Abstract
Flavonoids are important polyphenols, and widely distributed in plants. Anthocyanidin reductase (ANR), a precursor enzyme in (-)- epicatechin synthesis, could play an important role in anthocyanins biosynthesis. In the present study, the ANR gene was cloned from Vitis vinifera L. cv. Cabernet Sauvignon. Compared with VvANR (GenBank Accession No. NM_001280956.1), they differed in only 4 bp and shared similarity of 99.6%. The ANR protein belongs to the cl33424 Superfamily. In addition, many light-responsive elements and abiotic stress-related elements (ABRE, MYB, WRKY, etc.) were found in the promoter region of the ANR gene. STRING analysis predicted that VvMYBPA1 and VvMYBPAR may be involved in regulating the expression of the ANR gene. Different UV-C irradiation times were used for Cabernet Sauvignon berries during grape berry development, and the comparison analysis showed that UV-C irradiation improved significantly the accumulation of catechin and epicatechin during 20–50 days after full bloom, especially, under UV-C irradiation for 5 min. Furthermore, UV-C irradiation for 5 min had a greater effect on the expression of the ANR gene, the accumulation of catechin and epicatechin, and the enzymatic activity of ANR than UV-C irradiation for 10 min. Through the virus-induced gene silencing system, the ANR-silenced grape leaves were chlorotic, in which the contents of catechin and epicatechin were both significantly lower than those in the control, showing a very significant difference, respectively. In summary, our results demonstrated that the ANR gene played a crucial role in the accumulation of epicatechin in grapes, and its expression was upregulated by UV-C irradiation.
Introduction
Flavonoids play an important role in the interaction between the environment and plants, protecting them against various biotic and abiotic stresses. Flavonoids include flavonols, flavan-3-ols, condensed tannins (also called proanthocyanidins [PA]) and anthocyanins in grape berries [Citation1]. Anthocyanins are a group of bioactive natural pigments with numerous important physiological and ecological functions in plants [Citation2], and benefits health care [Citation3,Citation4]. Anthocyanin biosynthesis has been extensively studied in fruit trees, including apples [Citation5–8], pomegranates [Citation9,Citation10], kiwifruit [Citation11], grapes [Citation12–14] and the model plant Arabidopsis thaliana [Citation15]. Flavan-3-ols (such as catechin and epicatechin), as the initiators for PA polymerization, are important for antioxidant activity, protecting human low-density lipoprotein against oxidation more efficiently [Citation16,Citation17]. There are three key enzymes that catalyze the biosynthesis of flavan-3-ols: leucoanthocyanidin reductase (LAR), anthocyanidin synthase (ANS) and anthocyanidin reductase (ANR). At present, the ANR gene has been cloned and characterized in Arabidopsis [Citation18,Citation19], Medicago truncatula [Citation20], Malus crabapple [Citation21] and Vitis vinifera [Citation22,Citation23].
Grapevine (Vitis vinifera L.) is one of the most valuable horticultural crops in the world. The accumulation of anthocyanin in grapes is regulated by environmental factors, including high or low temperature [Citation24], water [Citation25] and light [Citation26]. Previous studies found that visible light primarily induced biosynthesis of PAs and affected their compositions, whereas ultraviolet (UV) light specifically induced the biosynthesis of flavonols [Citation27]. González-Aguilar et al. [Citation28] found that total phenols, total flavonoids, enzymatic activity of phenylalanine ammonia-lyase (PAL) and lipoxygenase (LOX) increased under UV-C treatment.
Anthocyanins are specific to red cultivars in grapes and UV-C treatment influenced the accumulation of anthocyanin. V. vinifera L. cv. Cabernet Sauvignon, one of the most important red grape cultivars, was selected as the plant material. UV-C irradiation was applied to clarify the role of the ANR gene in the epicatechin biosynthetic pathway in grape berry development. Here, we reported that the content of epicatechin displayed a similar profile to the expression of the ANR gene and its protein during grape berry development, which suggested that the accumulation of epicatechin was induced by the ANR gene under UV-C irradiation. We also verified the functions of the ANR gene in flavan-3-ols accumulation by a virus-induced gene silencing (VIGS) system to provide useful information for further study on the regulation mechanism of the ANR gene.
Material and methods
Plant materials and treatments
V. vinifera L. Cabernet Sauvignon were grown with 1.0 m × 2.5 m in a vineyard of Shanxi Agricultural University, Taigu County, Shanxi Province, PR China. Three grapevine plants were chosen as a group at random, and three grape clusters were from the top, middle and bottom of the canopy on each plant, respectively. The data were obtained from three biological replicates. Three 40 W UV-C lights (254 nm, Guangze Special Lights Co. Limited, Tianjin, China) were placed at 1.0 m distance from the tree (power 95 μW/m2) between the plant trees. Three treatments were performed: no UV-C irradiation (CK), UV-C irradiation for 5 min and UV-C irradiation for 10 min. UV-C irradiation was conducted on 15, 25, 35, 45, 55, 65, 75, 85, 95 and 105 days after full bloom, respectively, and the grape clusters were harvested on the 5th day after UV-C irradiation, respectively. Unhealthy berries were removed before the samples were quickly frozen in liquid nitrogen, and then held at −80 °C until use.
Cloning of ANR gene and related bioinformatics analysis
The primers were designed according to the CDS sequence of VvANR gene (GenBank Accession No. NM_001280956.1) from V. vinifera cv. Pinot Noir, ANR-F: 5′-ATGGCCACCCAGCACCCCATC-3′, ANR-R: 5′-TCAATTCTGCAATAGCCCCTTG-3′ and polymerase chain reaction (PCR) amplification was performed using the reverse transcription cDNA as a template. The PCR products were examined by electrophoresing samples in a 1.0% agarose/ethidium bromide (EB) gel with GeneSnap from SynGene (Cambridge, UK), and the obtained PCR products were sequenced by BGI (Beijing Genomics Institute).
The online software Protparam (https://web.expasy.org/protparam/) was used to analyze and predict the physical and chemical properties of the ANR protein. The subcellular location was predicted on the Softberry online website (http://linux1.softberry.com/) using the ProtComp tool. Through the alignment in the database of GenBank with BLASTN, ANRs with high similarity were searched in different species, and a neighbor-joining phylogenetic tree was constructed using MEGA X software. The promoter sequence of the ANR gene was extracted using TBtools software [Citation29], and its cis-acting elements were analyzed in the PlantCARE database (http://bioinformatics.psb.ugent.be/webtools/plantcare/html/). The candidate proteins that interact with the ANR protein were searched through the online STRING tool (https://string-db.org/).
Real-time PCR
Total RNA from fruit was extracted using the CTAB method [Citation30]. First-strand cDNA was synthesized using PrimeScriptTM RT Reagent Kit with gDNA Eraser (TaKaRa). Real-time PCR reactions were run an Applied Biosystems 7500 Real-Time PCR System with SYBR Premix Ex Taq II (TaKaRa, Dalian, Liaoning, China). Each reaction was performed in triplicate with a reaction volume of 20 µL. Cycling parameters were 94 °C for 1 min, 40 cycles of 94 °C for 10 s, 60 °C for 30 s, 72 °C for 1 min. Grapevine VvUbiquitin (Accession No. BN000705) was used as the standard reference gene. The sequence of VvANR (Accession No. DQ129684.1), VvMYBPA1 (Accession No. AM259485) and VvMYBPAR (Accession No. EU919682.1) were obtained from NCBI (https://www.ncbi.nlm.nih.gov/), and their primers were designed using Primer Premier 5.0 software and listed .
Table 1. Primers list of related genes for Real-time PCR.
Activity assay of ANR protein
Detection of ANR activity was performed according to the method of Wen et al. [Citation31]. Protein content was determined as described by Bradford [Citation32], bovine serum albumin (BSA) as the standard. ANR activity was measured by epicatechin content (EC). One unit of ANR was defined as the amount needed to catalyze the substrate to produce epicatechin at 45 °C within 20 min, namely, mg epicatechin. mg−1 protein. min−1.
VIGS assay of ANR in grapevine leaves
A specific cDNA fragment of ANR containing BamH I and EcoR I restriction site was introduced into the pTRV2 vector to produce pTRV2-ANR. pTRV2 was used as the control. Both vectors were infiltrated into grape leaves along with pTRV1. The recombinant vector pTRV2-ANR was introduced into A. tumefaciens strain GV3101 by electrofusion method, which was used to infect young grapevine leaves with petioles. The leaves immersing in the GV3101 harboring the construct pTRV2-ANR were vacuumed in a drier for 5 min, grown in dark for 24 h. Subsequently, the leaves were maintained under a photoperiod of 16 h light and 8 h dark, 25 °C, humidity of 60% for 7–20 days before being sampled. The needle hole injection method was performed and a disposable sterile needle hole device was used to inject the dorsal main leaf vein of the leaf. The degree of injection was based on droplets at the needle hole. The leaves appeared chlorotic and were quickly frozen in liquid nitrogen and stored at −80 °C until use.
Content determination of catechin and epicatechin
Catechin and epicatechin were determined by switching the detection wavelength in high performance liquid chromatography (HPLC) method [Citation33]. Elution was carried out with a mobile phase delivered using an XBridge C18 HPLC pump (Waters Corp., Milford, MA, USA) at a flow rate 0.8 mL/min, and 30 °C. A Waters 2695 wavelength absorbance detector (Waters Corp., Milford, MA, USA) was used for UV detection at 280 nm. The concentration was quantitatively determined by calculating the peak areas.
Data analysis
All measurements were done in technical triplicate. Mean values were obtained from three biological replicates and data signals were analyzed with Excel 2019 software. Significant differences (*p < .05) between mean values were evaluated by t-test using the software SPSS Statistics v.20 (IBM, Chicago, IL, USA). The graphs were constructed using GraphPad 8 software.
Results and discussion
Bioinformatics analysis of the coding region of ANR gene
Using PCR amplification, we obtained a sequence of 1017 bp from V. vinifera Cabernet Sauvignon. Compared with ANR from V. vinifera cv. Pinot Noir, ANR from Cabernet Sauvignon differed in 4 bps, with similarity of 99.6% (). The analysis of the conserved domains, physicochemical properties and subcellular localization of ANR protein predicted that the ANR protein contained 339 amino acids, belonging to the cl33424 Superfamily, with molecular weight of 36.74 kD, an isoelectric point of 5.77, 39 positively charged residues and 34 negatively charged residues. Among them, the content of Pyl and Sec were the lowest, reaching 0.9%, while Ala had the highest content of 9.2%, the instability index was 31.21 and the fat coefficient was 92.04. The prediction of subcellular localization indicated that the ANR protein was mainly secreted extracellularly. In summary, the results provided an important reference for further study of the ANR gene.
Figure 1. Phylogenetic tree of ANR proteins from grapevines and other species. The ANR protein in grapevines was translated from the coding region sequence after cloning and sequencing. ANR proteins from other species were retrieved from the NCBI GenBank database.
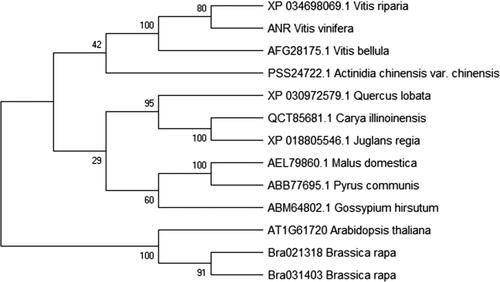
BLAST search and sequence alignment showed that the ANR protein shared 99.41% identity with V. riparia (XP_034698069.1), 98.2% with V. bellula (AFG28175. 1), 85.16% with Quercus lobata (XP_030972579.1), 84.37% with Malus domestica (AEL79860.1) and 83.78% with Pyrus communis (ABB77695.1). Using MEGA software, a phylogenetic tree of ANR with high similarity was constructed using the Neighbor-joining method, and the results displayed that the ANR had a high conservation with VvANR, being relatively distant from V. bellula but close to V. riparia, at the genetical level ().
Cis-acting elements prediction of ANR promoter
In order to analyze the potential molecular mechanism of the ANR gene, we obtained the upstream 2000 bp of ANR from the published V. vinifera genome, and identified the cis-acting elements in its promoter (2000 bp). The analysis identified the following regulatory elements (): light response elements (7), ABA response elements (1), MYB transcription factor binding sites (4), WRKY transcription factor binding sites (2) and so forth, which suggested that ANR may respond to UV-C irradiation and abiotic stresses. STRING database analysis demonstrated that some candidate proteins may interact with the ANR protein, such as VvCHI, VvF3H, VvUFGT and so forth (). Furthermore, two transcription factors, VvMYBPA1 and VvMYBPAR, may participate in the regulation of ANR gene expression.
Expression analysis of ANR, VvMYBPA1 and VvMYBPAR under UV-C irradiation
The ANR gene was investigated under UV-C irradiation during grape berry development to illustrate its function in epicatechin synthesis. ANR could be induced by UV-C irradiation (), and the expression level of ANR was higher during 20–50 days after full bloom than during 60–110 days after full bloom. For the effect of different irradiation times on the expression of the ANR gene, UV-C irradiation for 5 min functioned more potently than UV-C irradiation for 10 min. Whereas, VvMYBPA1 was also induced by UV-C irradiation, reaching the highest expression level 30 days after full bloom, but with a nonsignificant difference (). Moreover, during the first 50 days after full bloom, it had higher expression under UV-C irradiation for 5 min than under UV-C irradiation for 10 min, while during 60–110 days after full bloom, on the contrary, its expression was lower under UV-C irradiation for 5 min than under UV-C irradiation for 10 min, which was similar to VvMYBPAR (). Grapevine veraison occurred during 70 days after full bloom; clearly, the function of UV-C irradiation gradually decreased, especially, the effect of UV-C irradiation for 5 min on the investigated genes, ANR, VvMYBPA1 and VvMYBPAR. Therefore, the expression pattern of VvMYBPAR was close to that of the ANR gene during berry development under UV-C irradiation, and UV-C irradiation for 5 min may significantly induce the genes expression, while UV-C irradiation for 10 min may maintain the genes expression for a longer time.
Figure 3. Expression profiles of ANR (A), VvMYBPA1 (B) and VvMYBPAR (C) under UV-C irradiation during grape berry development. Sampling was performed 5 days after irradiation. Three grape clusters were from the top, middle and bottom of the canopy in one group. Error bars represent mean values ± SD (n = 3) of triplicate repeats. Upper case letters in each panel indicate significant differences at t-test p < .01 and lowercase letters indicate significant differences at p < .05.
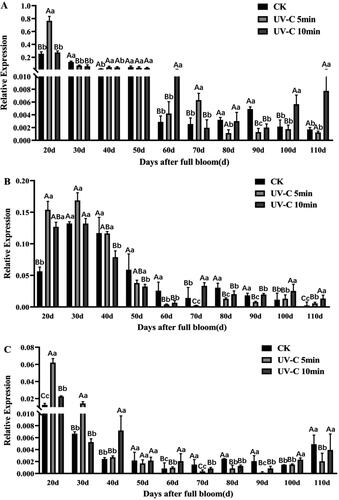
ANR enzyme activity increased after UV-C irradiation
The ANR enzyme activity under UV-C irradiation had a similar trend line to the non-irradiated control (CK), and reached the highest level at 100 days after full bloom with significant difference, which appeared higher during young berry, and decreased at berry maturity, which was in agreement with a previous study [Citation22]. Furthermore, the ANR enzyme activity was much higher under UV-C irradiation for 5 min than under UV-C radiation for 10 min and CK (). These results suggested that UV-C irradiation could increase the ANR enzyme activity.
Catechin and epicatechin were induced by UV-C irradiation
To illustrate the effect of the ANR protein under UV-C irradiation on catechin and epicatechin, we determined their contents. The contents of catechin and epicatechin both followed a decreasing trend during grape berry development in both durations of UV-C irradiation as well as in the non-irradiated control (CK) (). The accumulations of catechin had a similar trend to that of epicatechin both in the non-irradiated control (CK) and under UV-C irradiation. In short, UV-C irradiation contributed to the accumulation of catechin and epicatechin, and their contents were also higher under UV-C irradiation for 5 min than UV-C irradiation for 10 min during the first 50 days after full bloom, which was also found by Zhou et al. [Citation34].
Figure 5. Content of catechin (A) and epicatechin (B) during grape berry development after UV-C irradiation. Sampling was performed 5 days after irradiation. Upper case letters in each panel indicate significant differences at t-test p < .01 and lowercase letters indicate significant differences at p < .05
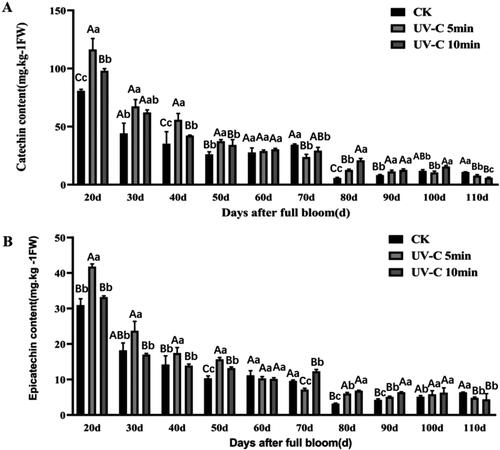
VIGS analysis of ANR in grapevine leaves
Flavonoids play an important role in disease resistance, signaling in plant–microbe interactions, protection from UV radiation and coloration of flowers and fruits [Citation35]. Flavonoids synthesis includes a branched pathway that yields both colorless compounds (flavonols) and colored pigments (anthocyanins, the polymeric phlobaphenes and PAs) [Citation36]. The formation of color, flavor, astringency and bitter taste in table grapevine and wine depend on the quantitative and qualitative patterns of PAs [Citation37,Citation38]. As we known, the VvANR gene is expressed throughout early flower and berry development, and encodes the enzyme responsible for converting anthocyanidins to flavan-3-ol [Citation19,Citation22].
To demonstrate whether the ANR protein was essential for catechin and epicatechin content in grapevine, we conducted pTRV2-ANR transient expression using VIGS system with two methods, vacuum infection and needle injection. The results showed that the ANR-silenced grape leaves were chlorotic (). Moreover, the contents of catechin and epicatechin in the ANR-silenced grape leaves were both significantly lower than the non-silenced control (). Therefore, we suggest that ANR was important for catechin and epicatechin content in grapevine.
Conclusions
In our study, the CDS sequence of the ANR gene was cloned from V. vinifera cv. Cabernet Sauvignon. Compared with VvANR from Pinot Noir, the obtained ANR gene differed in four bases, and the identity was 99.6%. According to the conserved protein domain analysis, the ANR protein belonged to the cl33424 superfamily. In addition, some light-responsive elements and abiotic stress-related elements (ABRE, MYB, WRKY, etc.) were found in the ANR promoter region. The ANR gene was induced by UV-C irradiation, especially, UV-C irradiation for 5 min which resulted in catechin and epicatechin accumulation during young fruit development. The subsequent VIGS analysis also showed that the contents of catechin and epicatechin in ANR-silenced grape leaves were both significantly lower than those in the non-silenced control, which provided a theoretical basis for revealing the molecular mechanism of the ANR gene modulating the accumulation of epicatechin under UV-C irradiation.
Supplemental Material
Download PDF (133.3 KB)Acknowledgment
The authors thank Wei Wang in the Experimental Center of Shanxi Agricultural University for providing experimental instrument.
Disclosure statement
No potential conflict of interest was reported by the authors.
Funding
The research was supported by the National Natural Science Foundation of China (No. 31372013), Key Research and Development Program Key Projects Scientific and Technological Project of Shanxi Province (201703D211001-4-2, 201803D211016-4), the Natural Science Foundation of Shanxi Province (201901D111222).
Data availability
The data supporting the findings of this study are available from the authors upon reasonable request.
Additional information
Funding
References
- Dixon RA, Xie DY, Sharma SB. Proanthocyanidins-a final frontier in flavonoid research? New Phytol. 2005;165(1):9–28.
- Ageorges A, Fernandez L, Merdinoglu D, et al. Four specific isogenes of the anthocyanin metabolic pathway are systematically co-expressed with the red colour of grape berries. Plant Sci. 2006;170(2):372–383.
- Jing P, Bomser J, Schwartz S, et al. Structure-function relationships of anthocyanins from various anthocyanin-rich extracts on the inhibition of colon cancer cell growth. J Agric Food Chem. 2008;56(20):9391–9398.
- He J, Giusti MM. Anthocyanins: natural colorants with health-promoting properties. Annu Rev Food Sci Technol. 2010;1:163–187.
- Honda C, Kotoda N, Wada M, et al. Anthocyanin biosynthetic genes are coordinately expressed during red coloration in apple skin. Plant Physiol Biochem. 2002;40(11):955–962.
- Meng R, Qu D, Liu Y, et al. Anthocyanin accumulation and related gene family expression in the skin of dark-grown red and non-red apples (Malus domestica Borkh.) in response to sunlight. Sci Hortic. 2015;189:66–73.
- Liu XJ, An XH, Liu x, et al. MdSnRK1.1 interacts with MdJAZ18 to regulate sucrose-induced anthocyanin and proanthocyanidin accumulation in apple. J Exp Bot. 2017;68(11):2977–2990.
- Jiang S, Wang N, Chen M, et al. Methylation of MdMYB1 locus mediated by RdDM pathway regulates anthocyanin biosynthesis in apple. Plant Biotechnol J. 2020;18(8):1736–1748.
- Rouholamin S, Zahedi B, Nazarian-Firouzabadi F, et al. Expression analysis of anthocyanin biosynthesis key regulatory genes involved in pomegranate (Punica granatum L.). Sci Hortic. 2015;186:84–88.
- Harel-Beja R, Tian L, Freilich S, et al. Gene expression and metabolite profiling analyses of developing pomegranate fruit peel reveal interactions between anthocyanin and punicalagin production. Tree Genet Genomes. 2019;15(2):22.
- Li W, Liu Y, Zeng S, et al. Gene expression profiling of development and anthocyanin accumulation in Kiwifruit (Actinidia chinensis) based on transcriptome sequencing. PLoS One. 2015;10(8):e0136439.
- Boss PK, Davies C, Robinson SP. Expression of anthocyanin biosynthesis pathway genes in red and white grapes. Plant Mol Biol. 1996;32(3):565–569.
- Bogs J, Jaffe FW, Takos AM, et al. The grapevine transcription factor VvMYBPA1 regulates proanthocyanidin synthesis during fruit development. Plant Physiol. 2007;143(3):1347–1361.
- Kekun Z, Zhongjie L, Le G, et al. Changes of anthocyanin component biosynthesis in 'Summer Black' grape berries after the red flesh mutation occurred. J Agric Food Chem. 2018;66(35):9209–9218.
- Shi MZ, Xie DY. Biosynthesis and metabolic engineering of anthocyanins in Arabidopsis thaliana. Recent Pat Biotechnol. 2014;8(1):47–60.
- Frankel EN, Kanner J, German JB, et al. Inhibition of oxidation of human low-density lipoprotein by phenolic substances in red wine. Lancet. 1993;341(8843):454–457.
- Meyer AS, Heinonen M, Frankel EN. Antioxidant interactions of catechin, cyanidin, caffeic acid, quercetin, and ellagic acid on human LDL oxidation. Food Chem. 1998;61(1–2):71–75.
- Pelletier MK, Murrell JR, Shirley BW. Characterization of flavonol synthase and leucoanthocyanidin dioxygenase genes in Arabidopsis. Further evidence for differential regulation of "early" and "late" genes. Plant Physiol. 1997;113(4):1437–1445.
- Xie DY, Sharma SB, Paiva NL, et al. Role of anthocyanidin reductase, encoded by BANYULS in plant flavonoid biosynthesis. Science. 2003;299(5605):396–399.
- Pang Y, Peel GJ, Wright E, et al. Early steps in proanthocyanidin biosynthesis in the model legume Medicago truncatula. Plant Physiol. 2007;145(3):601–615.
- Li H, Tian J, Yao YY, et al. Identification of leucoanthocyanidin reductase and anthocyanidin reductase genes involved in proanthocyanidin biosynthesis in Malus crabapple plants. Plant Physiol Biochem. 2019;139:141–151.
- Bogs J, Downey MO, Harvey JS, et al. Proanthocyanidin synthesis and expression of genes encoding leucoanthocyanidin reductase and anthocyanidin reductase in developing grape berries and grapevine leaves. Plant Physiol. 2005;139(2):652–663.
- Gagne S, Lacampagne S, Claisse O, et al. Leucoanthocyanidin reductase and anthocyanidin reductase gene expression and activity in flowers, young berries and skins of Vitis vinifera L. cv. Cabernet-Sauvignon during development. Plant Physiol Biochem. 2009;47(4):282–290.
- Kliewer WM, Torres RE. Effect of controlled day and night temperatures on grape coloration. Am J Enol Vitic. 1972;23(2):71–77.
- Kennedy JA, Matthews MA, Waterhouse AL. Effect of maturity and vine water status on grape skin and wine flavonoids. Am J Enol Vitic. 2002;53(4):268–274.
- Wang W, Tang K, Yang H-R, et al. Distribution of resveratrol and stilbene synthase in young grape plants (Vitis vinifera L. cv. Cabernet Sauvignon) and the effect of UV-C on its accumulation. Plant Physiol Biochem. 2010;48(2–3):142–152.
- Koyama K, Ikeda H, Poudel PR, et al. Light quality affects flavonoid biosynthesis in young berries of Cabernet Sauvignon grape. Phytochemistry. 2012;78:54–64.
- González-Aguilar GA, Zavaleta-Gatica R, Tiznado-Hernández ME. Improving postharvest quality of mango 'Haden' by UV-C treatment. Postharvest Biol Technol. 2007;45(1):108–116.
- Chen C, Chen H, Zhang Y, et al. TBtools: an integrative toolkit developed for interactive analyses of big biological data. Mol Plant. 2020;13(8):1194–1202.
- Wen PF, Chen JY, Wan SB, et al. Salicylic acid activates phenylalanine ammonia-lyase in grape berry in response to high temperature stress. Plant Growth Regul. 2008;55(1):1–10.
- Wen PF, Xing YF, Niu TQ, et al. Accumulation of flavanols, expression of leucoanthocyanidin reductase induced by UV-C irradiation during grape berry development. Sci Agric Sin. 2012;45(21):4428–4436.
- Bradford MM. A rapid and sensitive method for the quantitation of microgram quantities of protein utilizing the principle of protein-dye binding. Anal Biochem. 1976;72(1–2):248–254.
- Zhang A, Fang YL, Wang H, et al. Simultaneous determination of individual phenolics in grape tissues by switching detection wavelength in high performance liquid chromatography. Chin J Anal Chem. 2007;35(11):1614–1618.
- Zhou D, Liu Q, Peng J, et al. Metabolic analysis of phenolic profiles reveals the enhancements of anthocyanins and procyanidins in postharvest peach as affected by hot air and ultraviolet C. Postharvest Biol Technol. 2020;167:111227.
- Harborne JB, Williams CA. Advances in flavonoid research since 1992. Phytochemistry. 2000;55(6):481–504.
- Koes R, Verweij W, Quattrocchio F. Flavonoids: a colorful model for the regulation and evolution of biochemical pathways. Trends Plant Sci. 2005;10(5):236–242.
- Bagchi D, Bagchi M, Stohs SJ, et al. Free radicals and grape seed proanthocyanidin extract: importance in human health and disease prevention. Toxicology. 2000;148(2–3):187–197.
- Wen KS, Ruan X, Wang J, et al. Optimizing nucleophilic depolymerization of proanthocyanidins in grape seeds to dimeric proanthocyanidin B1 or B2. J Agric Food Chem. 2019;67(21):5978–5988.