Abstract
To investigate the expression and role of nicotinic acetylcholine α7 subunit (nAChRα7) in the hippocampus of rats with Parkinson's disease (PD) and Parkinson's disease dementia (PDD). PD model was established with 6-hydroxydopa. Then, methyllycaconitine was further used to prepare a PDD model. The behavioral indexes and malondialdehyde (MDA) and superoxide dismutase (SOD) levels were measured. The mRNA level and protein level of nAChRα7 in hippocampus was detected by reverse transcription quantitative polymerase chain reaction (RT-qPCR) and Western-blot, respectively. The α7nAchR and NeuN protein was detected by immunofluorescence. Compared with the PD model group, the escape latency of the PDD model group was significantly prolonged while the times of crossing platforms was significantly reduced (p < 0.05). The SOD level in the PDD and PD group decreased as compared with the control group (p < 0.05), while the MDA content increased (p < 0.05). The mRNA level and protein expression of nAChRα7 in the PDD and PD model group was significantly lower than that in the control group (p < 0.05). There was a significant difference in the nAChRα7 protein level between the PDD and PD model group (p < 0.05). Together, the nAChRα7 receptor level was significantly decreased in the PDD model group, indicating that the decrease in the nAChRα7 receptor levels is a possible mechanism of the pathogenesis of PDD.
Introduction
Parkinson's disease (PD) is the second most common degenerative disease of the nervous system. Middle-aged and elderly people are the main targets, and the harm is second only to cancer and cardiovascular diseases. Current research shows that Parkinson's disease has a clear trend of rejuvenation [Citation1]. The pathological manifestations of PD are mainly the degeneration and necrosis of dopaminergic neurons in the midbrain, accompanied by the accumulation of Lewy bodies in the cytoplasm of the remaining neurons, which eventually leads to a decrease in the secretion of dopamine in the nigrostriatal system [Citation2–5]. At the early stage, the motor system is often affected and the clinical features include resting tremor, facial expression reduction, slow movement and unstable position. With disease progression, the non-motor symptoms become obvious, including impaired cognitive dysfunction and Parkinson's disease dementia (PDD).
The nicotinic acetylcholine receptor (nAChR) is distributed in both nerve tissue (central and peripheral) and muscle tissue, and is classified into a neuronal type and a muscle type. The latter is present at the neural junction, and the former is mainly present in the nerve tissue and contains 8 alpha subunits and 3 beta subunits. The neuronal nicotinic acetylcholine receptor α7 (nAChRα7) formed by the α7 homopentamer is widely distributed in the brain and is highly expressed in the hippocampus [Citation6]. It is closely related to learning, memory, exercise and other activities, and participates in neuron survival, neurotransmitter release and synaptic plasticity, which can directly affect memory function and recognition ability [Citation7]. nAChRα7 plays a key role in neurology, neuropsychiatry and neurodegenerative diseases [Citation8]. Therefore, nAChRα7 was investigated in this study.
In China, the incidence of PDD is on the rise. A number of patients with PD who have no dementia symptoms have varying degrees of cognitive impairment [Citation8]. Many patients with PD have developed cognitive problems just after diagnosis or early onset [Citation9]. This causes reduced quality of life and psychological damage to the patient, and also increases the burden on the family and society, and even becomes one of the important factors that lead to the death of the patient during long-term treatment. The neuropathological and biochemical basis of PDD is still unclear. Early diagnosis relies mainly on clinical manifestations, but these manifestations are easily neglected or misjudged in clinical practice with other similar diseases. Therefore, further research on PDD is particularly important.
In this study, 6-hydroxydopa and methyllycaconitine was used to establish the PD and PDD model [Citation10,Citation11], and the behavioral indexes of each experimental group were observed. The expression of nAChRα7 in the hippocampus was detected by RT-qPCR and Western-blot. The expression of α7nAchR/NeuN in the body was detected by immunofluorescence double labeling. The obtained results may provide a theoretical basis for further study on the pathogenesis of PDD.
Materials and methods
Ethics statement
All animal experiments were conducted according to the ethical guidelines of Xinjiang Medical University and were approved by Xinjiang Medical University. All efforts were made to minimize animal suffering.
Animals
A total of 70 male Sprague-Dawley rats (SPF; 8 weeks; 300 ± 20 g body weight) were purchased from the Animal Center of Xinjiang Medical University. The rats were kept in the specific-pathogen-free (SPF) laboratory under an artificial circadian rhythm (12 h each day and night, 8:00 to 20:00 light; 20:00 to 8:00 the next day, dark) with room temperature of 18–25 °C.
Model establishment and animal grouping
The PD model was established in 50 male rats as described previously [Citation12]. In the first week, the rats were injected with 6-hydroxydopa solution at the right side of intracerebral substantia nigra pars compacta and midbrain ventral tegmental area. From week 2 to week 8, 0.5 mg/kg of apomorphine was injected intraperitoneally every week. The standard for successful PD rat model preparation was as follows [Citation3]: after injection of apomorphine, the body showed a constant head-to-tail rotation to the left side (the normal side) with an average speed of >7 r/min in 30 min, and a total rotational speed > 210 r.
Rats with PD (n = 25) were randomly selected to establish the PPD model as described previously [Citation13]. PD rats were anesthetized by intraperitoneal injection of 10% chloral hydrate (300 mg/kg body weight). No rat exhibited signs of peritonitis after the administration of 10% chloral hydrate. According to the Paxinos-Watson map, the PE-10 tube (outer diameter 0.6 mm and inner diameter 0.3 mm) was inserted into the skull about 3.5 mm, fixed with dental cement and disinfected. The incision was sutured and penicillin was injected to prevent wound infection. The rats were placed in an empty cage to be awakened. Each rat was kept in a single cage after surgery, and 3.4 μg/μL of methyllycaconitine was given after one week of routine feeding once 30 min before Morris water maze test. Rats with escape latency > l20S were identified as dementia rats by Morris water maze test. Rats (n = 20) without treatment were used as control.
Morris water maze test
Rats were subjected to double-blind water maze training [Citation14]. The positioning navigation experiment lasted for 5 days. Each day was divided into two periods of morning and afternoon, and in each time period, the rats were trained 4 times with an interval of >2 h. During the training, a quadrant was randomly selected, and the training was carried out from 4 different sites in 4 times in each time period. The time from entering water to climbing onto the platform was recorded, i.e. the escape latency.
After 5 days, the rats were subjected to the space exploration experiment of the Morris water maze. The platform was removed. The rats were put into water from a random quadrant and allowed to swim for 120 s. The number of times the rats crossed the original platform area were recorded, i.e. times of crossing platform.
Sample collection
After the water maze training, blood (2 mL) was taken from the posterior orbital venous plexus of rats and centrifuged at 1,000 g for 15 min to isolate the plasma. After that, rats were anesthetized with 2% sodium pentobarbital (30 mg/kg body weight; intraperitoneal injection) and then sacrificed by cervical dislocation. The mean weight of rats in the control group, PD group and PPD group was 608.5 + 25.913 g, 604.2 + 26.514 g, and 593.3 + 13.530 g, respectively, at the time of sacrifice. There was no significant difference in body weight among the three groups. Bilateral hippocampus was harvested from brain tissue on ice and was stored at −80 °C until use.
Enzyme-linked immunosorbent assay
The level of malondialdehyde (MDA) and superoxide dismutase (SOD) in plasma was determined by enzyme-linked immunosorbent assay (ELISA). The MDA ELISA Kit and SOD ELISA Kit (Cusabio, USA) were used. The procedures were conducted according to the protocols provided by the kits. The Absorbance value at 450 nm wavelength was read in a microplate reader (Thermo, USA). The experiment was repeated three times.
Real-time quantitative PCR
The mRNA level of nAChRα7 was detected by real-time quantitative PCR (RT-qPCR). Total RNA was extracted using an RNAprep pure Tissue Kit (Tiangen, China). RT-qPCR was performed with SuperReal Premix Plus (SYBER Green) (Tiangen, China). The primer sequence for nAChRα7 was as follows: Forward primer 3′-CTGATGAGCGCTTTGATGCC-5′ and reverse primer 3′-CTCCATAGGACCAGGACCCA-5′. β-Actin was used as an internal control, with primer sequences of CCCATCTATGAGGGTTACGC and TTTAATGTCACGCACGATTTC. The PCR procedure was 95 °C, 1 min, 95 °C, 10 s, 60 °C 34 s, and 40 cycles of 94 °C 1 min 30 s, 60 °C 1 min, 60 °C → 94 °C, 1.1 °C/s. The expression was calculated using 2−△△Ct as described previously.
Western blot
Total protein was isolated from brain tissues. The BCA method was used to determine the protein concentration. Proteins were separated by sodium dodecyl sulfate (SDS)-polyacrylamide gel electrophoresis and transferred to polyvinylidene fluoride membranes. After blocking, the membranes were incubated with primary antibodies against β-actin (1:200, Boster) and nAchRα7 (1:100, Biolegend, USA) at 4 °C overnight. Then the membranes were incubated with secondary antibodies of IgG (Boster, Wuhan, China) at 37 °C for 1 h. Bands were visualized using an enhanced chemiluminescence kit (Hanzhong, Tianjin, China). The gray values were analyzed by Quantity-one software (Bio-rad, USA). The relative protein level was determined by the ratio of the gray values of the target proteins to those of the β-actin bands.
Immunofluorescence
Brain tissues were made into tissue sections according to a routine procedure. Then, immunofluorescence was performed. Briefly, after blocking with 5% goat serum for 30 min, cells were incubated with the primary antibody of rabbit anti-NeuN antibody (1:100, Abcam, UK) and rat anti- nAchRα7 antibody overnight at 4 °C. After washing with PBS, secondary antibodies of FITC goat anti-rabbit IgG and CY3 goat anti-rat IgG (Boster, Wuhan, China) were added and incubated for 1 h under darkness. Then, the sections were stained with 10 μg/mL DAPI for 5 min. Finally, the sections were observed under a fluorescence microscope (Olympus, Japan). Image-Pro Plus 6.0 software (Media Cybernetics, USA) was used to measure the optical density value of each protein. Relative protein levels were expressed by the mean optical density value (integrated optical density divided by the relevant area).
Statistical analyses
The data were analyzed using SPSS 17.0 software. Data are expressed as the mean values with standard deviation (±SD). One-way analysis of variance (ANOVA) was used for data analysis followed by the least significant difference method (LSD). Differences were considered statistically significant at p < 0.05 level.
Results and discussion
Memory learning in PD and PDD model rats
The memory learning ability of PD and PDD model rats was detected by water maze test. As shown in , the escape latency of PD and PDD model groups was significantly increased compared to that of the control groups (p < 0.05). Compared with the PD model group, the escape latency of the PDD model group was significantly higher (p < 0.05). In the space exploration experiment, the crossing platform times of the PD and PDD model groups were significantly decreased compared to those of the control groups (p < 0.05). Compared with the PD model group, the crossing platform times of the PDD model rats were significantly shorter (p < 0.05). These results suggest that PDD is more serious than PD.
Table 1. Comparison of Morris water maze test results (mean ± SD).
Determination of SOD and MDA in plasma
To detect the level of SOD and MDA in plasma, ELISA was conducted. As shown in , the level of MDA in the control group was significantly lower than that in the PD and PDD model group (p < 0.05). The level of SOD in the control group was significantly higher than that in the PD and PDD model group () (p < 0.05). These results indicate that PD and PDD cause a decrease in SOD and MDA levels.
Differential expression of nAchRα7
The expression level of nAChRα7 directly or indirectly affects the incidence and prevalence of neurodegenerative diseases such as Parkinson's disease [Citation15]. To investigate the difference in nAChRα7 expression between the model group and the control group, the mRNA level and protein expression of nAChRα7 were quantitatively determined by RT-qPCR and western blot, respectively. RT-qPCR showed that the mRNA levels of nAChRα7 in the PDD and PD model group were significantly lower than that in the control group (p < 0.05, ). There was no significant difference between the PDD and PD model group (p > 0.05, ).
Figure 2. Expression of nAChRα7 in different groups. (A) Relative level of nAChRα7 mRNA detected by RT-qPCR. (B) and (C) Relative expression of nAChRα7 protein detected by Western Blot. β-actin was as an internal control. *p < 0.05.
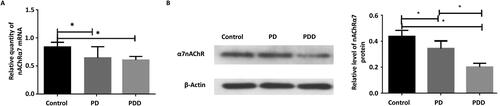
Western bolt showed that the protein expression of nAChRα7 in the control group was significantly higher than that in the PD and PDD model groups (p < 0.05, ). The expression of the nAChRα7 protein in the hippocampus of the PDD model group was significantly lower than that of the PD model group (p < 0.05, ). These results showed that both the nAChRα7 mRNA and protein levels were decreased in the hippocampus of the PD and PDD model rats.
Comparison of nAchRα7/NeuN expression between groups
To understand the subcellular localization and expression level of nAchRα7/NeuN in tissues, double fluorescent staining was used. The α7nAchR and NeuN proteins were stained by double fluorescent staining. As shown in , the distribution of the two proteins was different in different groups. The content of α7nAchR and NeuN protein in the control group was much higher than that in the PD group and the PDD group. The expression level of the NeuN protein was basically the same in the PD group and PDD group. However, the expression of the α7nAchR protein in the PDD group was much lower than that in the PD group (p < 0.05). These results show that the content of α7nAchR and NeuN in PDD and PD decreased, and the main difference between PDD and PD was the expression level of α7nAchR.
Comparative analysis
The pathological mechanism of PDD is still unclear [Citation16]. One study found that about 50% patients with PDD had pathological changes similar to Alzheimer's disease including senile plaques with β-amyloid aggregation and neurofibrillary tangles of tau [Citation17]. The more advanced the age of PD onset, the more cortical Lewy bodies, the more severe the amyloid vascular changes and the more obvious the pathological changes similar to Alzheimer's disease in PDD patients. Previous studies [Citation18,Citation19] have shown that nAChR is involved in many complex functions such as attention, learning, arousal and cognitive function. nAChRα7, one of the subtypes with more central nervous system distribution, plays an important role in the cognitive process [Citation20]. Pocivavsek et al. [Citation21] found that rats infused with the α4β2 receptor agonist, dihydrotricotine, had reduced spatial memory capacity in rats. Fernandes et al. [Citation22] showed that mice lacking the α7 subunit nAChR had low cognitive function such as learning and memory. In this study, the expression of nAChRα7 in the hippocampus region was investigated.
In this study, Morris water maze test showed that the spatial learning and memory ability of the PD and PDD model group were decreased, and the decrease was more obvious in the PDD model group. SOD and MDA levels are two important indicators in the oxidation-antioxidant system. SOD plays an antioxidant role and its content reflects the body's ability to scavenge oxidative free radicals. MDA is an indicator of oxidative stress [Citation23,Citation24]. ELISA showed that the SOD level in the PDD and PD model groups decreased significantly, while the MDA content increased significantly. RT-PCR and Western-Blot showed that the mRNA level and protein expression of nAChRα7 decreased in the PDD and PD model groups, which was significantly different from the control group. There was no significant difference in the nAChRα7 mRNA level between the PDD and PD model groups. There was a significant difference in the nAChRα7 protein expression between the PDD and PD model group. These results are consistent with the study by Barrantes et al. [Citation25] suggest that post-transcriptional changes were probably the basis for a significant decrease in nAChRa7 expression in the temporal cortex of Alzheimer's disease patients, with no reduction in mRNA. Immunofluorescence imaging in this study showed that the concentration of α7nAchR in the brain of the PDD and PD model group was significantly decreased. The concentration of NeuN in the brain in the two groups was similar. The nAchRα7 and NeuN levels in the PDD and PD model groups were significantly different from those in the control group.
Conclusions
In this study, the PDD model rats had decreased spatial learning and memory ability. The mRNA level and protein expression of nAChRα7 and the concentration of α7nAchR in the brain decreased in the PDD model group. The SOD level was decreased and the MDA level was increased in PD and PDD model rats. These results suggest that nAChRα7 could be used as the main target for detection of PDD and PD, and is probably one of the factors involved in the pathogenesis of PDD.
Disclosure statement
The authors report no conflict of interest
Data availability statement
The data that support the findings of this study are available on request from the corresponding author.
Additional information
Funding
References
- Cacabelos R. Parkinson's disease: from pathogenesis to pharmacogenomics. Int J Mol Sci. 2017;18(3):551.
- Fahn S. How do you treat motor complications in Parkinson's disease: medicine, surgery, or both? Ann Neurol. 2008;64(Suppl 2):S56–S64.
- Kieburtz K. Therapeutic strategies to prevent motor complications in Parkinson's disease. J Neurol. 2008;255(Suppl 4):42–45.
- Schapira AH, Emre M, Jenner P, et al. Levodopa in the treatment of Parkinson's disease. Eur J Neurol. 2009;16(9):982–989.
- Stocchi F, Tagliati M, Olanow CW. Treatment of levodopa-induced motor complications. Mov Disord. 2008;23(Suppl 3):S599–S612.
- Grando SA. Connections of nicotine to cancer. Nat Rev Cancer. 2014;14(6):419–429.
- Liu Y, Zeng X, Hui Y, et al. Activation of α7 nicotinic acetylcholine receptors protects astrocytes against oxidative stress-induced apoptosis: implications for Parkinson's disease. Neuropharmacology. 2015;91:87–96. PubMed PMID: 25486621; eng.
- Quik M, Zhang D, McGregor M, et al. Alpha7 nicotinic receptors as therapeutic targets for Parkinson's disease. Biochem Pharmacol. 2015;97(4):399–407. doi:.
- Gratwicke J, Jahanshahi M, Foltynie T. Parkinson's disease dementia: a neural networks perspective. Brain. 2015;138(Pt 6):1454–1476.
- Andriambeloson E, Huyard B, Poiraud E, et al. Methyllycaconitine- and scopolamine-induced cognitive dysfunction: differential reversal effect by cognition-enhancing drugs. Pharmacol Res Perspect. 2014;2(4):e00048.
- Cheng L, Chen L, Wei X, et al. NOD2 promotes dopaminergic degeneration regulated by NADPH oxidase 2 in 6-hydroxydopamine model of Parkinson's disease. J Neuroinflammation. 2018;15(1):243.
- Yan M, Chen H. A rat model of Parkinson's disease established by microinjection of 6 - hydroxydopamine into the different part of brain. J Pharm Pract. 2009;27(1):1–3.
- Jing ZH, Wei XM, Wang SH, et al. The synergetic effects of nitric oxide and nicotinic acetylcholine receptor on learning and memory of rats. Sheng Li Xue Bao. 2014;66(3):307–314.
- Li XY, Mei GH, Dong Q, et al. Enhanced neuroprotective effects of coadministration of tetrandrine with glutathione in preclinical model of Parkinson's disease. Parkinsons Dis. 2015;2015:931058. PubMed PMID: 26664824; PubMed Central PMCID: PMCPMC4667061.
- Bali ZK, Nagy LV, Budai D, et al. Facilitation and inhibition of firing activity and N-methyl-D-aspartate-evoked responses of CA1 hippocampal pyramidal cells by alpha7 nicotinic acetylcholine receptor selective compounds in vivo. Sci Rep. 2019;9(1):9324.
- Liao H, Fan J, Shen Q, et al. Alterations of interhemispheric functional connectivity in Parkinson's disease with depression: a resting-state functional MRI study. Front Hum Neurosci. 2020;14:193.
- Smith C, Malek N, Grosset K, et al. Neuropathology of dementia in patients with Parkinson's disease: a systematic review of autopsy studies. J Neurol Neurosurg Psychiatry. 2019;90(11):1234–1243.
- Titus DJ, Johnstone T, Johnson NH, et al. Positive allosteric modulation of the α7 nicotinic acetylcholine receptor as a treatment for cognitive deficits after traumatic brain injury. PLoS One. 2019;14(10):e0223180.
- van Goethem NP, Paes D, Puzzo D, et al. Antagonizing α7 nicotinic receptors with methyllycaconitine (MLA) potentiates receptor activity and memory acquisition. Cell Signal. 2019;62:109338.
- Tang X, Li Y, Ao J, et al. Role of α7nAChR-NMDAR in sevoflurane-induced memory deficits in the developing rat hippocampus. PLoS One. 2018;13(2):e0192498.
- Pocivavsek A, Icenogle L, Levin ED. Ventral hippocampal alpha7 and alpha4beta2 nicotinic receptor blockade and clozapine effects on memory in female rats. Psychopharmacology. 2006;188(4):597–604.
- Fernandes C, Hoyle E, Dempster E, et al. Performance deficit of alpha7 nicotinic receptor knockout mice in a delayed matching-to-place task suggests a mild impairment of working/episodic-like memory. Genes Brain Behav. 2006;5(6):433–440.
- Zhang Y, Li Y, Wang Y, et al. Effects of resveratrol on learning and memory in rats with vascular dementia. Mol Med Rep. 2019;20(5):4587–4593.
- Foyet HS, Keugong Wado E, Ngatanko Abaissou HH, et al. Anticholinesterase and antioxidant potential of hydromethanolic extract of Ziziphus mucronata (Rhamnaceae) leaves on scopolamine-induced memory and cognitive dysfunctions in mice. Evid Based Complement Alternat Med. 2019;2019:4568401.
- Barrantes FJ, Bermudez V, Borroni MV, et al. Boundary lipids in the nicotinic acetylcholine receptor microenvironment. J Mol Neurosci. 2010;40(1-2):87–90.