJ, Maroniche GA, et al. A novel, green, low-cost chitosan-starch hydrogel as potential delivery system for plant growth-promoting bacteria. Carbohydr Polym. 2018;202:409–417.
- Chang CT, Lin YL, Lu SW, et al. Characterization of a chitosanase from jelly fig (Ficus awkeotsang Makino) latex and its application in the production of water-soluble low molecular weight chitosans. PLoS One. 2016;11(3):e0150490. doi: 10.1371/journal.pone.0150490
- Kumaraswamy RV, Kumari S, Choudhary RC, et al. Engineered chitosan based nanomaterials: bioactivities, mechanisms and perspectives in plant protection and growth. Int J Biol Macromol. 2018;113:494–506.
- Shariatinia Z. Pharmaceutical applications of chitosan. Adv Colloid Interface Sci. 2019;263:131–194.
- Pakdel PM, Peighambardoust SJ. Review on recent progress in chitosan-based hydrogels for wastewater treatment application. Carbohydr Polym. 2018;201:264–279.
- de Araújo Braz EM, Costa de Silva SCC, da Silva DA, et al. Modified chitosan-based bioactive material for antimicrobial application: synthesis and characterization. Int J Biol Macromol. 2018;117(1):640–647.
- Saekhor K, Udomsinprasert W, Honsawek S, et al. Preparation of an injectable modified chitosan-based hydrogel approaching for bone tissue engineering. Int J Biol Macromol. 2019;123:167–173.
- Olad A, Bakht H, Hagh K. Graphene oxide and amin-modified graphene oxide incorporated chitosan gelatin scaffolds as promising materials for tissue engineering. Composites Part B. 2019;162:692–702.
- Jaiswal S, Dutta PK, Kumar S, et al. Methyl methacrylate modified chitosan: synthesis, characterization and application in drug and gene delivery. Carbohydr Polym. 2019;211:109–117.
- Cheah WY, Show P, Ng I, et al. Antibacterial activity of quaternized chitosan modified nanofiber membrane. Int J Biol Macromol. 2019;126:569–577.
- Tripathi N, Choppala G, Singh RS. Evaluation of modified chitosan for remediation of zinc contaminated soils. J Geochem Explor. 2017;182:180–184.
- Shankar A, Kongot M, Saini VK, et al. Removal of pentachlorophenol pesticide from aqueous solutions using modified chitosan. Arabian J Chem. 2018;13:1821–1830.
- Kumar S, Deepak V, Kumari M, et al. Antibacterial activity of diisocyanate-modified chitosan for biomedical applications. Int J Biol Macromol. 2016;84:349–353.
- Saba MW, Elzanaty AM, Abdel-Gawad OF, et al. Synthesis, characterization and antimicrobial activity of Schiff bases modified chitosan-graft-poly(acrylonitrile). Int J Biol Macromol. 2018;109:1280–1291.
- Tyliszczak B, Drabczyk A, Kudłacik-Kramarczyk S, et al. Preparation and cytotoxicity of chitosan-based hydrogels modified with silver nanoparticles. Colloids Surf B Biointerfaces. 2017;160:325–330.
- Elsayed NH, Monier M, Youssef I. Fabrication of photo-active trans-3-(4-pyridyl)acrylic acid modified chitosan. Carbohydr Polym. 2017;172:1–10.
- Fang Z, Lin D, Warner RD, et al. Effect of gallic acid/chitosan coating on fresh pork quality in modified atmosphere packaging. Food Chem. 2018;260:90–96.
- Kumar D, Pandey J, Kumar P. Synthesis and characterization of modified chitosan via microwave route for novel antibacterial application. Int J Biol Macromol. 2018;107:1388–1394.
- Fan Z, Qin Y, Liu S, et al. Synthesis, characterization, and antifungal evaluation of diethoxyphosphoryl polyaminoethyl chitosan derivatives. Carbohydr Polym. 2018;190:1–11.
- Liang W, Yu A, Wang G, et al. A novel water-based chitosan-La pesticide nanocarrier enhancing defense responses in rice (Oryza sativa L) growth. Carbohydr Polym. 2018;199:437–444.
- Mohapatra A, McGraw G, Morshed BI, et al. Electric stimulus response of chitosan microbeads embedded with magnetic nanoparticles for controlled drug delivery. 2014 IEEE Healthcare Innovation Conference (HIC), Seattle, WA.
- Balcerzak J, Mucha M. Method for preparation of hybrid spherical chitosan/poly(lactic acid) structures. Prog Chem Appl Chitin Deriv. 2008;13:57–64.
- Mohapatra A, Harris MA, LeVine D, et al. Magnetic stimulus responsive vancomycin drug delivery system based on chitosan microbeads embedded with magnetic nanoparticles. J Biomed Mater Res. 2018;106(6):2169–2176.
- Nnamonu LA, Sha’Ato R, Onyido I. Alginate reinforced chitosan and starch beads in slow release formulation of imazaquin herbicide - preparation and pharacterization. MSA. 2012;3:566–574.
- Akolade JO, Oyelola H, Oloyede B. Encapsulation in chitosan-based polyelectrolyte complexes enhances antidiabetic activity of curcumin. J Funct Foods. 2017;35:584–594.
- Liang J, Yan H, Puligundla P, et al. Applications of chitosan nanoparticles to enhance absorption and bioavailability of tea polyphenols: a review. Food Hydrocoll. 2017;69:286–292.
- Wang W, Jung J, Zhao Y. Chitosan-cellulose nanocrystal microencapsulation to improve encapsulation efficiency and stability of entrapped fruit anthocyanins. Carbohydr Polym. 2017;157:1246–1253.
- Belščak-Cvitanović A, Stojanović R, Manojlović V, et al. Encapsulation of polyphenolic antioxidants from medicinal plant extracts in alginate–chitosan system enhanced with ascorbic acid by electrostatic extrusion. Food Res Int. 2011;44(4):1094–1101.
- Udeni Gunathilake TMS, Ching YC, Chuah CH, et al. Influence of a nonionic surfactant on curcumin delivery of nanocellulose reinforced chitosan hydrogel. Int J Biol Macromol. 2018;118:1055–1064.
- Mohapatra А, Sahadat N, Morshed BI, et al. Stimuli-controlled drug delivery system development with implantable biocompatible chitosan microbeads. Proceedings of the 2014 IAJC-ISAM International Conference, Orlando, FL; 2014
- Dias AMA, Cortez AR, Barsan MM, et al. Development of greener multi-responsive chitosan biomaterials doped with biocompatible ammonium ionic liquids. ACS Sustainable Chem Eng. 2013;1(11):1480–1492.
- Moreno MA, Gómez-Mascaraque LG, Arias M, et al. Electrosprayed chitosan microcapsules as delivery vehicles for vaginal phytoformulations. Carbohydr Polym. 2018;201:425–437.
- Rojas J, Ciro Y, Zapata S. Chitosan as a potential microencapsulation carrier for ascorbic acid stabilization in heterodisperse systems. Int J Pharm Pharm Sci. 2015;7(1):69–72.
- Rubilar JF, Cruz RMS, Zuñiga RN, et al. Mathematical modeling of gallic acid release from chitosan films with grape seed extract and carvacrol. Int J Biol Macromol. 2017;104:197–203.
- Abdelkader H, Hussain SA, Abdullah N, et al. Review on micro-encapsulation with chitosan for pharmaceuticals applications. MOJCRR. 2018;1(2):77–84.
- Wang L-Y, Gu Y-H, Zhou Q-Z, et al. Preparation and characterization of uniform-sized chitosan microspheres containing insulin by membrane emulsification and a two-step solidification process. Colloids Surf B Biointerfaces. 2006;50(2):126–135.,
- Manjann KM, Shivakumar B, Pramod Kumar TM. Microencapsulation: An аcclaimed novel drug-delivery system for NSAIDs in arthritis. Crit Rev Ther Drug Carrier Syst. 2010;27(6):501–532.
- Ibrahim HM, Ali EHA, Sabry HA. Haloperidol-loaded chitosan nanocomposites improve liver and kidney functions and lipid profile of male rats. Res J Pharm Biol Chem Sci. 2017;8(3):1135–1145.
- Sacco P, Paoletti S, Cok M, et al. Insight into the ionotropic gelation of chitosan using tripolyphosphate and pyrophosphate as cross-linkers. Int J Biol Macromol. 2016;92:476–483.
- Ahmed TA, Aljaeid BM. Preparation, characterization, and potential application of chitosan, chitosan derivatives, and chitosan metal nanoparticles in pharmaceutical drug delivery. Drug Des Devel Ther. 2016;10:483–507.
- Yaneva Z, Georgieva N. Study on the physical chemistry, equilibrium, and kinetic mechanism of azure a biosorption by zea mays biomass. J Disp Sci Technol. 2014;35(2):193–204.
- Agarwal S, Rajoria P, Rani A. Adsorption of tannic acid from aqueous solution onto chitosan/NaOH/fly ash composites: equilibrium, kinetics, thermodynamics and modeling. J Environ Chem Eng. 2018;6(1):1486–1499.
- Shetta A, Kegere J, Mamdouh W. Comparative study of encapsulated peppermint and green tea essential oils in chitosan nanoparticles: Encapsulation, thermal stability, in-vitro release, antioxidant and antibacterial activities. Int J Biol Macromol. 2019;126:731–742.
- Tagaç AA, Sarp Ö, Yurdakoç K. Controlled release of Vitamin C from chitosan nanoparticles. HJBC. 2018;46(1):69–77.
- Liang J, Yan H, Yang H, et al. Synthesis and controlled-release properties of chitosan/β-Lactoglobulin nanoparticles as carriers for oral administration of epigallocatechin gallate. Food Sci Biotechnol. 2016;25(6):1583–1590.
- Vanden Braber NL, Paredes AJ, Rossi YE, et al. Controlled release and antioxidant activity of chitosan or its glucosamine water-soluble derivative microcapsules loaded with quercetin. Int J Biol Macromol 2018;112:399–404.
- Lee JH, Yeo Y. Controlled drug release from pharmaceutical nanocarriers. Chem Eng Sci. 2015;125:75–84.
- Kamaly N, Yameen B, Wu J, et al. Degradable controlled-release polymers and polymeric nanoparticles: Mechanisms of controlling drug release. Chem Rev. 2016;116(4):2602–2663.
- Son G, Lee B, Cho C. Mechanisms of drug release from advanced drug formulations such as polymeric-based drug-delivery systems and lipid nanoparticles. J Pharm Investig. 2017;47(4):287–296.
- Uhrich KE, Cannizzaro SM, Langer RS, et al. Polymeric systems for controlled drug release. Chem Rev. 1999;99(11):3181–3198.
- Marin E, Briceno MI, Caballero-George C. Critical evaluation of biodegradable polymers used in nanodrugs. Int J Nanomed. 2013;8:3071–3090.
- Park H, Park K, Shalaby W. Biodegradable hydrogels for drug delivery. 1st ed. Florida, USA: CRC Press; 1993.
- Lucio D, Martínez-Ohárriz MC. Chitosan: strategies to increase and modulate drug release rate. In: Shalaby E, editor. Biological activities and application of marine polysaccharides. London, UK: InTech;2017. Chapter 6.
- Ruiz-Caro R, Veiga-Ochoa MD. Characterization and dissolution study of chitosan freeze-dried systems for drug controlled release. Molecules. 2009;14(11):4370–4386.
- Nimesh S. Gene therapy: potential applications of nanotechnology, 2. Methods of nanoparticle preparation. 1st ed. Cambridge, UK: Woodhead Publishing Series in Biomedicine; 2013. p. 13–42.
- Hasheminejad N, Khodaiyan F, Safari M. Improving the antifungal activity of clove essential oil encapsulated by chitosan nanoparticles. Food Chem. 2019;275:113–122.
- Ren X, Hou T, Liang Q, et al. Effects of frequency ultrasound on the properties of zein-chitosan complex coacervation for resveratrol encapsulation. Food Chem. 2019;279:223–230.
- Mania S, Tylingo R, Michałowska A. The drop-in-drop encapsulation in chitosan and sodium alginate as a method of prolonging the quality of linseed oil. Polymers. 2018;10(12):1355–1371.
- Jamil B, Abbasi R, Abbasi S, et al. Encapsulation of cardamom essential oil in chitosan nano-composites: In-vitro efficacy on antibiotic-resistant bacterial pathogens and cytotoxicity studies. Front Microbiol. 2016;7(1580):1–10.
- Janssens B. Encapsulation of vitamin D3 and vitamin K2 in chitosan coated liposomes [MSc thesis]. Ghent, Belgium: Faculty of Pharmaceutical Sciences, Universita Degli Studi Di Salerno, Ghent University, 2018. 1–42.
- Hadiyanto MS, Majid D, Setyawan D, et al. Improvement of stability and antioxidant activities by using phycocyanin - chitosan encapsulation technique. IOP Conf Ser, Earth Environ Sci. 2017;55:1–7.
- Drewnowska O, Turek B, Carstanjen B, et al. Chitosan – a promising biomaterial in veterinary medicine. Pol J Vet Sci. 2013;16(4):843–848.
- ASTM F2103-01, Standard guide for characterization and testing of chitosan salts as starting materials intended for use in biomedical and tissue-engineered medical product applications. West Conshohocken, PA: ASTM International; 2001.
- Dornish M, Kaplan DS, Arepalli SR. Regulatory status of chitosan and derivatives. In: Sarmento B, Neves JD, editors. Chitosan based systems for biopharmaceuticals. Delivery, targeting and polymer therapeutics. Chichester (UK): Wiley; 2012. Chapter 24.