Abstract
Bilirubin oxidases (BODs), which catalyze the oxidation of bilirubin to biliverdin, are multi-copper oxidases that can be used in clinical diagnoses. Given their different reported enzymatic characterizations from laccases, BODs have gained considerable attention for their application in healthcare and environment management. In this study, a BOD gene from Myrothecium verrucaria was chemically synthesized and optimized to heterogeneous expression in Arabidopsis thaliana. The optimized DNA sequence showed 80.1% similarity to the original sequences. The gene was successfully transferred into A. thaliana, and the crude extracts of transgenic plants showed higher BOD activity compared with that of untransformed plants. BOD expressed in transgenic A. thaliana also exhibited an activity on the typical substrate (ABTS) of laccase and was able to decolorize the textile dyes crystal violet and malachite green. The results indicated that the M. verrucaria BOD gene could be transgenically exploited in plants for dye decolorization.
Introduction
Bilirubin oxidase (BOD), first found in guinea pigs in 1969, can catalyze the oxidation of bilirubin [Citation1]. In 1981, Murao and Tanaka isolated and purified BOD from Myrothecium sp, which was used to detect bilirubin for the first time [Citation2]. Later, they elaborated the characteristics of BOD from Myrothecium and then provided an approach for the application of BOD in determining bilirubin [Citation3]. This reaction can be used to determine the bilirubin content in serum by measuring the reduction in absorbance at 450 nm [Citation4]. In clinical examinations, BOD is used to measure the concentration of bilirubin in the serum and to confirm jaundice diagnosis; however, no methods are found for directly determining the serum levels of unconjugated bilirubin [Citation5,Citation6]. In addition to its clinical application, BOD plays an important role in bio-battery and biosensor manufacturing, cloth bleaching, and effluent degradation [Citation7].
BOD, which belongs to the multi-copper oxidase family, has attracted significant interest due to its utilization in decolorization of dyes, biofuel cells, and biosensors [Citation8]. The absorption and ESR spectra show that both BODs from Myrothecium verrucaria are multi-copper oxidases containing type 1, type 2, and type 3 copper ligands similar to laccase, ascorbate oxidase, and ceruloplasmin [Citation9]. Laccase is also a typical multi-copper-containing enzyme that was first reported in the sap of a Japanese lacquer tree, Rhus vernicifera [Citation10]. Laccases can use molecular oxygen to oxidize a broad spectrum of organic compounds by a radical-catalyzed reaction mechanism [Citation11]. Laccase has been extensively studied because of its ability to oxidize phenols, polyphenols, aromatic amines and other nonphenolic compounds [Citation12]. Although laccases and BODs belong to the same enzyme family of MCOs, the latter are less studied. In contrast to most laccases, BODs display high thermal stability, low sensitivity to chloride ions, and high activity in neutral pH, which make them excellent candidates for the four-electron reduction of O2 to water and their use in biofuel cells [Citation13].
At present, synthetic dyes are widely used in textile, cosmetic, printing, pharmaceuticals, and food processing industries, which produce a large amount of industrial wastewater containing dyes, thereby causing serious environmental pollution [Citation14]. The decolorization of dye wastewater by the action of laccase has been widely explored [Citation15,Citation16]. However, the catalytic activity of most laccases is in the range of acidic pH [Citation17], which restricts the further application of biological decolorization. Therefore, BOD can be a potential alternative to laccase, which is thus far primarily restricted to applications in biological treatment. BOD can also reportedly degrade rimazole brilliant blue R (RBBR) [Citation8]. This finding demonstrates that BOD has the potential for wastewater decolorization. In recent years, the application of BOD has been gradually broadened; BOD has been used in the manufacture of biological batteries and in the treatment of environmental wastewater [Citation18–20]. Furthermore, BODs are attractive candidates for enzyme cathodes in biofuel cells and in biosensors, because they are stable under physiological conditions in contrast to laccase [Citation8].
In this study, the BOD gene from M. verrucaria was optimized and heterologously expressed in Arabidopsis thaliana to evaluate the activity of BOD and the decolorization ability of transgenic plants.
Materials and methods
Chemicals, plant materials and growth conditions
Bilirubin and other chemicals used in the tests were obtained from Sinopharm Chemical Reagent Co., Ltd. (Shanghai, China) and were of analytical grade. All enzymes for DNA manipulation were purchased from TaKaRa (Dalian, China). 2-2′-Azino-bis-3-ethylbenzothiazoline-6-sulfonate (ABTS) was obtained from Shanghai Sangon Co., Ltd (Shanghai, China). Agrobacterium tumefaciens GV3101 and A. thaliana (ecotype Columbia L) plants were sourced from our laboratory. The seeds of A. thaliana were surface sterilized with bleaching powder (5%, w/v) for 20 min, washed with sterile water three times, placed in Petri dishes that contained Murashige and Skoog medium with 0.8% agar, and then placed in darkness at 4 °C for 3 days. All the plants grew in a growth room at 22 °C under standard long-day conditions (16:8 h day/night cycle and ∼120 µmol photons·m−2·s−1 light intensity).
Construction of the plant expression vector and plant transformation
The DNA sequence of BOD (E.C.1.3.3.5) from M. verrucaria was designed and synthesized based on the amino acid sequence of BOD. The synthetic gene was cloned into the pMD18-T vector and sequenced. The amino acid sequences used for analysis were retrieved from the database of the National Center for Biotechnology Information (http://www.ncbi.nlm.nih.gov/). The artificial DNA fragment of MvBODI was cloned into a pCAMBIA1301 vector, and the amplification sequence was subcloned between the HindIII and EcoRI sites. The constructed MvBODI/pCAMBIA1301 was introduced into Escherichia coli DH5α by electroporation and transformed into A. thaliana by floral dip method to generate transgenic plants and investigate the function of the BOD gene. Transgenic plants were obtained from plants grown on a medium containing 40 mg·L−1 hygromycin.
Expression of the MvBOD gene in A. thaliana
Genomic DNA was extracted from 3-week-old transgenic and wild-type (WT) seedlings. Polymerase chain reactions (PCR, Bio-Rad, T100 Thermal Cycler, Hercules, CA, USA) were run in 30 cycles lasting for 30 s at 94 °C, 30 s at 55 °C, and 30 s at 72 °C, with a final extension at 72 °C for 10 min with the primer BOD-1 (5-CTTGGTAGAAGAGAGACTGTC-3′) and BOD-2 (5-TTCATCAGCAGCAGCGTATGG-3′). The PCR products were separated on 1% (w/v) agarose gels.
The 3-week-old transgenic and WT seedlings were used to extract total RNA using the multisource total RNA miniprep kit according to the manufacturer’s instructions (Axygen, USA). A reverse transcription system was conducted using 5 µg of total RNA as the template (Promega, USA), and the products were qualitatively amplified by RT-PCR (reverse transcription-polymerase chain reaction). PCR and electrophoretic procedures were the same as previously described.
Crude protein was extracted from similar 3-week-old seedlings. Transgenic and WT plants were ground in liquid nitrogen using a mortar and pestle and homogenized using 0.2 mol L−1 citrate–phosphate buffer (pH 8.0). Plant tissues were suspended in this buffer at a concentration of 100 mg mL−1. The homogenate was centrifuged at 12,000 rpm and 4 °C (Beckman Coulter, Microfuge® 20 R, CA, USA), and the supernatant was used as crude enzyme. Native-PAGE was performed according to the reported method [Citation21]. ABTS was replaced with bilirubin (2.0 g L−1).
Effect of different parameters on enzyme activity
The effect of pH on the enzymatic activity was monitored with a reaction system (200 µL). The reaction mixture for the crude enzyme activity assay contained 2.0 mg mL−1 of bilirubin (10 µL) or 4 mmol L−1 of ABTS (10 µL) and 190 µL of crude enzyme (pH 2.2–8.0). The reaction system with ABTS was incubated at 37 °C for 1 h, and the reaction system with bilirubin was incubated at 37 °C for 12 h. Furthermore, at the optimal pH, the optimal temperature of crude enzyme was detected. The assay mixture was incubated at different temperatures between 20 °C and 80 °C at increments of 10 °C (1 h). The reduction in the absorbance of bilirubin and ABTS was measured at 445 and 420 nm with a micro-plate reader (Tecan, Infinite 200 Pro NanoQuant, SUI) to determine the effect of enzymatic activity.
CV and MG decolorization by recombinant BOD from A. thaliana
Fresh-weight transgenic line and WT seedlings (fresh weight of 100 mg) were obtained and ground in liquid nitrogen using a mortar and pestle and homogenized using 0.2 mol L−1 citrate–phosphate buffer at pH 4.0. The centrifuged (12,000 rpm, 4 °C, Beckman Coulter, Microfuge® 20 R, CA, USA) culture medium was used as crude enzyme. To determine the dye decolorization by crude enzyme, the experiment dye decolorization was assayed as follows: 180 µL of crude enzyme of seedlings was suspended with phosphoric acid-citrate (pH 4.0) and reacted with crystal violet (CV, 0.34 mmol L−1, 10 µL) and malachite green (MG, 1.0 mmol L−1, 10 µL) in the presence or absence of ABTS (4 mmol L−1, 10 µL) . The assay mixture was incubated at 37 °C for 12 h, and dye decolorization was evaluated by monitoring the decrease in maximum absorbance (CV, 595 nm; MG, 620 nm) by using a micro-plate reader (Tecan, Infinite 200 Pro NanoQuant, SUI).
CV and MG decolorization by WT and transgenic A. thaliana plants
WT and transgenic A. thaliana were transferred into MS liquid media (20 mL) with and without ABTS. CV (0.34 mmol L−1, 10 µL) and MG (1.0 mmol L−1, 10 µL) were added into these liquid media and grown for 36 h and 6 h at 28 °C. Samples were obtained from the media, and the plants were studied. To test the decolorization rate of these dyes, the reaction systems were detected with a micro-plate reader (Tecan, Infinite 200 Pro NanoQuant, SUI) (MG 620 nm and CV 595 nm). Decolorization was compared based on the absorbance reduction at the maximum absorbance wavelength of each dye and throughout the visible spectrum.
Results and discussion
Optimization and synthesis of the MvBOD gene
The BOD holoenzyme is composed of a polypeptide, two divalent copper ions, and a certain amount of carbohydrates. To improve the expression in A. thaliana, the codon usage was optimized to fit the plant codon preference. The G + C and A + T contents in the gene were balanced to improve the efficiency of gene transcription and RNA stability. The restriction enzyme sites in the MvBOD gene were eliminated before insertion into the vector, and the Bam HI and Sac I sites were added to the 5′- and 3′- end, respectively. The optimized DNA sequence was named MvBODI and showed 80.1% similarity to the original sequence. The DNA fragment was inserted into the vector to construct the expression vector MvBODI/pCAMBIA1301. This expression vector was transferred into A. tumefaciens GV3101 for plant transformation.
Transformation and expression of the BOD gene in A. thaliana
The genomic DNA extracted from A. thaliana seedlings was subjected to PCR to confirm the insertion of the foreign gene (). The PCR analysis also revealed that the BOD gene was successfully transformed in all of the six transgenic lines. The transgenic plants 471-4, 471-5, 471-10, 471-11 and 471-13 were selected for further investigation. Using the actin gene and WT plants as references, the foreign gene was actively and stably transcribed in the transgenic plants as demonstrated by RT-PCR (). Bilirubin could be oxidized to biliverdin. When the gel was stained for 24 h, the biliverdin became colorless and was oxidized by BOD under the slow action of oxygen and light. Therefore, the location of the BOD corresponds to a large band ().
Figure 1. Recombination, transcription and expression of Mv BODI in transgenic A. thaliana plants. The recombination and transcription of MvBOD in transgenic lines (471-4, 471-5, 471-10, 471-11, and 471-13) were confirmed by PCR (A) and RT-PCR (B). Native-PAGE of crude protein extracts of the transgenic lines (471-4,471-5, 471-11) (C).
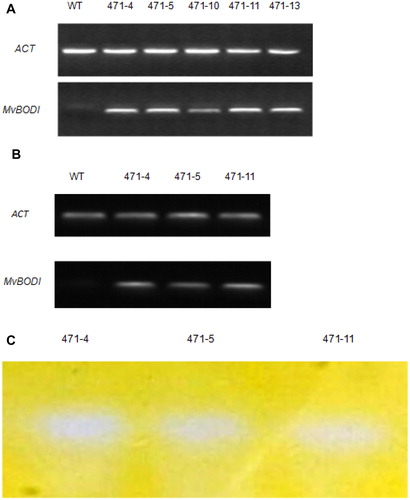
Effect of pH on the crude enzyme extracted from transgenic plants
To identify the activity and characteristics of recombinant BOD, the effect of pH on the crude enzymatic activity of BOD was determined at different pH values ranging from 2.2 to 8 using citrate–phosphate buffer. With bilirubin and ABTS as substrates, at different pH values, the analysis of bilirubin and ABTS spectra at 445 and 420 nm showed the optimal pH for bilirubin and ABTS. shows that the optimal pH for the crude enzyme differed depending on the substrates. The crude enzyme activity in BOD was only 33.4% when the catalytic reaction was carried out at pH 2.2, and attained a maximum value for bilirubin at pH 7. A similar result could be found in BOD from Bacillus pumilus [Citation22]. The present study showed that the BOD from M. verrucaria parent and mutant reached maximum enzyme activity for bilirubin at 40 °C when the reaction was observed at its optimal pH 7 [Citation23]. In addition, the optimal pH of BOD from Paraconiothyrium variabile for bilirubin was 7.5, and this BOD had strong stability and enzyme activity in the pH range of 6–11 [Citation24]. However, the enzyme activity was a maximum at pH 4.0 for ABTS. The optimal pH for the oxidation of ABTS was similar to that of other studies [Citation8,Citation25]. Such activity was higher at acidic pH than that at neutral pH. It was only 20.9% when the catalytic reaction was performed at pH 7.0. The results revealed that with different substrates, the optimal pH might be different, and the crude enzyme activity in a broad pH range might be considered as an advantage to broaden the scope of BOD application.
Figure 2. Effect of pH on the BOD activity in crude extracts from transgenic A. thaliana plants.
Note: The reactions were performed at 37 °C with bilirubin (0.1 mg mL−1, 12 h) and ABTS (0.2 mmol L−1, 1 h). The enzyme assays were performed in triplicate. Enzyme activity at optimal pH was assumed to be 100%. Relative activity was calculated as percentage of enzyme activity to maximum enzyme activity.
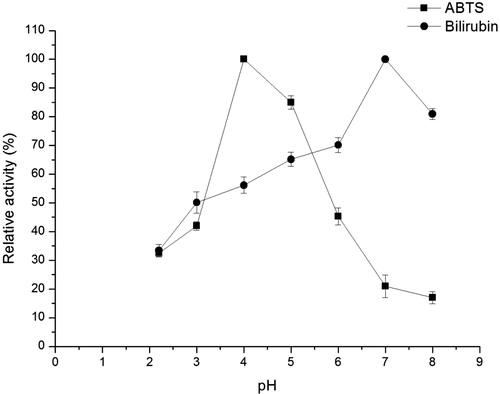
Effect of temperature on the crude enzyme extracted from transgenic plants
The effect of temperature on the crude enzyme activity by BOD was determined at different temperatures ranging from 20 °C to 60 °C during a one-hour incubation using citrate-phosphate buffer. Using different substrates at optimal pH, bilirubin and ABTS spectra were measured at 445 and 420 nm to determine the optimal temperatures. As shown in , with different substrates at different pH values, the optimal temperatures were also different. For bilirubin, the enzyme activity increased at temperatures ranging from 20 °C to 60 °C during a 1-hour incubation. The maximum enzyme activity was obtained at approximately 60 °C. At a temperature above 60 °C, the activity decreased; the activity was only 8% at 80 °C. However, the maximum enzyme activity from M. verrucaria was 40 °C [Citation7,Citation26]. In addition, the different optimal temperatures might be due to the different ecological origins and culture conditions. When ABTS was used as the substrate of the crude enzyme, the maximum activity was obtained at 40 °C, which was similar to Pakhadnia [Citation27] and Xu Han [Citation7]. Moreover, the activities at temperatures from 40 °C to 80 °C were reduced remarkably. Whether bilirubin is the substrate or ABTS, the enzyme activity could be maintained at above 40% at 20 °C. This finding showed that the enzyme might be used at room temperature. Furthermore, the difference between the optimal temperature of ABTS and bilirubin might be due to the substrate properties and pH conditions. In the present study, at above 80 °C, the crude enzyme almost cannot oxidize ABTS and bilirubin. The crude enzyme activity was enhanced with the increase in temperature to a certain degree, but at above 60 °C, the enzymatic activity decreased which might be due to enzyme inactivation at high temperature [Citation28].
Figure 3. Effect of temperature on BOD activity in the crude extracts from transgenic A. thaliana plants.
Note: The enzyme activity was measured in 0.2 mol L−1 phosphate–citrate buffer (pH 4) for ABTS (0.2 mmol L−1) or pH 7.0 for bilirubin (0.1 mg mL−1) at different temperatures (20 °C–80 °C) with increments of 10 °C for 1 h. The enzyme assays were performed in triplicate. Enzyme activity at optimal temperature was assumed to be 100%. Relative activity was measured by percentage of enzyme activity to maximum enzyme activity.
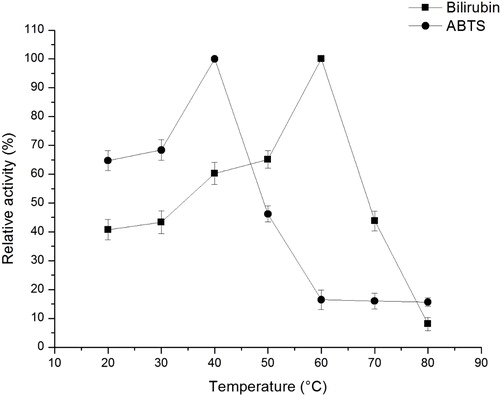
Decolorization of various triphenylmethane dyes by the crude enzyme extracted from transgenic plants
At present, many studies have been conducted on the degradation of dyes by laccases, but some of the laccases served as redox mediators when degrading dyes [Citation21,Citation29]. Claus et al. [Citation30] found that the laccase plus mediator system enhanced dye decolorization. BOD and laccase share similar mechanisms in decolorization, that is, electron transfer coupled with reduction of molecular oxygen to water, resulting in the oxidation of dye molecules [Citation30]. To evaluate the ability of BOD to degrade different dyes, we first tested the decolorizing ability of crude enzyme with and without ABTS as mediator. Using the crude enzyme extracts from transgenic and WT seedlings, the decolorization rates for MG and CV were determined (). As shown in , when the catalytic reaction was carried out at pH 4.0 for 12 h, the decolorization rate of MG by BOD in A. thaliana reached 93.2% with ABTS as mediator. The decolorization rate of CV can reach 70.9%. Without ABTS, the decolorization rates of MG and CV were 20.7% and 6.5%, respectively. The result demonstrated that the crude enzyme extract could degrade CV and MG, and with ABTS as mediaor, the decolorization rates were significantly improved. Liu et al. [Citation28] studied the improvement of RBBR decolorization efficiency with and without ABTS and found that ABTS can serve as a mediator in BOD-catalyzed RBBR decolorization. However, mediators were not required for BOD from Myrothecium sp. IMER1 to degrade RBBR dyes directly and effectively [Citation29].
Figure 4. Decolorization rate of CV and MG by crude enzyme of BOD from transgenic A. thaliana.
Note: Decolorization of CV (0.017 mmol L−1,) and MG (0.05 mmol L−1,) by crude enzyme of BOD expressed in transgenic A. thaliana. Decolorization was detected with or without 0.2 mmol L−1 ABTS as a mediator for 12 h.
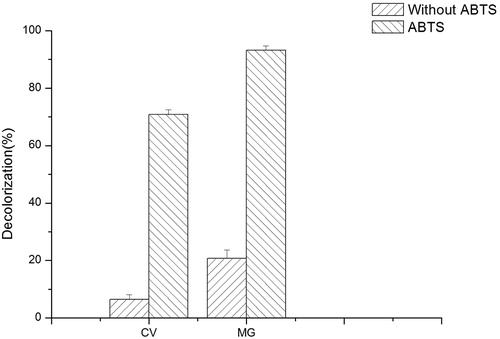
Dye decolorization by WT and transgenic A. thaliana plants
The decolorization rates of CV and MG by WT and transgenic plants from the hydroponic system are shown in . As shown in , after 36 h, the transgenic seedlings could decolorize CV and MG without ABTS, and almost all the CV and MG added in the media were degraded in the presence of ABTS. The results showed that ABTS, as a mediator, can effectively promote the degradation of CV and MG. show the decolorization rate of MG and CV by WT and transgenic seedlings. After 12 h of incubation, with ABTS as a mediator, the decolorization rate of CV reached 76.5%, and that without ABTS was only 43.0%. The decolorization rate reached 98.8% at 36 h with ABTS. At 5 h, the decolorization rate of MG reached 95.4% in the presence of ABTS, and that without ABTS reached 66.2%. In addition, the transgenic seedlings and the crude enzyme extract from the transgenic seedlings could degrade MG and CV without ABTS, but the decolorization rates of transgenic seedlings were relatively low. As shown in , WT A. thaliana plants could also decolorize or degrade CV and MG due to the adsorption of dyes onto the plant roots and extracellular enzymes produced by microbes and plants and direct mediation of organic matter breakdown [Citation31–34].
Figure 5. Analysis of decolorization by the hydroponic system. (A) Decolorization effect of WT and transgenic A. thaliana growing in liquid medium containing MG (0.05 mmol L−1,) for 6 h. (B) Decolorization effect of WT and transgenic A. thaliana growing in liquid medium containing CV (0.017 mmol L−1,) for 36 h. (C) Rate of MG decolorization by transgenic A. thaliana. Reaction incubation at 28 °C for 6 h. (D) Rate of CV decolorization by transgenic A. thaliana. Reaction incubation at 28 °C for 36 h.
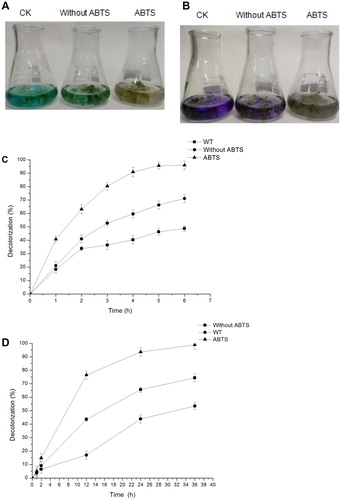
Our result indicated that the BOD gene from M. verrucaria can be transgenically exploited into plants for bioremediation. This finding may be applied in environmental remediation to degrade harmful dyes released into the environment. Different technologies might be used for decolorization of wastewater containing dyes; among them, biological processes are the most promising because they are environmentally safe [Citation35]. Environmental remediation through single physical, chemical or biological measures is difficult; therefore, combining biological methods with physical or chemical ones could improve its application scope and address its limitations.
Conclusions
This study aimed to identify the expression of exogenous BOD gene in A. thaliana and verify the decolorization ability of the recombinant protein and transgenic plants to dyes. The BOD gene from M. verrucaria could be expressed in A. thaliana. Such BOD could effectively decolorize MG and CV with ABTS. Furthermore, these results demonstrated that M. verrucaria BOD gene might be transgenically exploited in plants for bioremediation. Our results indicated that BOD has a high activity at neutral and acidic pH and unusual enzymatic properties in the presence of ABTS as well as high activity to decolorize textile dyes. Therefore, BOD could be a potential alternative to laccase, which is primarily restricted to applications in acidic media. Moreover, BOD could be suitable for environmentally sustainable applications.
Disclosure statement
No potential conflict of interest was reported by the author(s).
Additional information
Funding
References
- Brodersen R, Bartels P. Enzymatic oxidation of bilirubin. Eur J Biochem. 1969;10(3):468–473.
- Murao S, Tanaka N. A new enzyme “bilirubin oxidase” produced by Myrothecium verrucaria MT-1. Agric Biol Chem. 1981;45(10):2383–2384.
- Murao S, Tanaka N. Isolation and identification of a microorganism producing bilirubin oxidase. Agric Biol Chem. 1982;46(8):2031–2034.
- Seki Y, Takeguchi M, Murao S, et al. Abnormal effect of mercury ions on bilirubin oxidase activity. J Mol Catal A: Chem. 1995;96(1):L11–L13.
- Hooda V, Gahlaut A, Gothwal A, et al. Bilirubin enzyme biosensor: potentiality and recent advances towards clinical bioanalysis. Biotechnol Lett. 2017;39(10):1453–1410.
- Iwatani S, Nakamura H, Kurokawa D, et al. Fluorescent protein-based detection of unconjugated bilirubin in newborn serum. Sci Rep. 2016;6:28489.
- Han X, Zhao M, Lu L, et al. Purification, characterization and decolorization of bilirubin oxidase from Myrothecium verrucaria 3.2190. Fungal Biol. 2012;116(8):863–871.
- Durand F, Gounel S, Kjaergaard CH, et al. Bilirubin oxidase from Magnaporthe oryzae: an attractive new enzyme for biotechnological applications. Appl Microbiol Biotechnol. 2012;96(6):1489–1498.
- Shimizu A, Kwon JH, Sasaki T, et al. Myrothecium verrucaria bilirubin oxidase and its mutants for potential copper ligands. Biochemistry. 1999;38(10):3034–3042.
- Yoshida H. Chemistry of lacquer (urushi) Part I. J Chem Soc. 1883;43(0):472–486.
- Strong P, Claus H. Laccase: A review of its past and its future in bioremediation. Crit Rev Environ Sci Technol. 2011;41(4):373–434.
- Gianfreda L, Xu F, Bollag JM. Laccases: a useful group of oxidoreductive enzymes. Biorem J. 1999;3(1):1–26.
- Mano N. Features and applications of bilirubin oxidases. Appl Microbiol Biotechnol. 2012;96(2):301–307.
- Lamia A, Jihane C, Neji L, et al. Biodegradation of crystal violet by an isolated Bacillus sp. Ann Microbiol. 2009;59(2):267–272.
- Wikee S, Hatton J, Turbé-Doan A, et al. Characterization and dye decolorization potential of two laccases from the marine-derived fungus pestalotiopsis sp. Int J Mol Sci. 2019;20:1864.
- Khlifi R, Belbahri L, Woodward S, et al. Decolourization and detoxification of textile industry wastewater by the laccase-mediator system. J Hazard Mater. 2010;175(1-3):802–808.
- Liu J, Cai Y, Liao X, et al. Purification and characterisation of a novel thermal stable laccase from pycnoporus Sp. SYBC-L3 and its use in dye decolorisation. Biol Environ Proc R Irish Acad. 2013;113(1):1–39.
- Mano N, Edembe L. Bilirubin oxidases in bioelectrochemistry: Features and recent findings. Biosens Bioelectron. 2013;50:478–485.
- Ivnitski D, Artyushkova K, Atanassov P. Surface characterization and direct electrochemistry of redox copper centers of bilirubin oxidase from fungi Myrothecium verrucaria. Bioelectrochemistry. 2008;74(1):101–110.
- Sakasegawa SI, Ishikawa H, Imamura S, et al. Bilirubin oxidase activity of bacillus subtilis CotA. Appl Environ Microbiol. 2006;72(1):972–975.
- Wang B, Wang X, Tian Y, et al. Heterologous expression and characterization of a laccase from Laccaria bicolor in Pichia pastoris. Biotechnol Biotechnol Equip. 2015;30(1):1–6.
- Durand F, Kjaergaard CH, Suraniti E, et al. Bilirubin oxidase from Bacillus pumilus: A promising enzyme for the elaboration of efficient cathodes in biofuel cells. Biosens Bioelectron. 2012;35(1):140–146.
- Bayineni VK, Suresh S, Sharma A, et al. Improvement of bilirubin oxidase productivity of Myrothecium verrucaria and studies on the enzyme overproduced by the mutant strain in the solid-state fermentation. J Gen Appl Microbiol. 2018;64(2):68–75.
- Kataoka K, Tanaka K, Sakai Y, et al. High-level expression of Myrothecium verrucaria bilirubin oxidase in Pichia pastoris, and its facile purification and characterization. Protein Expr Purif. 2005;41(1):77–83.
- Durão P, Chen Z, Fernandes AT, et al. Copper incorporation into recombinant CotA laccase from Bacillus subtilis: characterization of fully copper loaded enzymes. J Biol Inorg Chem. 2008;13(2):183–193.
- Moreira-Gasparin FG, De Souza CG, Costa AM, et al. Purification and characterization of an efficient poultry feather degrading-protease from Myrothecium verrucaria. Biodegradation. 2009;20(5):727–736.
- Pakhadnia YG, Malinouski NI, Lapko AG. Purification and characteristics of an enzyme with both bilirubin oxidase and laccase activities from mycelium of the basidiomycete Pleurotus ostreatus. Biochemistry Mosc. 2009;74(9):1027–1034.
- Liu YX, Huang J, Zhang X. Decolorization and biodegradation of remazol brilliant blue R by bilirubin oxidase. J Biosci Bioeng. 2009;108(6):496–500.
- Zhang X, Liu Y, Yan K, et al. Decolorization of anthraquinone-type dye by bilirubin oxidase-producing nonligninolytic fungus Myrothecium sp. IMER1. J Biosci Bioeng. 2007;104(2):104–110.
- Claus H, Faber G, König H, et al. Redox-mediated decolorization of synthetic dyes by fungal laccases. Appl Microbiol Biotechnol. 2002;59(6):672–678.
- Schwab AP, Al-Assi AA, Banks MK. Adsorption of naphthalene onto plant roots. J Environ Qual. 1998;27(1):220–224.
- Qianfeng L, Jiasong F, Jiangtao L, et al. Depth-resolved variations of cultivable bacteria and their extracellular enzymes in the water column of the new britain trench. Front Microbiol. 2018;9:135.
- Elk M. A survey of plant root extracellular enzyme activity in native and invasive exotic plants of oak openings. Electronic Thesis or Dissertation. University of Toledo; 2010. Available from: https://etd.ohiolink.edu/.
- Wesenberg D, Kyriakides I, Agathos SN. White-rot fungi and their enzymes for the treatment of industrial dye effluents. Biotechnol Adv. 2003;22(1–2):161–187.
- Przystaś W, Zabłocka-Godlewska E, Grabińska-Sota E. Efficiency of decolorization of different dyes using fungal biomass immobilized on different solid supports. Braz J Microbiol. 2017;49(2):285–295.