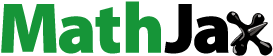
Abstract
The aim of this study was to investigate the extraction of antibacterial substances from Galla chinensis (ASGC) that act against the aquatic pathogen Vibrio harveyi, to identify the chemical composition and explore the mechanism of action. The optimal extraction process was as follows: anhydrous methanol, liquid/material ratio (mL/g) 26:1, extraction temperature 98 °C and extraction time 157 min. The diameter of the inhibition zones (21.31 ± 0.51 mm) under the optimal conditions agreed with the predicted value by experimental rechecking. The yield of ASGC was 39.39% ± 0.69%, and two compounds including gallic acid (GA) and penta-O-galloyl-β-d-glucose (5GG) were identified in ASGC. The minimal inhibitory concentration (MIC) values of ASGC and GA were 0.50 and 0.25 mg/mL, respectively. Meanwhile, 5GG and other chemical constituents did not exert antibacterial activities against V. harveyi (MICs > 1 mg/mL). Further investigation of the mechanisms of action of ASGC and GA against V. harveyi revealed their strong destructive effects on the growth of bacterial cells, the integrity and permeability of the cell membrane, and morphological alterations. The report provides a favorable prospect of GA as a potential control agent against V. harveyi in aquaculture.
Introduction
Outbreaks and epidemics of animal diseases in aquaculture, which have increasingly become a major factor affecting the aquaculture industry, have occurred with the continuous development of the world’s aquaculture industry, especially the promotion of high-density farming technology [Citation1]. Fulminant vibriosis caused by Vibrio harveyi is one of the most serious diseases that endanger aquaculture animals. This pathogen can infect many kinds of fish, shrimp and shellfish and cause a large number of deaths, resulting in huge economic losses to the aquaculture industry [Citation2–4]. At present, antibiotics are primarily used in preventing and treating aquatic animal diseases caused by Vibrio spp. However, the long-term application of antibiotics will cause the emergence of resistant strains, which cause not only difficulties in the treatment of aquatic animal diseases, but also certain harm to aquatic products, the environment and the human body [Citation5,Citation6]. Therefore, the demand for the prevention and control of V. harveyi in marine aquaculture is increasing day by day.
Chinese medicinal herbs have the advantages of abundant resources, few residues, small side effects and high safety. Given these characteristics, they have become the focus of current research on aquatic drugs [Citation7–9]. Galla chinensis is widely used in China and refers to the abnormal growing galls on the leaf tissue of Anacardiaceae plants such as Rhus chinensis Mill., R. potaninii Maxim. and R. punjabensis Stew. var. sinica (Diels) Rehd. et Wils., which are mainly formed by the parasitism of Melaphis chinensis (Bell) Baker [Citation10]. G. chinensis is rich in gallotannins and gallic acid (GA), which contribute to its antimicrobial, antiviral, antitumor, antioxidant, anti-inflammatory, liver-protective and antithrombin activities [Citation11,Citation12]. G. chinensis has been approved by the Ministry of Agriculture of China for the prevention and treatment of aquatic animal diseases. However, its antipathogenic substances remain unidentified and the overall medical efficacy is not stable. Therefore, the purity of the antibacterial substances of G. chinensis (ASGC) should be improved, and the antibacterial compounds should be identified.
In the present study, single-factor experiments, Box–Behnken design (BBD) and response surface methodology (RSM) were first applied to optimize the extraction process for ASGC active against V. harveyi. Then, the main antibacterial chemical constituents of ASGC were identified by using high-performance liquid chromatography (HPLC) and the Oxford cup method. Finally, the mechanisms of action of ASGC and GA toward V. harveyi were explored by using the bacterial growth curve, ultraviolet absorption, electric conductivity, and scanning electron microscopy.
Materials and methods
Materials and chemicals
G. chinensis was obtained from Anhui Chinese Medicinal Factory (Baozhou, China). Vibrio harveyi 1.8690 was obtained from China General Microbiological Culture Collection Center (CGMCC, Beijing, China). MH broth medium was obtained from Aoboxing Biotech Co., Ltd (Beijing, China). Gallic acid was purchased from Hefei Bomei Biotech Co., Ltd (Hefei, China). Penta-O-galloyl-β-d-glucose (5GG) was obtained from Chengdu PureChem-Standard Co., Ltd. (Chengdu, China). Methanol and other analytical grade chemicals were obtained from Sinapharm Chemical Reagent Co., Ltd. (Shanghai, China).
Single-factor experiments
The raw materials of G. chinensis were air dried, crushed by a pulverizer and passed through a 40-mesh sieve. One gram of G. chinensis fine powder was precisely weighed and used for each experiment. First, the influence of extraction solvent (distilled water, 50% methanol, anhydrous methanol, 50% ethanol, anhydrous ethanol) on the antibacterial activities of the extracts was investigated. Twenty milliliters of different extraction solvents and 1 g of the fine powder were added into a 100 mL round-bottom flask. The extraction was performed in a HH-4 thermostatic water boiler (Jiangnan Co., China) at 90 °C for 120 min.
Second, the influence of different concentrations of methanol on the antibacterial activities of the extracts was investigated. Twenty milliliters of different concentrations of methanol (60%–100%) and 1 g of the fine powder were placed into a 100 mL round-bottom flask. The extraction was conducted at 90 °C for 120 min.
Third, the influence of extraction temperature on the antibacterial activities of the extracts was investigated. Twenty milliliters of methanol and 1 g of the fine powder were placed into a 100 mL round-bottom flask, and the extraction was conducted at different temperatures (i.e. 60 °C, 70 °C, 80 °C, 90 °C and 100 °C) for 120 min.
Fourth, the effect of extraction time on the antibacterial activities of the extracts was investigated. Twenty milliliters of methanol and 1 g of the fine powder were placed into a 100 mL round-bottom flask, and the extraction was conducted at 90 °C for different times (i.e. 60, 90, 120, 150 and 180 min).
Last, the influence of liquid/material ratio on the antibacterial activities of the extracts was investigated. Different volumes (i.e. 10, 20, 30, 40, 50 mL) of methanol were separately added into a 100 mL round-bottom flask with 1 g of the fine powder. The extraction was conducted at 90 °C for 150 min.
After the above steps were conducted, the extracts were centrifuged at 1500 g for 10 min and then filtered under reduced pressure. The filtrates were diluted or concentrated to 20 mL and used to determine the anti-V. harveyi activity.
Box–Behnken design (BBD)
The extraction conditions were optimized by BBD. Design Expert 7.0.0 (Stat-Ease, Minneapolis, USA) was applied to analyze the experimental data and build the model [Citation4]. The BBD consists of 17 test points, 12 of which are factorial points and 5 are repeats of the center point. The extraction temperature (X1), extraction time (X2) and liquid/material ratio (X3) were selected as the independent variables, whereas the diameters of the inhibition zone of the extracts against V. harveyi were the response variable (Y, mm). shows the range and levels of the independent variables and the values of the response variable. By using the Design Expert 7.0.0 software, the aim is to obtain the maximum diameters of the inhibition zone of the extracts against V. harveyi. The following second-order polynomial equation was applied to evaluate the pattern of the system:
(1)
(1)
where Y represents the estimated response; β0, βi, βii and βij are regression coefficients in the intercept, linear, quadratic terms; and Xi and Xj are the independent factors.
Table 1. Coded (actual) values of the independent factors and response values of BBD.
Identification and preparation of chemical constituents in ASGC by HPLC
Two reference compounds (GA and 5GG) were first adopted to identify the main chemical compositions of ASGC by comparing the chromatograms of reference mixtures and ASGC using HPLC [Citation13]. Ultimate 3000 HPLC (Thermo Fisher Scientific, USA) equipped with a Shim-Pack CLC-ODS column (10.0 mm × 250 mm, 5 μm, 1.5 mL/min) was used and the detection wavelength was set at 280 nm. Water, 0.1% phosphoric acid (solvent A) and methanol (solvent B) were used as mobile phases. The gradient elution program was as follows (v/v): 0 min 5% B, 5 min 15% B, 35 min 40% B, 45 min 45% B and 50 min 95% B, followed by 10 min of re-equilibration. The other chemical constituents included in ASGC were subjected to HPLC using the above method.
Oxford cup method
The Oxford cup method was adopted to determine the antibacterial activities of the extracts against V. harveyi [Citation8]. Twenty milliliters of beef extract peptone medium (beef extract 0.3%, peptone 1%, agar 1.5%, and aged seawater) was poured into the plates and solidified. Approximately 100 μL of V. harveyi 1.8690 suspension (1 × 106 cfu/mL) was evenly coated on the plate. Thereafter, the Oxford cups were placed on the medium, and 200 µL of each extract was added into the cups. Then the plates were cultivated at 37 °C for 12 h, and the diameters of the inhibition zone were measured. The average value of the diameters of the inhibition zone (mm, n = 3) was treated as the anti-V. harveyi activity of the extracts.
The minimal inhibitory concentration (MIC) of ASGC and its 10 chemical components against V. harveyi 1.8690 were determined through the above method. ASGC and its 10 chemical components were dissolved in methanol and diluted by serial twofold dilution. The lowest concentration at which the compound produced an inhibition zone was defined as the MIC value [Citation14].
Serial dilution method
The MIC values of ASGC and GA against V. harveyi 1.8690 were determined by using the serial dilution method as shown below. ASGC and GA were dissolved in methanol and diluted by serial twofold dilution. Approximately 1.0 mL of V. harveyi 1.8690 suspension (1 × 106 cfu/mL) and 50 μL of different concentrations of sample solutions were added into the test tube (20.5 cm × 2.5 cm) with 4 mL of MH broth medium. Meanwhile, 50 μL of methanol was used as the negative control. The tubes were mixed well and cultured at 37 °C and 160 rpm for 24 h. Thereafter, the MIC was determined according to the bacterial growth state and defined as the lowest compound concentration without bacterial growth [Citation15].
Bacterial growth curve
The effects of ASGC and GA on the growth of V. harveyi 1.8690 were assessed as previously described [Citation15]. ASGC and GA were dissolved in methanol and prepared at 100-fold MIC concentration. Then 200 μL of ASGC or GA solutions was added into a 50 mL Erlenmeyer flask with 20 mL of MH broth medium and 200 μL of methanol was used as a negative control. After the solutions were mixed well, 200 μL of V. harveyi suspensions (1 × 106 cfu/mL) was added into each flask separately. The flasks were incubated at 37 °C and 160 rpm for 24 h. During the cultivation process, 200 μL of bacterial cultures was sampled at intervals of 6 h and added to the well of a 96-well microtiter plate for the determination of absorbance at 620 nm. The experiment was set up with three repetitions. The average of the absorbance and culture time were used as the ordinate and abscissa, respectively, for drawing the bacterial growth curve.
Integrity and permeability of the cell membrane
Bio-endogenous UV absorptive materials such as proteins, DNA and RNA would leak when the bacterial cell membrane was destroyed. The integrity of the cell membrane can be analyzed by determining the absorbance at 260 nm of the bacterial supernatants [Citation16]. Approximately 400 μL of the 100-fold MIC concentration of ASGC and GA dissolved in methanol and 400 μL of methanol were added to 100 mL Erlenmeyer flasks with 40 mL of MH broth medium. After the solutions were mixed well, 400 μL of V. harveyi suspensions (1 × 106 cfu/mL) was added into each Erlenmeyer flask separately. The flasks were incubated at 37 °C and 160 rpm; following culture time of 0, 1, 2, 3 and 4 h, 4.0 mL of bacterial samples were gathered and centrifuged at 1500 g for 10 min. Then, the supernatants were obtained, and the absorbance at 260 nm was determined. The experiment was set up with three repetitions. The average absorbance and culture time were used as the ordinate and abscissa, respectively, to evaluate the effects of ASGC and GA on the integrity of the cell membrane of V. harveyi.
The permeability of the cell membrane was evaluated by determining the electric conductivity of the bacterial suspension of V. harveyi 1.8690 after treatment with ASGC and GA. Approximately 1.0 mL of the supernatants after determining the absorbance at 260 nm and 4 mL of distilled water were added in a 20-mL beaker. Then the electric conductivity was determined using a pen conductivity meter. All data were reported as the conductivity values at each time interval.
Scanning electron microscopy
The effects of ASGC and GA on the morphology of V. harveyi 1.8690 were determined by scanning electron microscopy [Citation17]. Approximately 50 μL of the 100-fold MIC concentration of ASGC and GA dissolved in methanol and 50 μL of methanol were added into a 10 mL centrifuge tube with 5 mL of MH broth medium. After the solutions were mixed well, 50 μL of V. harveyi suspension (1 × 106 cfu/mL) was added into each tube separately. The tubes were cultivated at 37 °C for 3 h. Then the cultures were centrifuged at 1500 g for 5 min to obtain the thallus. The thallus was slowly washed thrice with 50 mmol/L phosphate buffer solution (pH 7.0). Thereafter, 1 mL of glutaric dialdehyde (2.5%) was added and placed at 4 °C overnight. The mixtures were centrifuged at 1500 g for 5 min. Then the collected thallus was mounted over the coverslip and dehydrated with a graded ethanol series. The sample was dried at a critical point with CO2, sputtered with a thin layer of gold and then observed using a scanning electron microscope (JSM-6390LA, Hitachi, Japan).
Statistical analysis
All experiments were performed in triplicate and average values are shown. Analysis of variance was performed by ANOVA procedure. p < 0.05 and p < 0.01 were regarded as significant and very significant, respectively.
Results and discussion
Optimization of the extraction parameters of ASGC
Before the optimization experiment, the extraction solvent, solvent concentration, extraction temperature, extraction time and liquid/material ratio were investigated as the important factors that independently affected the antibacterial activity against V. harveyi of the extracts.
reveals the effects of five different extraction solvents on the diameters of the inhibition zones of the extracts. These results indicated that methanol was the solvent that had the best effect. Then the influence of different methanol concentrations on the antibacterial activity of the extracts was investigated (). The diameters of the inhibition zones increased as the methanol concentration increased, indicating that ASGC was mainly hydrophobic. Therefore, anhydrous methanol was chosen as the extraction solvent.
Figure 1. Effect of different variables on the antibacterial activity of the extracts against V. harveyi: Extraction solvent (a), methanol concentration (b), extraction temperature (c), extraction time (d), liquid/material ratio (e).
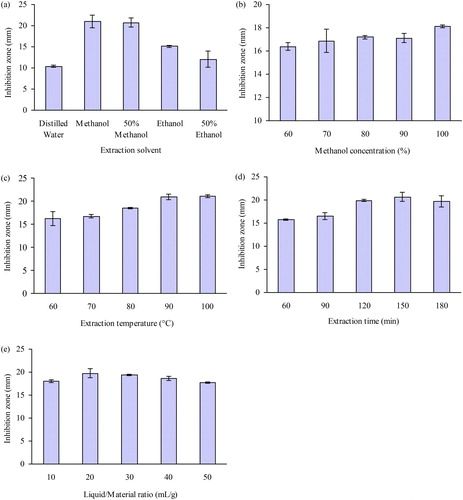
The diameters of the inhibition zones significantly increased when the extraction temperature was raised from 60 to 90 °C (). Increasing the extraction temperature can lower the viscosity, and increase the mass transfer coefficient and solubility of the active substances, which may facilitate the extraction of ASGC [Citation8]. shows a relatively rapid elevation in the diameters of the inhibition zone when the extraction time ranged from 60 to 150 min. Thereafter, the diameters of the inhibition zones no longer significantly increased. As can be seen in , the diameters of the inhibition zone significantly increased when the liquid/material ratio was changed from 10:1 to 20:1 mL/g. However, the diameters of the inhibition zone no longer increased when the ratio of the methanol solvent increased further. Thus, the extraction temperature of 90 °C, extraction time of 150 min, and liquid/material ratio of 20:1 mL/g were selected as the center points of the three independent variables in the RSM.
The extraction temperature (X1), extraction time (X2) and liquid/material ratio (X3) were selected for further optimization by BBD (). The application of RSM can provide an empirical relationship between the response variable and the test variables based on the parameter estimates. Multiple regression analysis of the experimental data was used to build the equation for the diameters of the inhibition zone as follows:
(2)
(2)
The results of the analysis of variance (ANOVA) are shown in . The determination coefficient value of EquationEquation (2)(2)
(2) , R2 (0.9045), is very close to 1, indicating that the regression model well defines the true behavior of the system. The p value (0.0077) indicates that the goodness-of-fit of the model is very good. The value of lack of fit (0.5530) showed no significant difference. Furthermore, the linear effects of extraction temperature and liquid/material ratio were very significant (p < 0.01). The linear effect of extraction time and the quadratic effect of extraction time were also significant (p < 0.05).
Table 2. ANOVA of the effects of temperature, time and liquid/material ratio on the diameters of the inhibition zone of the extracts using the quadratic response surface model.
The predicted maximum diameter of the inhibition zone was 21.14 mm under the following conditions: temperature X1 = 98 °C, extraction time X2 = 157 min and L/M ratio X3 = 26 mL/g by applying the regression analysis to EquationEquation (2)(2)
(2) . The above optimized conditions were used for the verification test and the average value of the inhibition zone was 21.31 ± 0.51 mm, which was not significantly different from the predicted value and revealed the feasibility of RSM for the optimization of ASGC extraction. Next, the solid ASGC was obtained by evaporating and drying the extracts under reduced pressure, and the yield was 39.39% ± 0.69% (n = 3). Solid ASGC was used for the upcoming determination of chemical compositions.
Identification and antibacterial activities of chemical components in ASGC
The HPLC chromatogram indicated that ASGC contains more than 10 chemical components including GA and 5GG (). The 10 chemical components in ASGC with retention times (tR) of 18.837, 23.047, 26.330, 27.660, 29.053, 31.953, 33.703, 35.080, 36.680 and 39.800 min were prepared, and their antibacterial activities against V. harveyi 1.8690 were measured using the Oxford cup method. The results showed that the MIC values of ASGC and GA were 0.50 and 0.25 mg/mL, respectively. However, the MIC values of other chemical components were more than 1 mg/mL, which indicated that GA is the main antibacterial compound of ASGC. The content of GA in ASGC was determined by linear regression equations obtained from the relationships of the peak area (mAu) and the concentration of GA as 5.28% ± 0.18%. The MIC values of ASGC and GA against V. harveyi determined by serial dilution assay were 0.50 and 0.25 mg/mL, respectively.
Mechanisms of action of ASGC and GA against V. harveyi
The effects of ASGC and GA on the growth of V. harveyi 1.8690 are shown in . As can be seen from the figure, the strain in the control group grew normally, and reached the logarithmic growth period in 18 h. However, the ASGC and GA groups basically did not grow in 18 h, which indicated that ASGC and GA can significantly inhibit the growth of bacteria at onefold MIC concentration. Thereafter, V. harveyi treated with onefold MIC of GA began to grow rapidly, which showed that the inhibition of V. harveyi by GA gradually decreases with the consumption of GA. The strain in the ASGC group still did not grow, indicating that gallotannins were hydrolyzed to GA and can provide sustained bacteriostatic effects.
The integrity of the cell membrane was measured by evaluating the effects of ASGC and GA on the release of 260 nm absorbing substances of V. harveyi (). There was almost no change in the absorbance value at 260 nm in the control group. With the prolongation of time, an obvious increase in the absorbance value at 260 nm was observed in the bacterial culture treated with onefold MIC of ASGC and GA. The effect of the ASGC group was better than that of the GA group, indicating a more visible leakage of the intracellular bio-macromolecules to the outer solution by cellular leakage. shows the effects of ASGC and GA on the permeability of the cell membrane of V. harveyi 1.8690 by assessing the electric conductivity of the bacterial suspension. The electric conductivity of the ASGC and GA treated groups were higher than that of the control group, indicating that ASGC and GA can destroy the permeability of the cell membrane.
Scanning electron microscopy was adopted to determine the effects of ASGC and GA on the cellular ultrastructure of V. harveyi 1.8690 (). The untreated V. harveyi had a short rod shape with elliptical ends and good bacterial growth. After treatment with ASGC and GA at onefold MIC concentration for 3 h, the morphology of the cells was abnormal, accompanied by distortion and deformation, partial cell rupture, and cytoplasmic outflow, resulting in blurred cell edges. The destruction effect of the ASGC-treated group on the cells was stronger than that of the GA-treated group, which was also consistent with the results measured by the above methods.
The problems of pathogen resistance and drug residues in aquatic products caused by the long-term application and abuse of antibiotics in the aquaculture industry are becoming more and more serious, and research to find antibiotic alternatives has become a highly interesting concern. At present, the development of antibiotic substitutes in aquaculture mainly focuses on probiotics [Citation18], microbial metabolites [Citation4,Citation19], plants and their extracts [Citation20,Citation21].
Chinese medicinal herbs have been successfully used in the prevention and treatment of human diseases for thousands of years. Regarding this view, crude powders, extracts or mixtures of medicinal plants have good prospects for the prevention and treatment of aquatic animal diseases and have been investigated [Citation21]. However, there are many difficulties in practical application, such as unstable therapeutic effect and unknown effective ingredients. Therefore, improving the purity of active substances of medicinal plants against aquatic pathogens and identifying their active compounds are helpful for the research and development of antibacterial agents. We have studied the extraction process of active substances of Rhubarb against V. harveyi and identified aloe emodin and rhein as the main antibacterial compounds [Citation8]. Similarly, in the present study, we optimized the extraction process of ASGC by using single-factor experiments and Box–Behnken design. Further, GA (3,4,5-trihydroxybenzoic acid) was identified as the antibacterial active molecule of ASGC.
GA is an important natural bioactive phenolic compound that is widely found in medicinal plant species such as Rhus spp., Quercus spp. and Punica spp. [Citation22]. GA has been reported to have antibacterial [Citation23,Citation24], antifungal [Citation25], antioxidant [Citation26], anticancer [Citation27], anti-inflammatory [Citation28], antiviral [Citation29] and antimutation properties [Citation30]. Although several studies have revealed that GA exerts antimicrobial effects toward human pathogens, few studies have reported on the antimicrobial action against aquatic pathogens. This study is the first to demonstrate that the anti-V. harveyi effect of GA from G. chinensis may be utilized for its potent application in preventing and controlling aquatic animal disease. To further explore the feasibility of GA as a biocontrol agent in aquaculture practice, more in-depth research is needed. It is necessary to compare the effects of GA and other antibiotics routinely used in aquaculture on the fish growth, health and resistance to pathogenic bacteria.
Conclusion
Single-factor experiments, BBD and RSM were applied to optimize the extraction process of ASGC. The optimized process was as follows: anhydrous methanol, liquid/material ratio (mL/g) 26:1, extraction temperature 98 °C and extraction time 157 min, and the yield of solid ASGC was 39.39% ± 0.69%. GA was identified as the main antibacterial component of ASGC and the content of GA in ASGC was 5.28% ± 0.18%. The MIC values of ASGC and GA were 0.50 and 0.25 mg/mL, respectively. The mechanism of action indicated that ASGC and GA exhibited anti-V. harveyi activities by disrupting the integrity and permeability of the cell membrane, resulting in the leakage of intracellular biomacromolecules and changes in cell morphology, which provides the basis for the development and application of GA in the prevention and control of aquatic animal diseases.
Disclosure statement
No potential conflict of interest was reported by the authors.
Additional information
Funding
References
- Guo L, Wang C, Zhu W, et al. Bioassay-guided fractionation and identification of active substances from the fungus Aspergillus tubingensis against Vibrio anguillarum. Biotechnol Equip. 2016;30(3):602–606.
- Morya VK, Choi W, Kim EK. Isolation and characterization of Pseudoalteromonas sp. from fermented Korean food, as an antagonist to Vibrio harveyi. Appl Microbiol Biotechnol. 2014;98(3):1389–1395.
- Thompson J, Gregory S, Plummer S, et al. An in vitro and in vivo assessment of the potential of Vibrio spp. as probiotics for the Pacific white shrimp, Litopenaeus vannamei. J Appl Microbiol. 2010;109(4):1177–1187.
- Guo L, Wang C. Optimized production and isolation of antibacterial agent from marine Aspergillus flavipes against Vibrio harveyi. 3 Biotech. 2017;7(6):383.
- Harikrishnan R, Balasundaram C, Heo MS. Effect of probiotics enriched diet on Paralichthys olivaceus infected with lymphocystis disease virus (LCDV). Fish Shellfish Immunol. 2010; 29(5):868–874.
- Cao H, He S, Wei R, et al. Bacillus amyloliquefaciens G1: a potential antagonistic bacterium against eel-pathogenic Aeromonas hydrophila. Evid Based Complem. Alternat Med. 2011;2011:824104.
- Abarike ED, Jian J, Tang J, et al. Traditional Chinese medicine enhances growth, immune response, and resistance to Streptococcus agalactiae in Nile tilapia. J Aquat Anim Health. 2019;31(1):46–55.
- Guo L, Guo J, Xu F. Optimized extraction process and identification of antibacterial substances from Rhubarb against aquatic pathogenic Vibrio harveyi. 3 Biotech. 2017;7(6):377.
- Wang E, Chen X, Wang K, et al. Plant polysaccharides used as immunostimulants enhance innate immune response and disease resistance against Aeromonas hydrophila infection in fish. Fish Shellfish Immunol. 2016;59:196–202.
- Tian F, Li B, Ji B, et al. Identification and structure-activity relationship of gallotannins separated from Galla chinensis. LWT Food Sci Technol. 2009;42(7):1289–1295.
- Huang XL, Liu MD, Li JY, et al. Chemical composition of Galla chinensis extract and the effect of its main component(s) on the prevention of enamel demineralization in vitro. Int J Oral Sci. 2012;4(3):146–151.
- Jang SE, Hyam SR, Jeong JJ, et al. Penta-O-galloyl-β-D-glucose ameliorates inflammation by inhibiting MyD88/NF-κB and MyD88/MAPK signalling pathways. Br J Pharmacol. 2013;170(5):1078–1091.
- Guo L, Wang L, Li X, et al. Enhanced production of questin by marine-derived Aspergillus flavipes HN4-13. 3 Biotech. 2020;10(2):54.
- Guo L, Cao X, Yang S, et al. Characterization, solubility and antibacterial activity of inclusion complex of questin with hydroxypropyl-β-cyclodextrin. 3 Biotech. 2019;9(4):123.
- Guo L, Zhang F, Wang X, et al. Antibacterial activity and action mechanism of questin from marine Aspergillus flavipes HN4-13 against aquatic pathogen Vibrio harveyi. 3 Biotech. 2019;9(1):14.
- Patra JK, Baek KH. Antibacterial activity and action mechanism of the essential oil from Enteromorpha linza L. against foodborne pathogenic bacteria. Molecules 2016;21(3):388.
- Qu L, She P, Wang Y, et al. Effects of norspermidine on Pseudomonas aeruginosa biofilm formation and eradication. Microbiologyopen 2016;5(3):402–412.
- Hai NV. The use of probiotics in aquaculture. J Appl Microbiol. 2015;119(4):917–935.
- Xu HM, Rong YJ, Zhao MX, et al. Antibacterial activity of the lipopetides produced by Bacillus amyloliquefaciens M1 against multidrug-resistant Vibrio spp. isolated from diseased marine animals. Appl Microbiol Biotechnol. 2014;98(1):127–136.
- Turker H, Yildirim AB. Screening for antibacterial activity of some Turkish plants against fish pathogens: a possible alternative in the treatment of bacterial infections. Biotechnol Equip. 2015;29(2):281–288.
- Awad E, Awaad A. Role of medicinal plants on growth performance and immune status in fish. Fish Shellfish Immunol. 2017;67:40–54.
- Kahkeshani N, Farzaei F, Fotouhi M, et al. Pharmacological effects of gallic acid in health and diseases: a mechanistic review. Iran J Basic Med Sci. 2019;22(3):225–237.
- Borges A, Ferreira C, Saavedra MJ, et al. Antibacterial activity and mode of action of ferulic and gallic acids against pathogenic bacteria. Microb Drug Resist. 2013;19(4):256–265.
- Rattanata N, Klaynongsruang S, Leelayuwat C, et al. Gallic acid conjugated with gold nanoparticles: antibacterial activity and mechanism of action on foodborne pathogens. IJN. 2016;11:3347–3356.
- Li ZJ, Liu M, Dawuti G, et al. Antifungal activity of gallic acid in vitro and in vivo. Phytother Res. 2017;31(7):1039–1045.
- Minh TN, Xuan TD, Tran HD, et al. Isolation and purification of bioactive compounds from the stem bark of Jatropha podagrica. Molecules 2019;24(5):889.
- Gu R, Zhang M, Meng H, et al. Gallic acid targets acute myeloid leukemia via Akt/mTOR-dependent mitochondrial respiration inhibition. Biomed Pharmacother. 2018;105:491–497.
- Khan BA, Mahmood T, Menaa F, et al. New perspectives on the efficacy of gallic acid in cosmetics & nanocosmeceuticals. Curr Pharm Des. 2018;24(43):5181–5187.
- You HL, Huang CC, Chen CJ, et al. Anti-pandemic influenza A (H1N1) virus potential of catechin and gallic acid. J Chin Med Assoc. 2018;81(5):458–468.
- Wang BS, Tang CH, Chiu CK, et al. Inhibitory effects of water extract from longan twigs on mutation and nitric oxide production. Food Chem. 2012;135(2):440–445.