Abstract
Colletotrichum fructicola causes strawberry anthracnose, which results in considerable economic losses in quality and production in Asia. However, the molecular mechanism of strawberry infection with C. fructicola is still poorly understood. Here, we employed RNA-Seq technology to mine the strawberry transcriptome for EST-SSRs (Expressed Sequence Tag–Simple Sequence Repeats) following C. fructicola infection. Fortunately, we detected 3019 potential EST-SSRs from the transcriptomic data of cv. Jiuxiang. The seven commonly occurring repeat motifs were A/T (278), GAA/TTC (263), GAG/CTC (215), AAG/CTT(188), GGA/TCC (163), AGA/TCT (153) and AGG/CCT (153). We identified 31, 84 and 129 differentially expressed EST-SSR-containing sequences corresponding to 24, 72 and 96 Hpi, while 13 DE-EST-SSRs -containing sequences were differentially expressed and common for all the three time points. Furthermore, we identified allele polymorphisms for some DE-EST-SSRs among several resistant and susceptible strawberry varieties and wild species. The EST-SSRs in our research were related to the reported QTL resistance loci in strawberry which are differentially expressed after C. fructicola infection and have allele polymorphisms in different strawberry varieties and wild species. These provide a clue for utilisation of the EST-SSRs in marker-assisted breeding to improve anthracnose-resistant breeding in future.
Introduction
The cultivated strawberry (Fragaria ×ananassa), also known as ‘the queen of fruits’, is very popular with consumers due to its flavor and nutritional value. Fragaria ×ananassa are generally octoploid with 2n = 8x = 56 chromosomes. Anthracnose caused by Colletotrichum spp. is one of the most devastating diseases for cultivated strawberry [Citation1–3], and can cause major problems in the industry of strawberry cultivation where infections are thought to be spread primarily through latently infected plants [Citation4, Citation5]. C. fructicola was formerly known as C. gloeosporioides, and is responsible for strawberry anthracnose in China, Korea and Japan [Citation5–8].
In strawberry, some research has been devoted to elaborating the molecular mechanism related to anthracnose resistance. Two class II chitinase genes are cloned, and analyzed in the strawberry plant [Citation9]. The genes that encode 03B3-thionins, peroxidases, chitinases and 03B2-1-3-glucanases are up-regulated during pathogen infection [Citation10], as shown by microscopic investigations of Colletotrichum fragariae infection [Citation11], and proteomic analyses of strawberry leaves infected with Colletotrichum fragariae [Citation12]. The SA- and JA-dependent signaling pathways are activated in strawberry upon interactions with Colletotrichum acutatum [Citation13]. Comparative transcriptomics revealed differential gene expression related to Colletotrichum gloeosporioides resistance in the octoploid strawberry ‘Yanli’ and ‘Benihoppe’ [Citation14].
In our lab, some progress has also been made in strawberry anthracnose research. NB-LRR genes display ecotype-specific responses to C. gloeosporioide in woodland strawberry [Citation15]. Salicylic acid is involved in the interactions between Colletotrichum gloeosporioides and two strawberry varieties [Citation16]. FaNBS-RPW8 fusions are related to C. fructicola infection in strawberry (Fragaria ×ananassa) [Citation17]. In the early strawberry/Colletotrichum fructicola interaction, a rapid quenching of the burst of host salicylic acid is common [Citation18]. Novel fungal pathogenicity is revealed by transcriptome analysis of Colletotrichum fructicola and strawberry [Citation19].
Simple Sequence Repeat (SSR) markers are abundant and related to transcribed sequences in plant genomes [Citation20]. EST-SSRs (Expressed Sequence Tag–Simple Sequence Repeat) markers are also used to explain the genetic diversity, germplasm characterization and population genetics [Citation21]. Traditional methods for SSR development are expensive and labor-intensive by the screening of genomic libraries and sequencing of positive clones. Now, the transcriptomic resources promote SSR markers development expedient. SSRs from GenBank sequences in the species of Fragaria and Rubus have been previously identified using two different data-mining methods [Citation22]. Four hypervariable SSR markers are developed to identify 75 accessions of strawberry cultivars [Citation23]; 96 strawberry cultivars are distinguished by amplifying SSR fragments from 18 SSR primer pairs [Citation24].
However, the EST-SSR markers related to strawberry anthracnose are still largely unknown or poorly explored. In this study, we generated comparative EST-SSR markers at different time periods after inoculation in susceptible cv. Jiuxiang (Fragaria ×ananassa), uncovering new resistance-related genes, EST-SSR markers by comparative transcriptomics analyses. These data deepen our understanding of EST-SSR markers and molecular mechanisms involved in strawberry defense.
Materials and methods
Plant materials and treatments
All the strawberry cultivars used in this work were from Shanghai Academy of Agricultural Sciences (SAAS) resource nursery, and were grown in a multi-span greenhouse at the Zhuanghang field trial station of SAAS.
For RNA-Seq, only the cultivar of ‘Jiuxiang’ was used, as it is comparatively susceptible to C. fructicola. For genotyping, two strawberry cultivars (‘Benihoppe’ and ‘Sweet Charlie’) were used. ‘Benihoppe’ is susceptible to C. fructicola, whereas ‘Sweet Charlie’ is resistant to C. fructicola [Citation14]. Two wild species were used, including F. vesca (‘Yellow wonder’ and ‘Hawaii4’), and F.nilgerrensis (‘Schlecht’).
For each independent C. fructicola inoculation experiment, healthy plants at the sixth compound stage were cultivated from a nursery in pots with sterilized substrate.
Three technical replicates from 15 independent individuals were used (five plants per replicate). Before C. fructicola inoculation, the pots were transferred to a growth chamber (Conviron, Adaptis A1000AR, Winnipeg, Canada) for 2 weeks. The conditions in the growth chamber were set as follows: 12-h-light/12-h-dark cycle, 125 μ mol m- 2 s−1 photo flux density, 70% relative humidity (RH) and a constant temperature of 25 °C. After inoculation, the plants were cultivated under continuous darkness. The compound leaves of ‘Jiuxiang’ at the sixth compound leaves stage were collected at 0, 24, 72 and 96 Hpi for RNA-Seq.
Cultivation of C. fructicola
The C. fructicola isolate (cgmcc3.17371) was maintained at the Shanghai Academy of Agricultural Sciences. The plant growth conditions and inoculation methods have been previously described [Citation17].
RNA-Seq
The cultivar Jiuxiang was infected with C. fructicola for RNA-Seq. C. fructicola was inoculated on leaves at the sixth compound leaves stage. We prepared the samples during the process of infection with C. fructicola at 24, 72 and 96 Hpi. RNA-Seq, construction of Illumina library, sequencing and raw reads have been previously described in our group [Citation19].
Mapping reads to the reference genome
Clean reads were preprocessed by removing the adaptor sequences and discarding the empty and low-quality sequences. The reads were then mapped to Fragaria_vesca_ v1.1.a2 [Citation25] using Bowtie 2 (version2.1.0) with the default parameters. Then we analyzed the key DEGs in enriched pathways and EST-SSR in fragaria_vesca_v4.0.a2 [Citation26] with the locus IDs of two nomenclatures between v2.0.a1 and v4.0.a2 ().
Identification Est-SSR markers and primers design
We employed MIcroSAtellite (MISA, v-1.0, http://pgrc.ipk-gatersleben.de/misa/misa.html) for microsatellite mining[Citation27]. In this study, the EST-SSRs were considered to contain motifs of one to six nucleotides in size and a minimum of 5 contiguous repeat units. The parameter was set to the default values (1-10, 2-6, 3-5, 4-5, 5-5 and 6-5). Therefore, the core unit was a single base, repeated 10 times or more; the core unit was two bases, repeated more than 6 times, and so on. The MISA results, Primer3 v2.3.5 (http://www-genome.wi.mit.edu/genome_software/other/primer3.html) was used to design the primer pairs with the default setting, and the size of PCR product ranged from 100 to 280 bp. Six varieties were selected to validate the polymorphism of 160 random SSR markers.
Identification of DE-Est-SSRs
The gene expression levels were calculated using the RPKM method (fragments per kb per million reads). Samtools were used to obtain the RPKM values. The RPKM values were ≥ 0.3. Baggerley’s test and the false discovery rate (FDR) had a significance level of ≤ 0.001, and the p-value was ≤ 0.01. The ratios of RPKM at 24, 72 and 96 Hpi to 0 Hpi ≥ 2 or ≤ 0.5 (fold change >1) were set as the threshold to determine the differentially expressed genes.
DNA extraction, Est-SSR amplification and genotyping
The genotyping of DE-EST-SSRs was done using genomic DNA extracted from strawberry varieties and wild species using the CTAB method. The DNA quality was confirmed using 1% agarose gels, and the quantity was confirmed using a UV–visible Spectrophotometer and a nano spectrophotometer (Implen, Westlake Village, CA, USA), respectively. The working concentration of DNA was adjusted to 50 ng μL−1.
The DE-EST-SSR primers were synthesized by Shanghai Sangon Biological Engineering Technology (Shanghai, China).
Polymerase chain reactions (PCRs) were performed in 20 μL reaction volumes containing 25 ng of template DNA, 10 μL of PrimeSTAR Max DNA Polymerase (TaKaRa, Japan), 1 μL of 10 μmol/L of each primer and 7 μL sterile distilled water. The following PCR program included an initial step at 98 °C for 3 min followed by 32 cycles of 98 °C for 10 s, the appropriate annealing temperature (temperature depended on primers) for 5 s at 55-60 °C and 5 s at 72 °C, and a final extension for 5 min at 72 °C in an Eppendorf Mastercycler PCR instrument. Finally, the products were examined in 1% agarose gels. Amplified products, which showed a band of the expected size, were sequenced for genotyping by Shanghai Sangon Biological Engineering Technology (Shanghai, China).
Results and discussion
Identification and characterization of the Est-SSR markers
In order to develop the strawberry anthracnose EST-SSR markers, we screened all 31,029 mapped genes in this transcriptome to mine EST-derived SSR motifs. In total, 3,019 putative EST-SSRs were detected in 2,497 genes, including 204 genes with one or more than one SSR (). The major motif types of the identified EST-SSRs were trinucleotides (2298; 76.12%), dinucleotides (363; 12.24%) and mononucleotides (292; 9.68%). We also detected some other motif types (2.2%), such as hexanucleotides, tetranucleotides and pentanucleotides, in this transcriptome (). In general, the number of EST-SSRs detected in each size category (mono-, di-, tri-, hexa-) decreased with increasing repeat number (). The top five abundant motifs of the trinucleotide repeats were (GAA/TTC)5 (127; 4.2%), (GAG/CTC)5 (118; 3.9%), (AAG/CTT)5 (117; 3.8%), (GGA/TCC)5 (100; 3.3%) and (AGA/TCT)5 (96; 3.2%). For the dinucleotide repeats, (AG/CT)6 (65; 2.2%) was the most common motif, followed by (GA/TC)6 (54; 1.8%). For the mononucleotide repeats, the most abundant motif was (A/T) > 10 (167; 5.50%), followed by (A/T)10 (111; 3.7%) ().
Figure 1. The distribution of repeat unit and repeat number for EST-SSRs in cv. Jiuxiang transcriptome. (A) Distribution of repeat units for mono-, di-, tri-, tetra-, penta- and hexa-nucleotide. (B–E) Distribution of the repeat number for mono- (B), di- (C), tri- (D), and hexa-nucleotides (E).
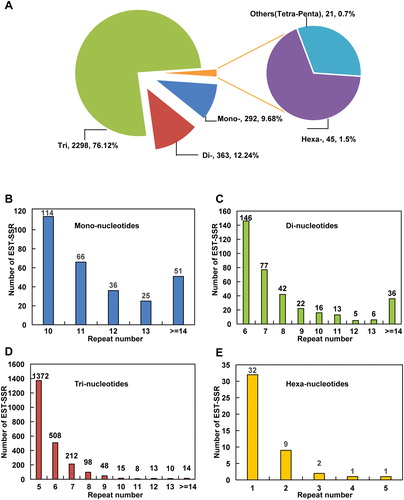
Table 1. Statistic of EST-SSR detected in ‘Jiuxiang’.
Table 2. Frequency of mono-, di- and trinucletide EST-SSR repeat motifs in cv. Jiuxiang.
Within the mono-, di- and trinucleotides, five tandem repeats (1393; 46.14%) were the most frequently observed, followed by those with six tandem repeats (647; 21.43%) and seven tandem repeats (293; 9.7%) (, ). Approximately 79% (2,388) of the identified EST-SSRs were 20 bp in length, whereas only 3% (92) of the EST-SSRs were longer than 20 bp. A total of 2,510 SSR-containing sequences were adequate for primer design, whereas the remaining 256 sequences did not qualify for primer design because the sequences flanking the SSRs were too short or inappropriate for primer design.
DE- Est-SSRs after C.fructicola inoculation
In our study, we reported the differentially expressed EST-SSRs (DE-EST-SSRs) after C.fructicola inoculation in strawberry. Interestingly, a total of 31, 84 and 129 differentially expressed EST-SSR-containing sequences were detected in 24 Hpi, 72 Hpi and 96 Hpi compared with 0 Hpi, respectively (). Thirteen differentially expressed EST-SSR-containing sequences (named EST-SSR01- EST-SSR13) showed increasing or decreasing expression patterns in all three libraries simultaneously () in this transcriptome. Furthermore, the expression patterns of six EST-SSR-containing sequences (EST-SSR01, EST-SSR03, EST-SSR06, EST-SSR10, EST-SSR11 and EST-SSR13) were downregulated in susceptible cv. Jiuxiang at 24 Hpi and 72 Hpi, and the reduced expression level was maintained at 96 Hpi. Three EST-SSR-containing sequences (EST-SSR02, EST-SSR09 and EST-SSR12) were downregulated in susceptible cv. Jiuxiang in the three libraries continuously. Two EST-SSR-containing sequences (EST-SSR07 and EST-SSR08) were slightly upregulated at 24 Hpi and then were sharply downregulated at 72 Hpi and 96 Hpi. Two other EST-SSR-containing sequences (EST-SSR04 and EST-SSR05) were stably upregulated in the three libraries simultaneously (). Of the 13 differentially expressed EST-SSR-containing sequences, there were 4 transcription factors. Three differentially expressed EST-SSR transcription factors were MYB family proteins, and one was WRKY DNA-binding protein (, ). Previous reports have shown that MYB and WRKY transcription factors can participate in the resistance response against pathogen infection by regulating defense genes [Citation28, Citation29]. TaWRKY70 was identified within the QTL-2DL region, along with three resistance genes that biosynthesize RRI metabolites to resist Fusarium graminearum infection in wheat[Citation30]. Here, we deduced that the DE-EST-SSR6, 8, 13 and 11, which are encoded by MYB and WRKY DNA-binding protein, might play an important role in strawberry defense against C. fructicola infection. EST-SSR1 belongs to facilitator superfamily, which plays an important role in multidrug resistance in fungi [Citation31]. The EST-SSR04 belonging to GRAS family might play important roles in regulating root and shoot development, which can improve strawberry resistance to abiotic stresses [Citation32]. Ethylene responsive factors (ERFs) are important in regulating plant pathogen resistance, abiotic stress tolerance and plant development [Citation33]. The EST-SSR09 of ERF members have greatly enlarged the ERF protein family and revealed more important roles of ERFs in strawberry resistance. Ethylene responsive and MYB transcription factors within the confidence interval for five of the meta-QTLs are recommended as potential targets for partial resistance to white mold in common bean [Citation34]. In our data, the DE-EST-SSR09 (ERF member) and DE-EST-SSR6, 8 and 13 (MYB family) might provide new clues for the mining of QTL loci of resistance to strawberry anthracnose. Therefore, our research provided some new DE-EST-SSRs resources for strawberry anthracnose resistance.
Figure 2. Expression patterns of DE-EST-SSRs after C. fructicola inoculation. (A) Heatmap of differentially expressed EST-SSRs. (B) Venn diagram of differentially expressed EST-SSRs. (C) Expression trends of 13 differentially expressed EST-SSRs at 24, 72, and 96 Hpi.
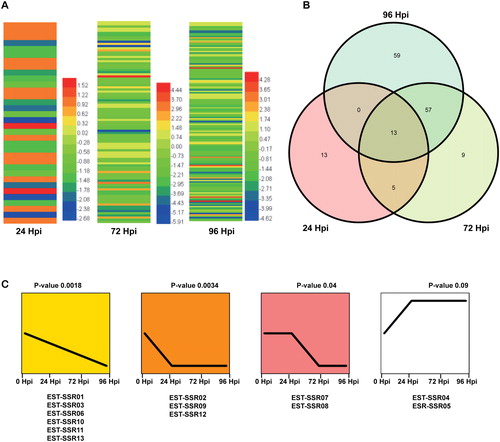
Table 3. Information about 13 differentially expressed EST-SSRs in all three libraries (24, 72 and 96 Hpi).
The allele polymorphisms of DE-Est-SSRs in strawberry varieties and wild species
We detected DE-EST-SSRs polymorphisms by genomic PCRs and genome sequencing. Among the 13 DE-EST-SSRs, six DE-EST-SSRs showed polymorphisms in different cultivars and wild species. In detail, we analyzed the DE-EST-SSRs polymorphisms in the susceptible cultivar ‘Benihoppe’, resistant cultivar ‘Sweet Charlie’ compared with wild species varieties ‘Yellow Wonder’, ‘Hawaii4’ and ‘Schlecht’. Our previous experiment proved that F.vesca is more susceptible than F. nilgerrensis to C. fructicola (unpublished data). Fortunately, our results showed that 6 bases of the EST-SSR04 allele were deleted in resistant ‘Sweet Charlie’ compared with susceptible ‘Benihoppe’. Interestingly, this phenomenon of 6 deleted bases in the EST-SSR04 allele was also consistent in relatively resistant ‘Schlecht’ variety and relatively susceptible ‘Yellow Wonder’ and ‘Hawaii4’ varieties (). There were nucleotide substitution variations within the EST-SSR13 allele between ‘Sweet Charlie’ and ‘Benihoppe’ (). However, regardless of the EST-SSR04 allele or EST-SSR 13 allele, there was very high homology within wild species, compared with ‘Benihoppe’ and ‘Sweet Charlie’ cultivars (). Meanwhile, we also found that other DE-EST-SSRs alleles were also polymorphic in different varieties. For EST-SSR06, there was no ‘GCGGCT’ SSR locus in ‘Benihoppe’, and mutated (GCGGCT)2 SSR allele in ‘Sweet Charlie’ cultivar (). The genotype of the EST-SSR07 locus was (AG)6 in ‘Hawaii4’ and (AG)7 in other varieties (). (GAA)6 SSR locus in EST-SSR08 varied in ‘Schlecht’ and ‘Benihoppe’ (). For EST-SSR09, there was no (ACC)5(TCC)5 SSR allele in ‘Sweet Charlie’, and mutated (ACC)4(TCC)5 SSR allele in ‘Benihoppe’ cultivar (). Thus, the DE-EST-SSRs showed polymorphism in SSR alleles in different varieties and wild species.
Figure 3. Genotyping of EST-SSR04 and EST-SSR13 in different cultivars and species by PCR and PCR products sequencing. The same EST-SSR with differential SSR genotypes but the same amplicon length. The nucleic acid bases of SSR loci are shown in different colors and the nucleotide substitution variations are shown in black.
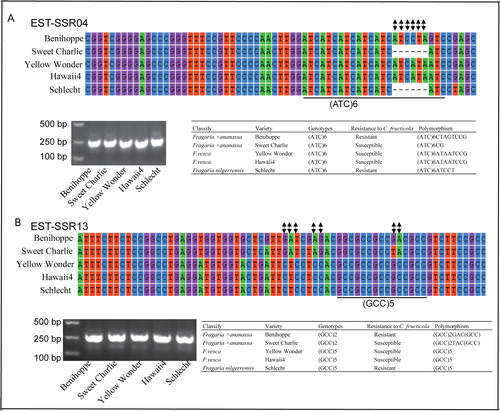
The DE-Est-SSRs related to strawberry anthracnose resistance QTL loci
One of anthracnose resistance QTL loci contains SCAR markers, which are linked to Rca2 anthracnose resistance gene [Citation35]. FaRCg1 is a quantitative trait locus conferring resistance to Colletotrichum crown rot caused by Colletotrichum gloeosporioides in octoploid strawberry [Citation36]. FaRCa1 is a major subgenome-specific locus conferring resistance to Colletotrichum acutatum in strawberry, and is effective against European isolates from pathogenicity group 2 [Citation37]. We found that the two published strawberry anthracnose resistance QTLs of FaRCg1 and FaRCa1 are on LG 6B from 66-72 cM to 55-56 cM on LG 6B (). Furthermore, we found that published strawberry resistance QTLs are largely located in chromosome 1, 2, 4, 5 and 6 in strawberry (Fragaria ×ananassa) for strawberry anthracnose, powdery mildew and Verticillium dahliae resistance [Citation38, Citation39] (). In our data, 4 of the 13 DE-EST-SSRs were located in chromosome 6, which might be one of the main adjacent QTLs for strawberry anthracnose resistance related to FaRCa1 and FaRCg1. Also, the DE-EST-SSR6 correspond to strawberry powdery mildew QTL resistance locus FaRPa2A, with a contiguous distance in chromosome 2 from 25245976-25246956 to 20692727 20699757 in Fragaria ×ananassa genome (). In summary, the candidate DE-EST-SSRs, which were located in the vicinity of strawberry resistance QTLs, might be related to resistance in more than one phenotyping event.
Furthermore, we have analyzed the transcriptome of C. fructicola and strawberry under C. fructicola inoculation [Citation19]. A recent report on strawberry anthracnose pathogen Colletotrichum fructicola showed that the assembly of C. fructicola genome contains 18143 predicted genes with 58.0 Mb [Citation40]. In this research, we have focused on the DE-EST-SSRs after C.fructicola inoculation. These will provide an important cue for understanding the mechanism of fungal pathogenicity and the system of strawberry multilayered defense.
Conclusions
Marker-assisted breeding has not been widely utilized in cultivated strawberry (heterologous octoploid) breeding. In this study, we investigated the EST-SSR in the transcriptome under C. fructicola inoculation using the Illumina sequencing technology. A total of 3019 specific EST-SSRs were identified in cv. Jiuxiang. Also, there were 31, 84 and 129 differentially expressed EST-SSR genes at 24, 72 and 96 Hpi respectively, and 13 candidate DE-EST-SSRs were presented at all the three studied time points. The allele polymorphisms of candidate DE-EST-SSRs were analyzed among the typical resistant and susceptible strawberry varieties and wild species. Four of the 13 DE-EST-SSRs were simultaneously positioned on chromosome 6, and were located in the vicinity of FaRCg1, FaRCa1 and FaRPa2A. The EST-SSRs in our research might be related to resistance loci in strawberry, and provide clues for the development of resistance-related SSR markers in cultivated strawberry.
Supplemental Material
Download MS Excel (1.3 MB)Supplemental Material
Download PDF (716.1 KB)Disclosure statement
No potential conflcit of interest was reported by the author(s).
Additional information
Funding
References
- Jayawardena R, Huang J, Jin B, et al. An account of Colletotrichum species associated with strawberry anthracnose in China based on morphology and molecular data. Mycosphere. 2016;7(8):1147–1191.
- Freeman S, Horowitz S, Sharon A. Pathogenic and nonpathogenic lifestyles in Colletotrichum acutatum from strawberry and other plants. Phytopathology. 2001;91(10):986–992.
- Xiao CL, Mackenzie SJ, Legard DE. Genetic and pathogenic analyses of Colletotrichum gloeosporioides isolates from strawberry and noncultivated hosts. Phytopathology. 2004;94(5):446–453.
- Sreenivasaprasad S, Talhinhas P. Genotypic and phenotypic diversity in Colletotrichum acutatum, a cosmopolitan pathogen causing anthracnose on a wide range of hosts. Mol Plant Pathol. 2005;6(4):361–378.
- Gan P, Nakata N, Suzuki T, et al. Markers to differentiate species of anthracnose fungi identify Colletotrichum fructicola as the predominant virulent species in strawberry plants in Chiba Prefecture of Japan. J Gen Plant Pathol. 2017;83(1):14–22.
- Nam MH, Park MS, Lee HD, et al. Taxonomic re-evaluation of Colletotrichum gloeosporioides isolated from strawberry in Korea. Plant Pathol J. 2013;29(3):317–322. [cited 2020 Apr 22]
- Han Y, Zeng X, Xiang F, et al. Distribution and characteristics of Colletotrichum spp. associated with anthracnose of strawberry in Hubei, China. Plant Dis. 2016;100(5):996–1006.
- Hirayama Y, Asano S, Watanabe K, et al. Control of Colletotrichum fructicola on strawberry with a foliar spray of neutral electrolyzed water through an overhead irrigation system. J Gen Plant Pathol. 2016;82(4):186–189.
- Khan AA, Shih DS. Molecular cloning, characterization, and expression analysis of two class II chitinase genes from the strawberry plant. Plant Sci. 2004;166(3):753–762.
- Casado DA, Encinas VS, Santos B, et al. Analysis of strawberry genes differentially expressed in response to Colletotrichum infection. Physiol Plant. 2006;128(4):633–650.
- Guidarelli M, Carbone F, Mourgues F, et al. Colletotrichum acutatum interactions with unripe and ripe strawberry fruits and differential responses at histological and transcriptional levels. Plant Pathology. 2011;60(4):685–697.
- Fang X, Chen W, Xin Y, et al. Proteomic analysis of strawberry leaves infected with Colletotrichum fragariae. J Proteomics. 2012;75(13):4074–4090.
- Amil-Ruiz F, Garrido-Gala J, Gadea J, et al. Partial activation of SA- and JA-defensive pathways in strawberry upon Colletotrichum acutatum interaction. Front Plant Sci. 2016;7:1036. [cited 2020 Apr 22]
- Wang F, Zhang F, Chen M, et al. Comparative transcriptomics reveals differential gene expression related to Colletotrichum gloeosporioides resistance in the octoploid strawberry. Front Plant Sci. 2017;8:779. [cited 2020 Apr 22]
- Li J, Zhang Q-Y, Gao Q-H, et al. Genome-wide identification and comparative expression analysis of NBS–LRR-encoding genes upon Colletotrichum gloeosporioides infection in two ecotypes of Fragaria vesca. Gene. 2013;527(1):215–227.
- Zhang Q-Y, Zhang L-Q, Song L-L, et al. The different interactions of Colletotrichum gloeosporioides with two strawberry varieties and the involvement of salicylic acid. Hortic Res. 2016;3:16007. [cited 2020 Apr 22]
- Zou X, Guo R, Zhang L, et al. Identification of FaNBS-encoding genes responsive to Colletotrichum fructicola infection in strawberry (Fragaria× ananassa Duchase). Australasian Plant Pathol. 2018;47(5):499–510.
- He C, Duan K, Zhang LQ, et al. Fast quenching the burst of host salicylic acid is common in early strawberry/Colletotrichum fructicola interaction. Phytopathology. 2019;109(4):531–541. [cited 2020 Apr 22]
- Zhang L, Huang X, He C, et al. Novel fungal pathogenicity and leaf defense strategies are revealed by simultaneous transcriptome analysis of Colletotrichum fructicola and strawberry infected by this fungus. Front Plant Sci. 2018;9:434. [cited 2020 Apr 22]
- Uncu AO, Uncu AT. High-throughput simple sequence repeat (SSR) mining saturates the carrot (Daucus carota L.) genome with chromosome-anchored markers. Biotechnology & Biotechnological Equipment. 2020;34(1):1–9.
- You Y, Huang X, Liu H, et al. Leaf transcriptome analysis and development of EST-SSR markers in Arrowhead (Sagittaria trifolia L. var. Sinensis). Tropical Plant Biol. 2020;13(2):189–200.
- Lewers KS, Styan SMN, Hokanson SC, et al. Strawberry GenBank-derived and genomic simple sequence repeat (SSR) markers and their utility with strawberry, blackberry, and red and black raspberry. JASHS. 2005;130(1):102–115.
- Honjo M, Nunome T, Kataoka S, et al. Strawberry cultivar identification based on hypervariable SSR markers. Breed Sci. 2011;61(4):420–425.
- Han B-M. Genetic relationships of strawberry cultivars by SSR analysis. J Plant Gen Res. 2013;14(3):428–433.
- Darwish O, Shahan R, Liu Z, et al. Re-annotation of the woodland strawberry (Fragaria vesca) genome. BMC Genomics. 2015;16(1):29. [cited 2020 Apr 22]
- Liu K, Zhou Z, Wan X, et al. Deep learning network integrated multi-spectral data and interferometric imaging radar altimeter data of Tiangong-2 for land use classification. Proceedings of the Tiangong-2 Remote Sensing Application Conference. 2019. p. 232–240.
- Thiel T, Michalek W, Varshney R, et al. Exploiting EST databases for the development and characterization of gene-derived SSR-markers in barley (Hordeum vulgare L.). Theor Appl Genet. 2003;106(3):411–422.
- Shan T, Rong W, Xu H, et al. The wheat R2R3-MYB transcription factor TaRIM1 participates in resistance response against the pathogen Rhizoctonia cerealis infection through regulating defense genes. Sci Rep. 2016;6:28777. [cited 2020 Apr 22]
- Knoth C, Ringler J, Dangl JL, et al. Arabidopsis WRKY70 is required for full RPP4-mediated disease resistance and basal defense against Hyaloperonospora parasitica. Mol Plant Microbe Interact. 2007;20(2):120–128.
- Kage U, Yogendra KN, Kushalappa AC. TaWRKY70 transcription factor in wheat QTL-2DL regulates downstream metabolite biosynthetic genes to resist Fusarium graminearum infection spread within spike. Sci Rep. 2017;7:42596. [cited 2020 Apr 22]
- Chen LH, Tsai HC, Yu PL, et al. A major facilitator superfamily transporter-mediated resistance to oxidative stress and fungicides requires Yap1, Skn7, and MAP kinases in the citrus fungal Pathogen Alternaria alternata. PLoS One. 2017;12(1):e0169103. [cited 2020 Apr 22]
- Zhang B, Liu J, Yang ZE, et al. Genome-wide analysis of GRAS transcription factor gene family in Gossypium hirsutum L. BMC Genomics. 2018;19:348 https://doi.org/10.1186/s12864-018-4722-x. [cited 2020 Apr 22]
- Zhang H, Zhang D, Chen J, et al. Tomato stress-responsive factor TSRF1 interacts with ethylene responsive element GCC box and regulates pathogen resistance toRalstonia solanacearum. Plant Mol Biol. 2004;55(6):825–834.
- Vasconcellos RCC, Blessing OO, Alvaro S, et al. Meta-QTL for resistance to white mold in common bean. Plos One. 2017;12(2):e0171685. [cited 2020 Apr 22]
- Lerceteau K, Hler E, Guérin G, et al. Identification of SCAR markers linked to Rca2 anthracnose resistance gene and their assessment in strawberry germplasm. Theor Appl Genet. 2005;111(5):862–870.
- Anciro A, Mangandi J, Verma S, et al. FaRCg1: a quantitative trait locus conferring resistance to Colletotrichum crown rot caused by Colletotrichum gloeosporioides in octoploid strawberry. Theor Appl Genet. 2018;131(10):2167–2177.
- Salinas N, Verma S, Peres N, et al. FaRCa1: a major subgenome-specific locus conferring resistance to Colletotrichum acutatum in strawberry. Theor Appl Genet. 2019;132(4):1109–1120.
- Antanaviciute L, Urbanovski N, Harrison N, et al. Mapping QTL associated with verticillium dahliae resistance in the cultivated strawberry (Fragaria × ananassa). Hortic Res. 2015;2:15009. [cited 2020 Apr 22]
- Cockerton HM, Vickerstaff RJ, Karlström A, et al. Identification of powdery mildew resistance QTL in strawberry ((Fragaria ×ananassa). Theor Appl Genet. 2018;131(9):1995–2007.
- Armitage AD, Nellist C,a, Bates HJ, et al. Draft genome sequence of the strawberry anthracnose pathogen Colletotrichum fructicola. Microbiol Resource Announcement. 2020;9(12):e01598–19. [cited 2020 Apr 22]