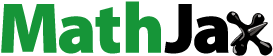
Abstract
Hip replacement is one of the most effective surgical procedures in medicine. Despite the fact that the clinical results of arthroplasty are usually excellent, some implants loosen with time and require revision. To help resolve this problem, research has focused on providing conditions that have a positive biological effect on bone building elements, resulting in a fast, seamless proliferation and differentiation of mesenchymal stem cells (MSC) into osteoblasts. That is why developing an in vitro model for studying MSC functions at the implant–tissue contact zone is essential. The aim of this study was to investigate the proliferation and differentiation capacity of human bone-marrow derived MSC in contact with medical implant-grade Ti-6Al-4V alloy and β-tricalcium phosphate (β-TCP). Human MSC were isolated from bone marrow aspirates obtained from the femoral canal during hip replacement surgeries and seeded on Ti-6Al-4V alloy squared specimens and β-TCP granules. The results indicate that MSC were able to effectively adhere to both biomaterial surfaces and, at the same time, retain their proliferative and osteogenic/adipogenic differentiation potential. We suggest that both Ti-6Al-4V alloy and β-TCP surfaces provide suitable conditions for the proliferation and differentiation of bone marrow MSC and can be used as in vitro models to explore MSC functions at the bone–implant interface.
Introduction
Joint replacement is one of the most common orthopaedic surgeries with well tracked and documented results over the last decades [Citation1]. Despite the significant evolution of arthroplasty prostheses over the years, the high incidence of bone implant failures still remains one of the major concerns, which are mainly ascribed to incomplete or ineffective osseointegration and to stress shielding due to significant mismatch of the mechanical properties between the implant and the surrounding bone [Citation2]. The successful integration between bone tissue and implanted biomaterial relies to a large extent on the contribution of mesenchymal stem cells (MSC) and their continuous proliferation and differentiation. The key importance of MSC for the processes of osseointegration and tissue regeneration is determined both by their function as precursors to osteoblasts and chondrocytes [Citation3, Citation4] and by their immunomodulatory, anti-inflammatory and pro-angiogenic properties [Citation5–7]. In arthroplasty and bone tissue engineering, in vitro testing of the interactions between MSC and diverse orthopaedic biomaterials represents an obligatory step [Citation8–10]. All biomechanical, biochemical and biological approaches that enhance bone integration and provide MSC with good functional capacity are a step forward in the endeavours to achieve life-long prosthetic replacement [Citation11]. To this end, there is a need for an effective in vitro model for studying MSC functions at implant–tissue interface. Therefore, in the present study we aimed to evaluate the response of human bone marrow-derived MSC to Ti-6Al-4V alloy and β-tricalcium phosphate (β-TCP) in terms of proliferation and differentiation features.
The titanium alloy Ti-6Al-4V has been extensively employed in orthopaedic–prosthetic replacements because of its superior corrosion resistance and biocompatibility combined with excellent mechanical properties [Citation12, Citation13]. However, titanium and titanium alloys still do not provide all the ideal implant properties and performance required in standard clinical practice. These could be improved in vivo by various surface modifications [Citation14]. A common method is coating the metallic surface with a thin film of bioactive materials, such as calcium phosphate, which is actively involved in bone bonding [Citation15–17]. Being the main inorganic component in bone tissue, calcium phosphate is inherently biocompatible and facilitates osseointegration [Citation18, Citation19].
Subjects and methods
Ethics statement
All patients provided written informed consent forms. Ethical approval for this study was acquired from the local Ethics Committee.
Clinical samples
Bone marrow biopsies (5–10 mL) were obtained from 8 patients (mean age: 58.1 years; range: 43–70 years) undergoing elective hip replacement surgery in the Clinic of Orthopedics and Traumatology, Queen Giovanna-ISUL University Hospital, Sofia, Bulgaria.
Materials
Ti-6Al-4V alloy (medical grade 5 titanium; ISO 5832-3) squared specimens (8 × 8 mm) were provided by Implant G Ltd. (Etropole, Bulgaria). High purity β-tricalcium phosphate (β-TCP; β-Ca3(PO4)2) granules (BIO 1®; ø 3,0 mm) were purchased from SBM (Lourdes, France).
Isolation and culture of human bone marrow-derived mesenchymal stem cells
MSC were isolated from fresh bone marrow aspirates and further characterized as previously described [Citation20]. Briefly, bone marrow samples were centrifuged at 300 g for 10 min and the supernatant plasma was discarded. The cell pellet was resuspended and incubated in erythrocyte (RBC) lysis buffer (0.15 mol/L NH4Cl; 0.1 mmol/L ethylenediaminetetraacetic acid (EDTA); 0.01 mol/L NaHCO3; pH 7.2 − 7.4). After erythrocyte removal, bone marrow nuclear cells were washed twice in sterile phosphate-buffered saline (PBS) and cultured in 10 cm2 6-well plates (EuroClone) at a concentration of 1.0 × 105 cells/cm2 in low-glucose Dulbecco’s modified Eagle’s medium (LG-DMEM; Sigma-Aldrich) supplemented with 10% faetal calf serum (FCS; Sigma-Aldrich) and 1% antibiotic/antimycotic solution (Sigma-Aldrich). After 48 h, nonadherent cells were discarded. Standard culture conditions were used (humidified atmosphere, 37 °C, 5% CO2) and the medium was changed every 4 days. At 80-90% confluence, adherent cells were harvested (0.05% trypsin/1.0 mmol/L EDTA; Sigma-Aldrich) and expanded in 25 cm2 flasks (EuroClone). Cells at second, third and fourth passages were used for further experiments.
Flow cytometry analysis
For the phenotypic characterization, MSC at 3rd passage were harvested (0.05% trypsin/1.0 mmol/L EDTA; Sigma-Aldrich), washed once and resuspended at a concentration of 1 × 105 cells per sample in PBS, pH 7.4. Surface markers were analysed using the following specific anti-human monoclonal antibodies: anti-CD45-FITC (2D1; eBioscience); -CD29-PE (MAR4; BD Pharmingen); -CD73-PE (AD2; BD Pharmingen); -CD90-FITC (5E10; eBioscience); -CD105-PE (SN6; eBioscience). In addition, isotype-matched negative control antibodies (mouse IgG1-FITC (203), ImmunoTools and mouse IgG1-PE (P3.6.2.8.1), eBioscience) were used to determine the level of background staining. After 30 min incubation (4 °C), labelled cells were washed twice in a CellWash solution (BD Biosciences) and fixed in FIX solution (BD Biosciences) as recommended by the manufacturer. The specific fluorescent labelling was analysed on a FACSCalibur flow cytometer (BD Biosciences) using the BD CellQuest Pro software (BD Biosciences).
Immunocytochemistry
Human MSC (passage 3) were seeded in triplicates on Ti-6Al-4V specimens and β-TCP granules and grown for 72 h at 37 °C, 5% CO2. Cells were fixed in 4% paraformaldehyde, pH 7.4 for 20 min and non-specific binding sites were blocked with 1% bovine serum albumin (BSA; Sigma-Aldrich) for 60 min at room temperature. Samples were incubated for 60 min at room temperature (RT) with the following commercial monoclonal antibodies: anti-CD29 (MAR4; BD Pharmingen); -CD73 (AD2; BD Pharmingen); -CD90 (5E10; eBioscience) and -CD105 (SN6; eBioscience). The secondary antibody used was an anti-mouse IgG Alexa Fluor 594 (1:1000, 60 min at RT; Invitrogen). Cell nuclei were counterstained with Hoechst 33258 (1:1000; Sigma-Aldrich) for 5 min at RT. The specimens/granules were rinsed with PBS, pH 7.4 between each step and mounted in Eukitt quick-hardening mounting medium (Sigma-Aldrich). Images were captured and analysed using confocal laser scanning microscopy (CLSM, Leica TCS-SPE).
MSC proliferation and population doubling time (PDT)
Alamar Blue (AB) assay was used to measure cell viability and proliferation. At passage 4, MSC were seeded in triplicates on Ti-6Al-4V specimens and β-TCP granules at an initial density of 2.0 × 104 cells/cm2. Control cultures were maintained on standard polystyrene (PS) plastic surface (96 well plate; EuroClone) at an initial density of 1.25 × 104 cells/cm2. Cellularized specimens/granules were cultured for 24 h and then moved to fresh wells (24/96-well plates respectively) prior to assay on day 0. All samples underwent continued culturing for maximum 7 days. Cell proliferation was evaluated on a daily basis by measuring the reduction of AB fluorometric/colorimetric growth indicator according to the manufacturer’s protocol (alamarBlue®, Bio-Rad Laboratories). The growth medium was replaced with 1:10 diluted AB stock solution in standard culture medium (LG-DMEM/10% FCS) and cells were incubated for 4 h at 37 °C in a humidified, 5% CO2 atmosphere. Thereafter, 100 μL aliquots were transferred to a fluoromicrotiter plate (Costar, Corning Incorporated) and fluorescence was measured at wavelengths of 544 nm (excitation) and 590 nm (emission) on a FLUOstar OPTIMA microplate reader (BMG Labtech). As this assay is non-toxic to cells, the same set of specimens/granules was used for further testing by adding fresh culture media. A standard graph was prepared by seeding a different number of cells in 24/96-well plates (EuroClone) and AB fluorescence intensity was evaluated as described. Each experiment was repeated 3 times and the mean value was presented as a final result.
The population doubling time (PDT) was calculated using the formula:
where t − t0 is culture time (h); C is the final cell concentration; and C0 is the initial cell concentration.
Osteogenic differentiation
MSC at passage 3 were plated on Ti-6Al-4V specimens, β-TCP granules and tissue culture polystyrene (control) at a density of 2 × 104 cells/cm2 in triplicates and were cultured in a specific osteogenic medium: LG-DMEM supplemented with 10% FCS, 1% antibiotic/antimycotic solution, 100 nmol/L dexamethasone (Sigma-Aldrich), 0.2 mmol/L ascorbic acid (Sigma-Aldrich) and 10 mmol/L β-glycerophosphate (Sigma-Aldrich). The osteogenic induction medium was replaced every 3 days for 3 weeks. For the negative control, cells were grown in LG-DMEM/10% FCS. At the end of the induction period, osteogenic differentiation rate was quantitatively determined by measuring the alkaline phosphatase (ALP) activity. Control osteogenic differentiated MSC were additionally stained with Alizarin red S.
Adipogenic differentiation
To promote adipogenic differentiation, MSC (3rd passage) were seeded at 2 × 104 cells/cm2 on Ti-6Al-4V specimens, β-TCP granules and polystyrene plastic surface (24 well plate; Costar) in triplicates and were grown to 80 − 90% confluence. Afterwards, adipogenic differentiation was induced by culturing the cells for 21 days in the following adipogenic medium: LG-DMEM supplemented with 10% FCS, 1% antibiotic/antimycotic solution, 1 µmol/L dexamethasone, 10 µg/mL bovine insulin (Sigma-Aldrich), 0.5 mmol/L 3-isobutyl-1-methyl-xanthine (IBMX; Sigma-Aldrich) and 60 µmol/L indomethacin (Sigma-Aldrich). The medium was refreshed twice a week. During the differentiation period, the non-induced control cells were maintained only in LG-DMEM/10% FCS. The presence of adipogenic differentiation was qualitatively (formation of neutral lipid-vacuoles) and quantitatively evaluated at day 21 on the basis of Oil red O staining.
Alkaline phosphatase assay
Osteogenically differentiated MSC were washed in PBS, pH 7.4 and permeabilized with 0.2% Triton X-100 (Sigma-Aldrich) in alkaline phosphatase buffer (0.05 mol/L Na2CO3, 0.5 mmol/L MgCl2; pH 9.5) for 20 min. For the assessment of ALP activity, 4-p-nitrophenyl phosphate (pNPP; Sigma-Aldrich) was used as a reaction substrate. Thus, 3.5 mmol/L pNPP in ALP buffer was added to the cell lysates and following an incubation period of 20 min at RT, the absorbance of the final coloured solution was measured spectrophotometrically at 405 nm wavelength (FLUOstar OPTIMA, BMG Labtech).
Alizarin red S staining
Calcium mineralization of extracellular matrix was evaluated as an advanced osteogenic marker using Alizarin red S staining. Briefly, differentiated MSC were washed with 0.9% NaCl, fixed with 4% paraformaldehyde (20 min, RT) and then immersed in 2% Alizarin red S (Sigma-Aldrich) solution at pH 4.2 for 30 min, followed by 3 washes in dH2O.
Oil red O staining
Oil red O staining was performed according to the optimized protocol described by Kraus et al. [Citation21]. Cells were rinsed in PBS, pH 7.4 and fixed with 4% paraformaldehyde for 20 min at RT. Washed cells were stained with 0.2% Oil Red O (Sigma-Aldrich) solution in 40% 2-propanol for 30 min at RT, followed by washing with dH2O to remove the unbound dye. The formation of intracellular lipid vacuoles was visualized and imaged using an inverted light microscope (AM 6000; Leica).
For quantitative assessment of the degree/level of differentiation, the dye was eluted with 100% 2-propanol for 10 min at RT on an orbital shaker. The eluate (100 µL) was transferred to a clear microtiter plate and absorption was measured at 485 nm wavelength (FLUOstar OPTIMA, BMG Labtech).
Statistical analysis
Data are expressed as mean values with standard deviation (±SD). Statistical significance of difference among mean values was evaluated by Student’s t test or Mann–Whitney rank sum test. The data were analysed using STATGRAPHICS Centurion XV (Statpoint Technologies, Inc.) and SigmaPlot 12.5 (Systat Software, Inc.) statistical software. For all analyses, differences were considered statistically significant at p ≤ 0.05. Correlation analyses were performed using CurveExpert Basic (version 1.4, Hyams Development) statistical software.
Results and discussion
Total joint replacement is recognized as the most cost-effective and reliable treatment for degenerative joint disease that features pain alleviation and full functional recovery [Citation22]. Despite its long-term successes, when it comes to maximizing the outcome, total joint replacement still faces multiple challenges, of which the ones with particular importance are the reduced ability of MSC to fully adhere and to behave in ways that improve bone restoration and joint functionality.
In relation to the bone compartment, mesenchymal stem cells comprise a population of multipotent progenitors that reside in bone marrow stroma, periosteum, endosteum and the walls of the local microvasculature [Citation23]. These are the cells that give rise to osteoblasts which in turn are the major cells involved in the development, growth, function and maintenance of bones [Citation24].
Immunophenotypic profile of the isolated MSC
All primary cell cultures used in this study were initially phenotypically and functionally characterized in order to verify their MSC identity. The purity of MSC cultures at passage 3 was found to be more than 95% (). Flow cytometry analysis () confirmed that culture-expanded MSC from all donors (n = 8) strongly expressed the specific panel of MSC surface markers (presented as mean ± SD values of the percentage of positively stained cells): CD29 (99.2 ± 0.32), CD73 (98.9 ± 0.55), CD90 (97.6 ± 2.21) and CD105 (95.9 ± 7.80), and were negative for the haematopoietic marker CD45 (3.4 ± 4.53).
Figure 1. Immunophenotypic characterisation of human bone marrow-derived MSC at passage three. (a) MSC cultures (n = 8) were highly positive for CD29, CD73, CD90 and CD105, and negative for CD45. Results are presented as mean ± SD. (b) Representative flow cytometry histograms of the fundamental surface markers used to define MSC.
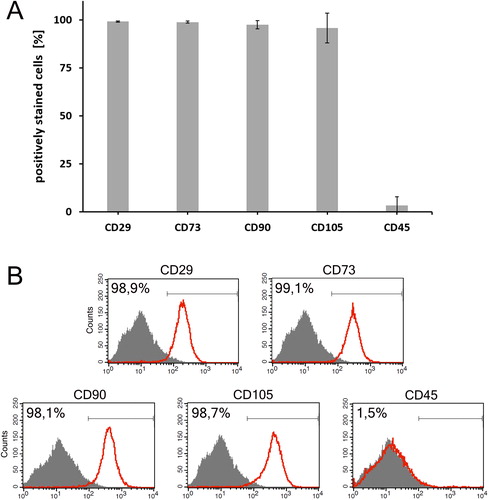
Proliferation activity and differentiation potential of MSC cultured on tissue culture polystyrene
Human MSC seeded on polystyrene surface were used as control cultures to determine the growth kinetics and differentiation potential of the isolated cells (). The proliferation capacity was evaluated during a 112-hour culture period. As demonstrated by the growth curve (), after a short lag-phase of about 24 h MSC grew exponentially until the last measurement point (i.e. 112nd hour). The population doubling time (PDT) of MSC cultured on polystyrene surface was calculated to be 36.9 h.
Figure 2. Characterization of MSC cultured on polystyrene plastic surface. (a) Growth kinetics curve. (b–k) Differentiation potential; Osteogenesis was displayed by calcium deposition (d, e) visualized with Alizarin Red staining (f and g are non-induced controls; magnification: d and f - × 100, e and g - × 200) and (b) measured quantitatively by ALP assay (results are shown as mean ± SD of 3 independent experiments; *p < 0.05). (h, i) Adipogenic differentiation was indicated by the accumulation of neutral lipid vacuoles stained with Oil Red O (j and k are non-induced controls; magnification, × 100) and (c) further quantitatively confirmed (results are presented as mean ± SD of 3 independent experiments; *p < 0.05).
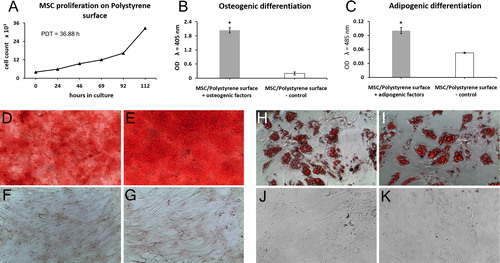
The osteogenic capacity of MSC was validated by an increased AP activity () and positive Alizarin red S staining () of the differentiated cultures. After 21 days of culture in osteogenic inductive medium, ALP activity in differentiated MSC was more than 10-fold higher compared to undifferentiated control cells (p < 0.05; ). Moreover, successful osteogenesis was confirmed by an intense red-stained extracellular matrix mineralization. The lack of extracellular calcium deposits in undifferentiated control cultures is shown in .
Adipogenesis was detected cytochemically (Oil red O staining) by the accumulation of multiple intracellular lipid-filled droplets in a significant fraction of the cells (). Undifferentiated control cells are shown in . The successful differentiation of MSC treated with adipogenic factors was confirmed by quantitative measurement of the degree of Oil red O staining (), which was found to be 2 times higher (p < 0.05) than that in control cultures.
All these findings concerning the phenotypic and functional characteristics of the cultured bone-marrow cells indicate that they possess all the required features to be defined as MSC, according to the minimal criteria of the International Society of Cellular Therapy [Citation25]. These include features like plastic-adherence, fibroblast-like morphology, expression of a specific panel of phenotypic markers and multipotent differentiation capabilities.
MSC adhesivity, growth kinetics and differentiation on Ti-6Al-4V alloy and β-TCP
Ingrowth and direct structural and functional integration of new bone tissue with the surface of the implant is defined as osseointegration [Citation26–28]. This process, which includes a series of biological events resembling direct fracture healing, mainly relies on the proper functions of MSC [Citation29]. Upon implantation, the prosthesis gets in contact with resident and invading MSC, whose ability to proliferate and differentiate in response to different growth factors and other biologically active molecules ultimately determines the successful outcome of bone-to-implant integration. Therefore, understanding the regulation of the mechanisms by which MSC exert their biologic activities on the biomaterial surface is essential for optimizing the long-term stability of prosthetic implants.
The objective of this study was to develop and establish a reliable and efficient in vitro culture system suitable for in-depth investigation of the behaviour and functionality of bone marrow-derived MSC cultures grown under different conditions and under the influence of various bioactive factors when in contact with the surface of two of the most commonly used biomaterials in endoprosthetics, Ti-6Al-4V alloy and β-TCP.
The ability of MSC to adhere to Ti-6Al-4V alloy specimens and β-TCP granules was assessed using confocal microscopy. As demonstrated in , 72 h after initial seeding, the presence and wide spreading of spindle-shaped fibroblast-like cells, positive for the standard basic panel of MSC phenotypic markers, were visualized on both biomaterial surfaces.
Figure 3. Representative fluorescence images of morphological and immunophenotypic characteristics of human bone marrow-derived MSC cultured on Ti-6Al-4V alloy specimens (left panel) and β-TCP granules (right panel). Cells were visualized by staining nuclei with Hoechst 33258 alone or in combination with specific antibodies against some of the surface markers characteristic for MSC. Scale bar = 100 μm. H, Hoechst.
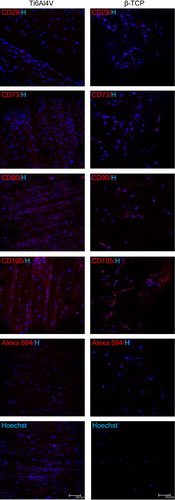
The data from the AB assay indicated that human bone marrow-derived MSC were able not only to effectively adhere to the surface of both tested materials, but also to proliferate continuously forming standard S-shaped growth curves (). Despite the active proliferation, the MSC cultured on Ti-6Al-4V alloy and β-TCP grew at much slower rates compared to the polystyrene cultures (), with PDT of 112.4 h and 56.6 h, respectively ().
Figure 4. Proliferation ability and differentiation potential of MSC cultured on Ti-6Al-4V alloy (a, c, e) and β-TCP (b, d, f). (a and b) MSC proliferation profiles on Ti-6Al-4V alloy specimens (a) and β-TCP granules (b) evaluated with Alamar Blue assay. (c and d) Differentiation towards osteogenic lineage quantitatively demonstrated by ALP assay. (e and f) Quantification of adipogenic differentiation by measuring the absorbance of Oil red O eluates. Data are presented as mean ± SD; *p < 0.05.
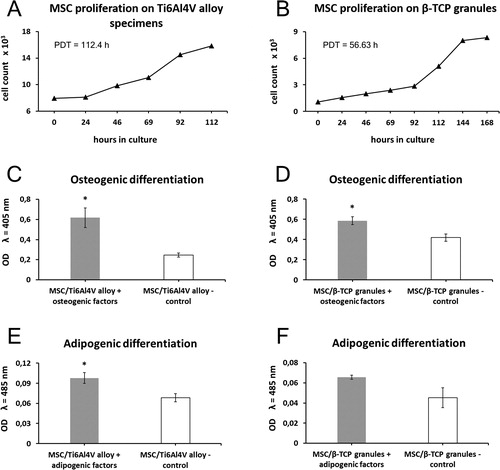
These results agree with previous studies [Citation30, Citation31] which also reported decreased proliferative activity of bone marrow-derived MSC grown on Ti-6Al-4V alloy and β-TCP surfaces compared to those seeded on tissue culture plates. However, there are reports of no differences between Ti-6Al-4V alloy and polystyrene plastic surface in terms of MSC proliferation rates [Citation32, Citation33]. All these contradictory data may be due to different experimental design, conditions, approaches and/or methodology used by different researchers, which makes it impossible to draw clear and accurate conclusions from all the diverse and incomparable studies.
We further demonstrated that MSC grown on both Ti-6Al-4V alloy and β-TCP retained their potential for osteogenic and adipogenic differentiation. For osteogenically stimulated MSC cultured on both Ti-6Al-4V specimens and β-TCP granules, the quantitative AP assay revealed significantly higher levels of alkaline phosphatase activity (2.6-fold and 1.5-fold, respectively, p < 0.05; ) in comparison to those for the undifferentiated control cells, indicating a successful osteogenic differentiation.
Furthermore, MSC that had been loaded onto both culture systems, were capable of adipogenic differentiation. Compared to undifferentiated controls, MSC seeded on Ti-6Al-4V alloy () and β-TCP () showed a marked increase in Oil red O staining intensity (1.4-fold and 1.4-fold respectively) after the 21-day treatment with adipogenic factors.
There are a number of recent reports on the biological properties of different or newly developed and promising calcium phosphate-based ceramics and titanium alloys [Citation16, Citation34–37]. However, they all reflect a common research approach to analyze or compare biomaterial properties based on how structural diversities or chemical/physical modifications of the tested biomaterials may affect MSC responses and functional activity. In contrast to this approach, we did not aim to evaluate the advantages and disadvantages of the biomaterials that we used in the context of their interaction with MSC. Our goal was to demonstrate methodologically how, while preserving their biological properties and physical characteristics, these biomaterials could be implemented in vitro as a platform for the research of the mechanisms and the factors that could improve MSC proliferation and differentiation. By using well-characterized, clinically approved and commercially available biomaterials such as Ti-6Al-4V alloy and β-TCP, which are commonly used for the production of arthroplasty prostheses, we demonstrated an easily reproducible and methodologically accessible in vitro system for studying MSC functions at the bone-implant interface. On the basis of our current and previous [Citation38] results it can be concluded that both Ti-6Al-4V alloy and β-TCP surfaces provide favourable conditions for the proliferation and multilineage differentiation of bone marrow MSC, and are suitable for use in in vitro models for the study of MSC functions at the bone–implant contact zone.
Conclusions
Here we evaluated the ability of well-predefined and characterized human BM-MSC to exert some of their key functional characteristics while cultured on size- and shape-standardized specimens made of two tested biomaterials. The primary cell cultures used in our model satisfied the phenotypical and functional criteria employed to define MSC. The response of bone marrow-derived MSC to Ti-6Al-4V alloy and β-TCP showed that these cells maintained to some extent their proliferative capacity and osteogenic/adipogenic differentiation potential. Furthermore, the data indicated that the methods applied in our model for the detection and evaluation of cell proliferation and differentiation possess sufficient reliability and sensitivity, which make their usage convenient in these particular experimental conditions. Both Ti-6Al-4V alloy and β-TCP surfaces provided favourable conditions for the proliferation and multilineage differentiation of bone marrow MSC. These models can be used in vitro in studies on MSC functions at the bone–implant contact zone.
Disclosure statement
No potential conflict of interest was reported by the author(s).
Additional information
Funding
References
- Katz JN. Total joint replacement in osteoarthritis. Best Pract Res Clin Rheumatol. 2006;20(1):145–153.
- Webster TJ. Nanophase ceramics: the future orthopedic and dental implant material In: Ying JY, editor. Advances in Chemical Engineering. Advances in Chemical Engineering, 27. New York: Academic Press; 2001; p. 125–66.
- James AW. Review of signaling pathways governing MSC osteogenic and adipogenic differentiation. Scientifica (Cairo). 2013;2013:684736.
- Giuliani N, Lisignoli G, Magnani M, et al. New insights into osteogenic and chondrogenic differentiation of human bone marrow mesenchymal stem cells and their potential clinical applications for bone regeneration in pediatric orthopaedics. Stem Cells Int. 2013;2013:1–11.
- Iyer SS, Rojas M. Anti-inflammatory effects of mesenchymal stem cells: novel concept for future therapies. Expert Opin Biol Ther. 2008;8(5):569–581.
- Kaplan JM, Youd ME, Lodie TA. Immunomodulatory activity of mesenchymal stem cells. Curr Stem Cell Res Ther. 2011;6(4):297–316.
- Chen L, Tredget EE, Wu PY, et al. Paracrine factors of mesenchymal stem cells recruit macrophages and endothelial lineage cells and enhance wound healing. PLoS One. 2008;3(4):e1886.
- Jorgensen C, Gordeladze J, Noel D. Tissue engineering through autologous mesenchymal stem cells. Curr Opin Biotechnol. 2004;15(5):406–410.
- Anderson JM, Langone JJ. Issues and perspectives on the biocompatibility and immunotoxicity evaluation of implanted controlled release systems. J Control Release. 1999;57(2):107–113.
- Fukuda N, Kanazawa M, Tsuru K, et al. Synergistic effect of surface phosphorylation and micro-roughness on enhanced osseointegration ability of poly(ether ether ketone) in the rabbit tibia. Sci Rep 2018;8(1):16887.
- Pajarinen J, Lin TH, Nabeshima A, et al. Mesenchymal stem cells in the aseptic loosening of total joint replacements. J Biomed Mater Res. 2017;105(4):1195–1207.
- Niinomi M. Recent metallic materials for biomedical applications. Metall and Mat Trans A. 2002;33(3):477–486.
- Long M, Rack HJ. Titanium alloys in total joint replacement–a materials science perspective. Biomaterials 1998;19(18):1621–1639.
- Kulkarni M, Mazare A, Schmuki P, Igliĉ A. Biomaterial surface modification of titanium and titanium alloys for medical applications In: Seifalian A, de Mel A, Kalaskar D, editor. Nanomedicine. Manchester, UK: One Central Press; 2014. p. 111–136.
- Viteri VS, Fuentes E. Titanium and Titanium Alloys as Biomaterials In: Gegner J, editor. Tribology – Fundamentals and Advancements. Rijeka, Croatia: Intech; 2013. p. 155–181.
- Chudinova EA, Surmeneva MA, Timin AS, et al. Adhesion, proliferation, and osteogenic differentiation of human mesenchymal stem cells on additively manufactured Ti6Al4V alloy scaffolds modified with calcium phosphate nanoparticles. Colloids Surf B Biointerfaces. 2019;176:130–139.
- Su Y, Cockerill I, Zheng Y, et al. Biofunctionalization of metallic implants by calcium phosphate coatings. Bioact Mater. 2019;4:196–206.
- Suchanek WL, Yoshimura M. Processing and properties of hydroxyapatite-based biomaterials for use as hard tissue replacement implants. J Mater Res. 1998;13(1):94–117.
- LeGeros RZ. Properties of osteoconductive biomaterials: calcium phosphates. Clin Orthop Relat Res. 2002;(395):81–98.
- Bochev I, Elmadjian G, Kyurkchiev D, et al. Mesenchymal stem cells from human bone marrow or adipose tissue differently modulate mitogen-stimulated B-cell immunoglobulin production in vitro. Cell Biol Int. 2008;32(4):384–393.
- Kraus NA, Ehebauer F, Zapp B, et al. Quantitative assessment of adipocyte differentiation in cell culture. Adipocyte 2016;5(4):351–358.
- Pajarinen J, Lin TH, Sato T, et al. Interaction of materials and biology in total joint replacement - successes, challenges and future directions. J Mater Chem B Mater Biol Med. 2014;2(41):7094–7108.
- Knight MN, Hankenson KD. Mesenchymal stem cells in bone regeneration. Adv Wound Care (New Rochelle). 2013;2(6):306–316.
- Jayakumar P, Di Silvio L. Osteoblasts in bone tissue engineering. Proc Inst Mech Eng H. 2010;224(12):1415–1440.
- Kyurkchiev D, Bochev I, Ivanova-Todorova E, et al. Secretion of immunoregulatory cytokines by mesenchymal stem cells. WJSC. 2014;6(5):552–570.
- Isaacson BM, Jeyapalina S. Osseointegration: a review of the fundamentals for assuring cementless skeletal fixation. Orthop Res Rev 2014;6:55–65.
- Albrektsson T, Albrektsson B. Osseointegration of bone implants. A review of an alternative mode of fixation. Acta Orthop Scand. 1987;58(5):567–577.
- Guglielmotti MB, Olmedo DG, Cabrini RL. Research on implants and osseointegration. Periodontol 2000. 2019;79(1):178–189.
- Deschaseaux F, Sensebe L, Heymann D. Mechanisms of bone repair and regeneration. Trends Mol Med. 2009;15(9):417–429.
- Lu H, Kawazoe N, Tateishi T, et al. In vitro proliferation and osteogenic differentiation of human bone marrow-derived mesenchymal stem cells cultured with hardystonite (Ca2ZnSi 2O7) and {beta}-TCP ceramics. J Biomater Appl. 2010;25(1):39–56.
- Mante M, Daniels B, Golden E, et al. Attachment of human marrow stromal cells to titanium surfaces. J Oral Implantol. 2003;29(2):66–72.
- Tognarini I, Sorace S, Zonefrati R, et al. In vitro differentiation of human mesenchymal stem cells on Ti6Al4V surfaces. Biomaterials 2008;29(7):809–824.
- Deligianni DD, Katsala N, Ladas S, et al. Effect of surface roughness of the titanium alloy Ti-6Al-4V on human bone marrow cell response and on protein adsorption. Biomaterials. 2001;22(11):1241–1251.
- Pereira RC, Benelli R, Canciani B, Scaranari M, et al. Beta-tricalcium phosphate ceramic triggers fast and robust bone formation by human mesenchymal stem cells. J Tissue Eng Regen Med. 2019;13(6):1007–1018.
- Cunha A, Zouani OF, Plawinski L, et al. Human mesenchymal stem cell behavior on femtosecond laser-textured Ti-6Al-4V surfaces. Nanomedicine (Lond.). 2015;10(5):725–739.
- Rojo L, Gharibi B, McLister R, et al. Self-assembled monolayers of alendronate on Ti6Al4V alloy surfaces enhance osteogenesis in mesenchymal stem cells. Sci Rep. 2016;6(1):30548.
- Li Y, Jiang T, Zheng L, et al. Osteogenic differentiation of mesenchymal stem cells (MSCs) induced by three calcium phosphate ceramic (CaP) powders: A comparative study. Mater Sci Eng C Mater Biol Appl. 2017;80:296–300.
- Antonov B, Bochev I, Mourdjeva M, et al. Porous coated titanium implants do not inhibit mesenchimal stem cells proliferation and osteogenic differentiation. Biotechnol Biotec Eq. 2013; 27(6):4290–4293.