Abstract
The aim of the present study was to determine the content of residual monomer methyl methacrylate (MMA) in cold and hot polymerized poly(methyl methacrylate) (PMMA), both widely applicable in dentistry, by using MHE-GC-MS (multiple headspace extraction analysis by gas chromatography-mass spectrometry) in combination with GC-FID (gas chromatography with flame-ionization detection). The samples of PMMA were obtained by free-radical polymerization of MMA at room temperature (cold polymerized PMMA) and at 100 °C (hot polymerized PMMA), according to the manufacturer’s instructions. The MHE-GC-MS method consisted of ten successive extractions of MMA from the polymerized samples. According to the constructed calibration curve, the content of residual MMA in PMMA samples (mgMMA/gPMMA) was determined. Intramuscular implantation of sample materials was performed in experimental animals (rats) in order to demonstrate potential adverse effects of the unpolymerized residual reactants to the tissue in direct contact with polymeric implants. The content of residual monomer in cold polymerized PMMA was higher (15.75 mgMMA/gPMMA) in comparison to hot polymerized PMMA (10.96 mgMMA/gPMMA). The implanted samples of cold-polymerized acrylic resin showed intense inflammatory response of the surrounding tissue. According to the obtained results, hot polymerized PMMA is safer for use in dentistry.
Introduction
Poly(methyl methacrylate) (PMMA) represents an important polymeric material that is widely used in paper, paint and car industry, but also in medicine (bone cement, contact lenses, dialysis membranes) and dentistry [Citation1]. PMMA is used in dentistry for manufacturing and readaptation of dentures, as well as manufacturing of occlusal splints and orthodontic appliances. Dental acrylic resins are clinically acceptable due to their natural appearance, durability, satisfying thermal characteristics, low absorption and insolubility in oral fluids, as well as the lack of taste and smell of the material [Citation2,Citation3]. Acrylic dentures can be manufactured by cold and hot polymerization of MMA.
On the other hand, it is well known that residual compounds that remain in the polymer after polymerization can diffuse from dental extensions into saliva and cause local allergic and toxic effects such as cheilitis, stomatitis, tingling and numbness in the mouth, painful sensations of different intensity, oral candidiasis [Citation4–8]. Allergic reactions to acrylic appliances can be additionally manifested as a severe clinical form, called erythema multiforme [Citation9]. Contact stomatitis in children caused by using orthodontic appliances is also described in clinical practice [Citation10]. In addition, the mechanical properties of polymers become poorer with increasing concentrations of residual reactants [Citation11,Citation12]. The most abundant residual compounds in the polymerized acrylates are: methyl methacrylate (MMA), hydroquinone, benzoyl peroxide, ethylene glycol dimethacrylate, benzoic acid, methyl benzoate and formaldehyde [Citation13–16]. Cold polymerizing acrylates have non-homogeneous and porous structure, and hence the higher percentage of potentially toxic substances [Citation17–19] (For review, see [Citation20]).
Although the concentration of the residual compounds in the polymer decreases with time, PMMA always contains a small amount of unreacted MMA due to the thermodynamic equilibrium between these two compounds. Therefore, the determination of residual monomers in the polymer represents one of the fundamental analyses in production and quality control of dental acrylic resins in order to obtain clinically acceptable and biocompatible dental appliances. For the determination of residual monomer content, gas chromatography with flame-ionization detector (GC-FID) as a sensitive and robust method is most commonly used. In addition to this method, gas chromatography-mass spectrometry (GC-MS), nuclear magnetic resonance (NMR), fourier transform infra-red spectroscopy (FTIR) and high performance liquid chromatography (HPLC) can be applied [Citation21,Citation22].
Multiple headspace extraction (MHE) in combination with GC-MS and GC-FID methods (MHE-GC-MS method) represent an alternative to frequently applied methods for polymer samples analysis. Previous sample preparation, such as residual monomer extraction using adequate solvent, is not required. The method includes multiple successive extractions of the residual reactant from the solid and/or complex polymeric matrix, under the same experimental conditions [Citation22]. A standard (reference) substance is used for calibration curve preparation and is analyzed under identical experimental conditions as the sample. Based on the obtained results and calibration curve, the total mass of residual reactant in the sample can be determined as a function of the peak area in the chromatogram.
The aim of this work was to determine the amount of residual MMA monomer in hot and cold polymerized acrylic resins for use in dentistry by using MHE-GC-MS in combination with GC-FID. Histopathology analysis of the implants surrounding muscle tissue of experimental rats was performed in order to prove the inflammatory effect of the dental resins unpolymerized components.
Materials and methods
Acrylic resin specimen preparation
The materials used in the study and applied polymerization methods are presented in .
Table 1. Applied materials and polymerization methods.
To determine the amount of residual monomer, disk-shaped specimens of each material were produced by using a circular stainless steel mould of 10 mm in diameter and 2 mm in depth and prepared following the manufacturer’s directions. Acrylate samples were grained in a chopper (Ultimate Chopper, Austria) before the analysis.
For the intramuscular implantation test, six samples of parallelepiped shaped material (1 × 2 × 3 mm) with rounded edges were made (n = 12). The samples were polished using a standard procedure in order to avoid mechanical irritation of the experimental animal tissue. According to the type of tested materials, the samples were divided into two experimental groups (G1 and G2), further subdivided into two groups depending on the observation time duration. All of the tested samples were disinfected with 70% ethanol and rinsed with saline (0.9% NaCl). The samples were stored in sterile Petri dishes at room temperature until implantation.
MHE-GC-MS and GC-FID analysis
An adequate amount of sample (cold or hot polymerized PMMA) was put in a headspace vial of 20 cm3, closed with aluminium crimp cap with silicon/PTFE septum (PTFE/Si, 20 mm, Agilent Technologies, USA) and placed in an HS autosampler (Agilent 7697A Headspace Sampler) of a gas chromatograph (Agilent Technologies 7890B) equipped with an inert, selective mass detector (Agilent MSD 5977A). Chromatography was performed by using a weakly polar, silica capillary HP-5MS column. Helium was used as the carrier gas, at a constant flow rate of 1 cm3/min. The parameters of the HS autosampler as well as the temperature programme are given in . The applied ionization voltage was 70 eV and mass detection was carried out in Scan mode, in m/z ranges of 35–650.
Table 2. Experimental conditions used for MHE-GC-MS analysis.
GC-FID analysis was carried out under identical experimental conditions as GC-MS analysis. The temperature of the flame-ionization detector (FID) was set at 300 °C. Data processing was performed using MSD ChemStation, MassHunter Qualitative Analysis and AMDIS_32 software (Agilent Technologies, USA). Compound identification was based on the comparison of their EI mass spectra with those available from NIST (NIST 11) and RTL (RTLPEST3) libraries. Peak areas were integrated automatically.
Calibration curve construction
The preparation of MMA standards was as follows: different masses of MMA monomer (ranging from 0.53 to 3.71 mg) were measured in five headspace vials of 20 cm3, closed with aluminium crimp caps and placed in the HS autosampler of the gas chromatograph. Every sample was extracted 10 times until almost exhaustive evaporation (around 2%) was achieved. The total peak area was determined and a calibration curve was constructed as dependency of total peak area on MMA mass (Supplementary material Figure S1).
Residual monomer analysis
The residual monomer content in the samples of hot and cold polymerized PMMA was determined under identical conditions to those applied for calibration curve construction.
Intramuscular implantation test
Ethics statement
Experimental investigations on animals were performed in accordance with the Helsinki Declaration (Approval of the Ethics Committee of the Faculty of Medicine in Nis, number 01-2066-1).
Experimental design
Laboratory male Wistar rats, 11–12 weeks of age and 180–200 g of average weight, were used in the experiment. Six animals were used for each of the tested materials (n = 12).
Implantation tests were designed for examining the biological response of the surrounding tissue to the tested materials upon their application (ISO 10994-6: 2007) [Citation23].
General anesthetized animals (10% Ketamidor, Richer Pharma AG, Austria, intraperitoneally) were placed in prone position on a special wooden framework. The implantation region was shaved and rinsed with Povidone iodine. The sample of the tested material was implanted in m.biceps femoris of the left leg of the experimental animal using a sterile needle 4/18. The wounds were left to heal spontaneously without antibiotic protection.
A one-week observation period and a two-month observation period were designed. After each of the observation periods, three animals from each experimental subgroup were sacrificed. Tube-like portions of muscular tissue surrounding the implants were taken as samples for the analysis. After histopathological processing, the samples were stained using a classical method – Hematoxylin & Eosin (HE) stain. The stained histopathological samples were analyzed pathohistologically using the image analysis system Lucia 3.2G (Laboratory Imaging, the Czech Republic) under a microscope NU-2 (Carl Zeiss, Germany). The microscopic analysis results were evaluated on the basis of the presence of inflammatory reaction, tissue fibrosis, degenerative changes, as well as potential necrosis and destructive changes in capillary walls. Healthy muscle tissue was used as control.
Results and discussion
MHE-GC-MS and GC-FID analysis
The reduction of the MMA peak area during ten successive extractions is shown in .
The evaporation profiles of the MMA standard as well as the evaporation profiles of MMA from cold and hot polymerized PMMA samples are shown in . The contribution of each MMA peak in the total MMA peak for ten successive extractions of MMA, PMMA cold and PMMA hot is shown in .
Figure 2. Evaporation profiles of MMA standard and MMA released from samples of cold polymerized and hot polymerized PMMA.
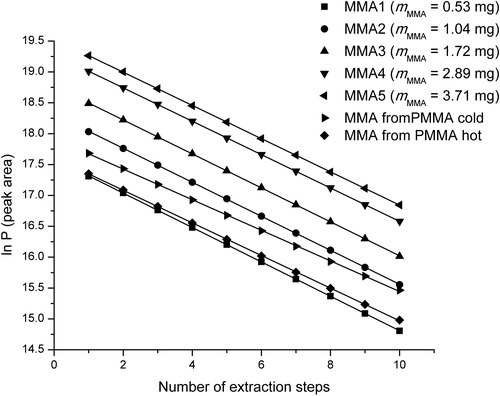
The evaporation profiles of the MMA standard and MMA from polymers were almost the same. The contribution of each extraction step in the total peak area was similar for all three samples (MMA, PMMA cold and PMMA hot), ranging from approximately 25% for the 1st extraction to about 2% for the 10th extraction. It is noticeable that the change of monomer concentration and the sample type did not lead to changes in the evaporation profile. Taking this into account and according to the obtained calibration curve and the linear equation, it was possible to determine the amount of MMA monomer in the samples of cold and hot polymerized PMMA ().
Table 3. Content of MMA in the samples of cold and hot polymerized PMMA.
The overlapped gas chromatograms of MMA and cold and hot polymerized PMMAs after the first extraction are shown in . The peak derived from MMA was present at retention time of 5.4 min and it absolutely matched the peak of the residual monomer in polymers.
Figure 4. Overlapped peaks (treatment 5.4 min) of MMA and cold and hot polymerized PMMA after the first extraction.
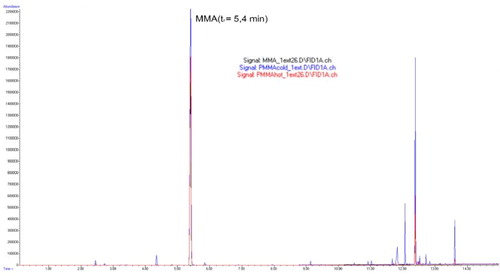
Other compounds identified in the sample of cold polymerized acrylate are shown in . The dominant compound present in the headspace is the residual, unreacted monomer MMA, present in about 90% of the MMA standard and PMMA hot sample and 71% of PMMA cold sample based on peak area. Match qualities above 80% are very strong and indicate a very close spectral match between the unknown peak and the NIST library entry. Definitive identification, however, requires comparison against analytical standards.
Table 4. Compounds identified in PMMA cold sample after 1st extraction by headspace GC-MS.
The mass spectrum of MMA used for monomer identification in polymers is shown in .
The obtained mass spectra were identical for MMA and for residual MMA in the samples of cold and hot polymerized PMMA, indicating the presence of residual MMA in the polymers. The mass spectrum of MMA monomer consisted of molecular ion observed at m/z 100, as well as fragmented ions observed at m/z 69 [C4OH5+], m/z 41 [C3H5+] and m/z 39 [C3H3+], which is in accordance with the literature data [Citation19,Citation21].
Intensive and long-term use of PMMAs in dentistry is a reliable proof of their good quality. Although imperfect, they fulfil most of the requirements imposed by dental practice. On the other hand, continuous research is performed in order to further improve the qualitative properties of the acrylic resins [Citation6].
Incomplete PMMA polymerization reduces the physico-mechanical and biological quality of dental restorations. It has been proven that unreacted MMA is an allergen and tissue irritant [Citation9,Citation24–26]. The monomer remaining in the resin structure could have a negative impact on the mechanical properties of the material by lowering the glass transition temperature (Tg) [Citation11]. The presence of residual monomer in the polymer decreases the molecular weight of the polymer and results in a more porous polymer structure, which significantly reduces hardness, strength and elasticity while increasing the solubility and liquid absorption [Citation11,Citation27–29]. Release of residual monomer in the saliva is compensated by water absorption, which leads to the plasticization of the material, making it more flexible and resilient [Citation28,Citation29].
The amount of residual monomer depends on the type of acrylate as well as the polymerization conditions [Citation30,Citation31]. Literature data show that the residual MMA amount is significantly higher in cold polymerized acrylate (5%) than in the hot polymerized acrylate (0.2–0.5%) [Citation32]. The obtained values of the MMA content determined by using MHE-GC-MS and GC-FID methods in samples of cold and hot polymerized acrylate were 15.75 mgMMA/gPMMA and 10.96 mgMMA/gPMMA, which corresponds to MMA content of 1.575% and 1.096%, respectively. As the process of cold acrylate polymerization at normal pressure and at the room temperature is often very rapid, the cold polymerized acrylates have non-homogenized, porous structure and, thus, a higher percent of residual reactants than hot polymerized acrylates. Therefore, it can be assumed that polymerization at high temperatures, close to the Tg value (105–124 °C) results in more compact structure of the polymer material and, therefore, a lower amount of potentially toxic substances, which is in accordance with literature data [Citation33,Citation34].
Intramuscular implantation test
A more intensive reaction was found during the first observation period. Pathological analysis of muscular tissue after a one-week observation period showed acute inflammatory reactions to the presence of the tested cold polymerized material. There was intensive presence of inflammatory cells (polymorphonuclears, lymphocytes, plasmocytes and macrophages), proliferation of connective tissue, as well as neovascularization (). Two months after implantation, fibrosis of the muscle tissue indicated healing of the observed tissue (). Hot polymerized implants caused low-intensity inflammatory changes as compared to cold polymerized implants (). This may be attributed to more complete polymerization of hot polymerized PMMA. The presence of the hot polymerized implants in muscle tissue led to the proliferation of connective tissue cells and intensive fibrosis, while the reaction had a chronic course.
Figure 6. Intense inflammatory reaction and de novo formation of blood vessels one week after implantation of cold polymerized acrylate in m.biceps femoris of male Wistar rats. HE, ×100.
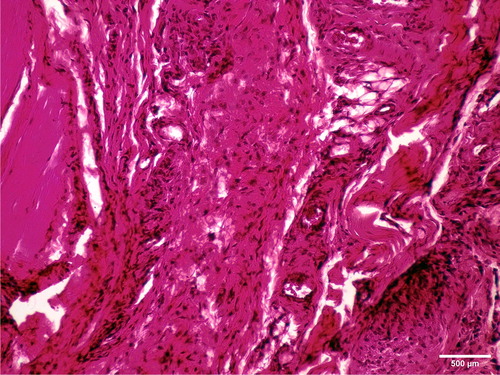
Figure 7. Slight fibrosis two months after implantation of cold polymerized acrylate in m.biceps femoris of male Wistar rats. HE, ×200.
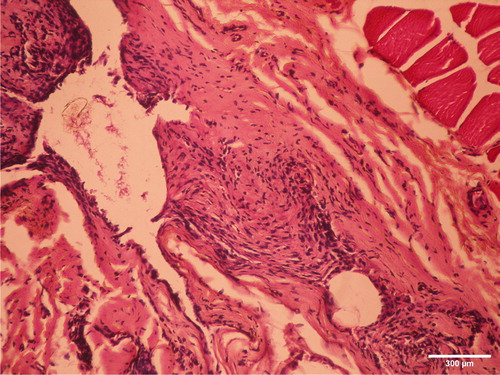
Figure 8. Proliferation of connective-tissue cells and intense fibrosis with a significantly lower intensity of inflammation one week after implantation in m.biceps femoris of male Wistar ratsof hot polymerized acrylic samples. HE, ×100.
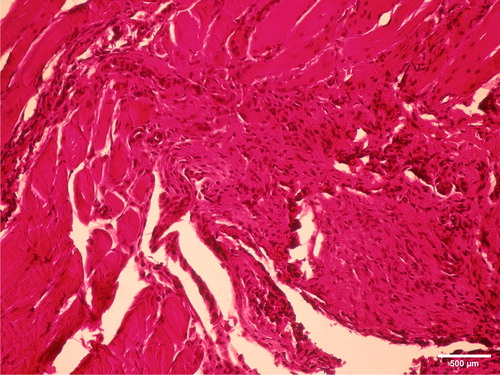
The results of tissue reaction test to the implanted material (ISO 10994-6: 2007) [Citation23] have no direct influence on clinical application. However, if it is assumed that the release of unpolymerized components from the acrylic materials adversely affects the tissues in contact with the material, this type of test may be useful. Cold polymerized acrylates induced intensive inflammation reaction after the implantation procedure, which is in accordance with the results of the examination of their cytotoxic effect [Citation35–37].
The release of residual monomer into the oral cavity is intensive [Citation38,Citation39], especially in the case of cold polymerized acrylates, which contain a significant amount of residual monomer. Some studies [Citation31–34] have shown that the additional polymerization (post-polymerization) of acrylate polymers and immersion into a water bath, can improve their biological and mechanical properties, by decreasing residual monomer content. In that way, the residual monomer release into the oral cavity can be significantly reduced. Therefore, MHE-GC-MS could also be applied for simple and efficient monitoring of post-polymerization processes in order to improve the quality and safety of synthesized polymers.
Conclusions
Multiple headspace extraction in combination with GC-MS and GC-FID is only recently gaining ground in polymer samples analysis. This method allows direct analysis of dental acrylic resins, without prior preparation of samples. By using this method, rapid and effective comparison of different dental acrylic materials is performed, enabling us to select the safer one, in this case hot polymerized PMMA, for use in clinical practice.
Supplemental Material
Download PDF (623.5 KB)Disclosure statement
The authors declare no conflict of interest.
Additional information
Funding
References
- Maitz MF. Applications of synthetic polymers in clinical medicine. Biosurf Biotribol. 2015;1(3):161–176.
- de Morais FAI, Mello BA, Souza IA, et al. Polymers a base methylmethacrylate. Importance in dentistry. Int J Dent. 2007;6:63–66.
- Frazer RQ, Byron RT, Osborne PB, et al. PMMA: an essential material in medicine and dentistry. J Long Term Eff Med Implants. 2005;15(6):629–639.
- LeSueur BW, Yiannias JA. Contact stomatitis. Dermatol Clin. 2003;21(1):105–114.
- Gawkrodger DJ. Investigation of reactions to dental materials. Br J Dermatol. 2005;153(3):479–485.
- Charasseangpaisarn T, Wiwatwarrapan C, Leklerssiriwong N. Ultrasonic cleaning reduces the residual monomer in acrylic resins. J Dent Sci. 2016;11(4):443–448.
- Lygre GB, Gjerdet NR, Gronningsaeter AG, et al. Reporting on adverse reactions to dental materials-intraoral observations at a clinical follow-up. Commun Dent Oral Epidemiol. 2003;31(3):200–206.
- Kostic M, Pejcic A, Igic M, et al. Adverse reactions to denture resin materials. Eur Rev Med Pharmacol Sci. 2017;21(23):5298–5305.
- Rashid H, Sheikh Z, Vohra F. Allergic effects of the residual monomer used in denture base acrylic resins. Eur J Dent. 2015;9:614–619.
- Goncalves TS, Morganti MA, Campos LC, et al. Allergy to auto-polymerized acrylic resin in an orthodontic patient. Am J Othod Dentofacial Orthop. 2006; 129:431–435.
- Lung CYK, Darvell BW. Minimization of the inevitable residual monomer in denture base acrylic. Dent Mater. 2005;21(12):1119–1128.
- Lung CYK, Darvell BW. Methyl methacrylate in poly(methyl methacrylate)-validation of direct injection gas chromatography. J Chromatogr A. 2004;1061(1):93–98.
- Hasenwinkel JM, Lautenschlager EP, Wixson RL, et al. Effect of initiation chemistry on the fracture toughness, fatigue strength, and residual monomer content of a novel high-viscosity, two-solution acrylic bone cement. J Biomed Mater Res. 2002;59(3):411–421.
- Mikai M, Koike M, Fujii H. Quantitative analysis of allergenic ingredients in eluate extracted from used denture base resin. J Oral Rehabil. 2006;33(3):216–220.
- Golbidi F, Asghari G. The level of residual monomer in acrylic denture base materials. Res J Biol Sci. 2009; 4:244–249.
- Ayman AD. The residual monomer content and mechanical properties of CAD\CAM resins used in the fabrication of complete dentures as compared to heat cured resins. Electron Physician. 2017;9(7):4766–4772.
- Iça RB, Öztürk F, Ates B, et al. Level of residual monomer released from orthodontic acrylic materials. Angle Orthod. 2014;84(5):862–867.
- Singh RD, Gautam R, Siddhartha R, et al. High performance liquid chromatographic determination of residual monomer released from heat-cured acrylic resin. An in vivo study. J Prosthodont. 2013;22(5):358–361.
- Linan LZ, Bonon A, Lima NMN, et al. Quality control of poly(methyl methacrylate) to medical purpose by multiple headspace extraction. Chem Eng Trans. 2013;32:1699–1704.
- Kostić M, Nikolić L. Contemporary dental materials. Adv Techol. 2019;8(1):78–85.
- Quintella CM, de Souza GGB, Maciel JB. Ionic fragmentation of methyl methacrylate induced by synchrotron radiation and multiphoton ionization. J Braz Chem Soc. 1998;9(6):521–524.
- Urban VM, Cass QB, Oliveira RV, et al. Development and application of methods for determination of residual monomer in dental acrylic resins using high performance liquid chromatography. Biomed Chromatogr. 2006;20(4):369–376.
- International Standards Organization (ISO). Biological evaluation of medical devices – part 6: tests for local effects after implantation. Geneva: ISO; 2007. Standard No. ISO 10993-6:2007.
- Davis CC, Squier CA, Lilly GE. Irritant contact stomatitis: a review of the condition. J Periodontal. 1998;69(6):620–631.
- Leggat PA, Kedjarune U. Toxicity of methyl methacrylate in dentistry. Int Dent J. 2003;53(3):126–131.
- Pfeifer CS, Shelton ZR, Braga RR, et al. Characterization of dimethacrylate polymeric networks: a study of crosslinked structure formed by monomers used in dental composites. Eur Polym J. 2011;47(2):162–170.
- Lung CYK, Darvell BW. Methyl methacrylate monomer-polymer equilibrium in solid polymer. Dent Mater. 2007;23(1):88–94.
- Jadhav SS, Mahajan N, Sethuraman R. Comparative evaluation of the amount of the residual monomer in conventional and deep-frozen heat cure polymethylmethacrylate acrylic resin: an in vitro study. J Indian Prosthodont Soc. 2018; 18(2):147–153.
- Azzarri MJ, Cortizo MS, Alessandrini JL. Effect of the curing conditions on the properties of an acrylic denture base resin microwave-polymerised. J Dent. 2003;31(7):463–468.
- Sadamori S, Ganefiyanti T, Hamada T, et al. Influence of thickness and location on the residual monomer content of denture base cured by three processing methods. J Prosthet Dent. 1994;72(1):19–22.
- Urban VM, Machado AL, Oliveira RV, et al. Residual monomer of reline acrylic resins: effect of water-bath and microwave post-polymerization treatments. Dent Mater. 2007;23(3):363–368.
- Vergani CE, Seo RS, Pavarina AC, et al. Flexural strength of autopolymerizing denture reline resins with microwave postpolymerization treatment. J Prosthet Dent. 2005;93(6):577–583.
- Teng H, Koike K, Zhou D, et al. High glass transition temperatures of poly(methyl methacrylate) prepared by free radical initiators. J Polym Sci A Polym Chem. 2009;47(1):315–317.
- Krunić N, Nikolić L, Kostić M, et al. In vitro examination of oral tissue conditioners potential toxicity. Hem Ind. 2011; 65:697–706.
- Dahl JE, Frangou-Polyzois MJ, Polyzois GL. In vitro biocompatibility of denture relining materials. Gerodontology 2006;23(1):17–22.
- Jorge JH, Giampaolo ET, Machado AL, et al. Cytotoxicity of denture base acrylic resins: a literature review. J Prosthet Dent. 2003;90(2):190–193.
- Kostić M, Krunić N, Nikolić L, et al. [Residual monomer content determination in some acrylic denture base materials and possibilities of its reduction]. Vojnosanit Pregl. 2009;66(3):223–227.
- Vallittu PK, Miettinen V, Alakuijala P. Residual monomer content and its release into water from denture base materials. Dent Mater. 1995;11(5-6):338–342.
- Kostic M, Nikolic L, Nikolic V, et al. Effects of water boiling, microwave, and water bath postpolymerization on mechanical properties of acrylic denture resins. Hem Ind. 2018;72(3):129–137.