Abstract
The functions of a large number of genes, including gene families with domains of unknown functions (DUF), still remain unclear. In this study, we analyzed four members of OsDUF1664 (OsDUF1664.1-OsDUF1664.4) in rice Nipponbare. By phylogenetic analysis, DUF1664 members in rice and Arabidopsis were classified into three major groups (I, II, III). Under drought conditions, the expression level of OsDUF1664.3 in rice was significantly elevated. Overexpression of OsDUF1664.3 in Escherichia coli led to a significant enhancement of catalase (CAT) and peroxidase (POD) activities, and improved bacterial resistance to drought. The results of this study will provide important information for further study of the function of the OsDUF1664 family in rice.
Keywords:
Introduction
Due to the variability of external environmental factors, plants often face the stresses of one or more adverse environmental factors, which ultimately affect the normal growth and development of plants [Citation1]. Abiotic stresses in environmental stress mainly include drought stress, salt stress, high and low temperature stress, nutrient stress and heavy metal stress [Citation2]. Affected by abiotic stress, the average yield of crops can even be reduced by more than 50%, and by 2050, world food production needs to increase at least twice to meet the needs of population growth [Citation3]. Drought is an important limiting factor for food production. In general, drought affects the normal growth and development of plants by affecting the plasma membrane, the photosynthetic rate and disrupting the levels of plant hormones [Citation4, Citation5]. In order to avoid the harmful effects of the changing environment, plants have developed a number of physiological and biochemical strategies [Citation6], but the specific regulatory mechanisms for drought are not fully understood.
The domain of unknown function (DUF) is a protein domain with unknown function. When a protein family with no functional annotation is added to the Pfam database (http://pfam.xfam.org/family), this family is named DUF plus a number. Currently, there are approximately 3000 unknown functional domain protein families that are collected in the protein family database, accounting for 26.5% of the protein families (Pfam) [Citation7,Citation8]. At present, the functions of only a few DUF proteins have been elucidated recently. Some DUF-containing proteins are involved in plant growth and development, such as members of DUF231, DUF724 and DUF1218 families [Citation9–11], while some other DUF-containing proteins [Citation12–14], play important roles in plant stress responses. TaSRG, a wheat DUF662 domain-containing transcription factor, significantly affects salt tolerance in transgenic rice and Arabidopsis [Citation12]. Arabidopsis RING-DUF1117 E3 ubiquitin ligases genes AtRDUF1 and AtRDUF2 are induced by ABA and drought stress, and suppression of Arabidopsis AtRDUF1 and AtRDUF2 reduced tolerance to ABA-mediated drought stress [Citation13]. OsDSR2, encoding a protein with a DUF966 domain, negatively regulated rice response to salt and simulated drought stresses as well as ABA signaling [Citation14]. The transcriptional levels of some DUF1618 gene family members varied under stress and hormone treatment conditions, so it was suggested that some DUF1618 members were involved in stress responses [Citation15]. OsSIDP366, a DUF1644 gene, positively regulates salt and drought resistance in rice [Citation16].
The OsDUF1664 family consists of plant proteins of unknown specific function with an average length of approximately 100 residues. There are five DUF1664 members in Arabidopsis and the function of all members is also not reported. Currently, the OsDUF1664 family in rice has not been functionally identified. In this study, we focused on the identification and functional analysis of the OsDUF1664 family. We also studied the expression profiles of four members of OsDUF1664 and their expression patterns under various stresses. We found that overexpression of OsDUF1664.3 in Escherichia coli could improve the tolerance to drought.
Materials and methods
Database searches
The sequence of assumed OsDUF1664 family proteins in rice was obtained by retrieving the rice genome annotation project(RGAP version 7)database (http://rice.plantbiology.msu.edu/) with domain number PF07889. Then we downloaded all the related protein sequences of the OsDUF1664 family from RGAP and confirmed with the SMART database (http://smart.embl-heidelberg.de/smart/batch.pl) [Citation17]. Five DUF1664 members’ sequences in Arabidopsis were also downloaded from the Arabidopsis information resource (https://www.arabidopsis.org). If there were several gene models at one locus, only the complete gene model was selected for further sequence analysis.
Sequence analysis
The information about chromosomal localization, amino acid length, full-length cDNA accessions for each OsDUF1664 family member was obtained from the rice genome annotation project. The protein localization of OsDUF1664 members was predicted by online protein location prediction software TargetP 2.0 (http://www.cbs.dtu.dk/services/TargetP/) [Citation18]. Sequence alignment was done using Clustal Omega (https://www.ebi.ac.uk/Tools/msa/clustalo). In order to understand the evolutionary relationship among members of DUF1664, we constructed a neighbor-joining (NJ) phylogenetic tree with 1000 bootstrap replicates using MEGA4 [Citation19].
Plant growth and various stress and ABA treatment
Rice (Oryza sativa L. subsp. japonica cv. Nipponbare) seeds were germinated for 3 days before they were transferred to a 30-L nutrient solution container for hydroponic growth [Citation20]. In order to further understand the expression patterns of OsDUF1664 family members, 15 different tissues of rice Nipponbare under normal conditions were sampled, as follows: Lb1, leaf blade at the four-leaf stage; Lb2, leaf blade from plants with four tillers; Lb3, leaf blade at the ripening stage; Ls1, leaf sheath at the four-leaf stage; Ls2, leaf sheath from plants with four tillers; Rt1, root at the four-leaf stage; Rt2, root from plants with four tillers; St1, stem from plants with four tillers; St2, stem at the ripening stage; An, 1.2–1.5 mm anther; Pi, pistil from 10–14 cm inflorescence; Em1, embryo at 7 days after flowering; Em2, embryo at 28 days after flowering; En1, endosperm at 7 days after flowering; En2, endosperm at 28 days after flowering.
When the fourth leaf of rice seedlings appeared, they were subjected to various stresses and ABA treatment. For the salt treatment, high salinity stress was achieved by adding NaCl to the solution to a final concentration of about 200 mmol/L. For the drought tests, intact seedlings were put in the air without water supply. For the cold tests, the seedlings were transferred to a chamber at 4 °C (12-h-light/12-h-dark cycle). For ABA treatment, 100 μmol/L ABA was sprayed onto the leaves of seedlings. For heat treatment, the seedlings were exposed to 42 °C. Stress treatment continued until the seedlings were sampled. For salt stress and drought stress, seedlings were sampled at 0, 4, 8 and 16 h. For ABA, heat and cold stress, seedlings were sampled at 0, 1, 3, 8 h.
RNA extraction and real-time polymerase chain reaction
The total RNA was extracted using Trizol reagent (GIBCO, Burlington, ON, USA) according to the manufacturer’s instructions. In order to degrade any possible DNA contamination in total RNA, it was treated with RNase-free DNase for 15 min. Real-time PCR was performed as described before [Citation21]. The rice Actin1 gene (LOC_Os03g50885) was used as an internal control with primers 5′-TGGCATCTCTCAGCACATTC-3′ and 5′-TGCACAATGGATGGGCCAGA-3′. The primers used in the expression analysis of the OsDUF1664 gene family members are listed in in the Supplementary Appendix. The 2-△△Ct method was used to analyze the relative expression data as mentioned previously [Citation22].
Table 1. OsDUF1664 genes and the properties of the putative proteins they encode.
Assay for drought stress tolerance of E. coli transformants
In order to construct the expression vector pET32a-OsDUF1664.3, specific primers were designed as follows: sense, 5′-GGATCCATGGTGCTTGGCAACGTCGC-3′ (BamHI site underlined) and antisense, 5′-AAGCTT AACAAAGGCAACCAACCAGT-3′ (HindIII site underlined). The amplified products were cloned into a pET32a vector at the BamHI-HindIII site to express the fusion protein, which has a Trx•Tag™ thioredoxin at the N-terminus [Citation23]. The transformed E. coli Rosetta cells (Sanborn, MN, USA) were grown in Luria–Bertani (LB) liquid medium containing 100 µg/mL of ampicillin at 37 °C overnight, then they were inoculated into fresh LB medium (1:100 dilution) supplemented with ampicillin (100 µg/mL) to be incubated until the exponential growth phase (optical density at 600 nm of 0.5–0.6). Isopropylthio-β-D-galactoside (IPTG) was added into the cultures to induce the expression of the transformed gene. For the drought (mimic) tolerance assay, the growth curve of E. coli transformants was plotted. The OD values of pET-32a, pET32a-OsDUF1664.3 cells were measured at 0, 2 h, 4 h, 6 h, 8 h, 10 h, 12 h, 14 h, 16 h and 18 h under normal conditions and 300 mmol/L mannitol treatment.
Measurements of antioxidant enzyme activity
The bacteria were collected by centrifugation. After ultrasonic cell-breakage at 4 °C, the supernatant (as a crude enzyme solution) was collected by spinning at 12,000 rpm (13,200 xg rcf) for 20 min. Catalase (CAT), superoxide dismutase (SOD) and peroxidase (POD) activities were determined as described previously [Citation24].
Data analysis
The experiment was arranged as a completely randomized design and all the experiments were repeated three times. Data are mean values from three independent experiments, with standard error of the means. Statistical analysis was performed using the Student’s t-test by EXCEL version 2003.
Results and discussion
Identification and sequence analysis of OsDUF1664 family members in rice
We searched the rice genome annotation project (RGAP) using domain number PF07889 and obtained four members of the OsDUF1664 family. And then these OsDUF1664 family members were confirmed by the SMART database ( in the Supplementary Appendix). The four genes are named OsDUF1664.1 to OsDUF1664.4 according to their position on the pseudomolecules (). These members are distributed on chromosome 1 (OsDUF1664.1 and OsDUF1664.2), chromosome 5 (OsDUF1664.3 and OsDUF1664.4) (). Other information about the OsDUF1664 family, including the amino acid length, full length cDNA accessions, molecular weights (MW), isoelectric points (pI) and subcellular localizations, is listed in . The predicted length of the OsDUF1664 proteins varies from 287 (OsDUF1664.4) to 367 (OsDUF1664.3) amino acids (). All the members of OsDUF1664 family are located in the secretory pathway by online protein location prediction software TargetP 2.0.
Figure 1. Phylogenetic relationship of OsDUF1664 members in rice and five DUF1664 members in Arabidopsis. Note: The unrooted tree was generated using MEGA4.0 program by the maximum-likelihood method.
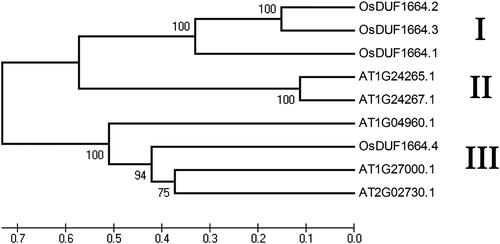
The multiple sequence alignment analysis of the OsDUF1664 members was performed using Clustal Omega (https://www.ebi.ac.uk/Tools/msa/clustalo) ( in the Supplementary Appendix). The percent identity was 47.40% for OsDUF1664.1 and OsDUF1664.2, 55.26% for OsDUF1664.1 and OsDUF1664.3, 25.48% for OsDUF1664.1 and OsDUF1664.4, 78.39% for OsDUF1664.2 and OsDUF1664.3, 24.81% for OsDUF1664.2 and OsDUF1664.4 and 28.25 for OsDUF1664.3 and OsDUF1664.4. The phylogenetic analysis was carried out using MEGA4 in order to examine the evolutionary relationship among DUF1664 family members in rice and Arabidopsis. Here, the reference sequence includes five Arabidopsis DUF1664 members. The results showed that all the members of the DUF1664 family can be divided into three major groups (I, II, III) (). Group I consists of 3 members of OsDUF1664 family in rice (OsDUF1664.1, OsDUF1664.2 and OsDUF1664.3). Group II contains 2 members of Arabidopsis DUF1664 (AT1G24265.1 and AT1G24267.1). Group III includes one member in rice (OsDUF1664.4) and three members in Arabidopsis (AT1G04960.1, AT1G27000.1 and AT1G02730.1). OsDUF1664.2 and OsDUF1664.3 have the highest similarity in the sequences and they are very close in evolutionary relationship. OsDUF1664.4 has low similarity with three other members of OsDUF1664 in rice and it was classified into group III.
Figure 2. Real-time PCR analysis of OsDUF1664 genes in different tissues of Nipponbare rice. Fifteen representative tissues are as follows: Lb1, leaf blade at four-leaf stage; Lb2, leaf blade from plants with four tillers; Lb3, leaf blade at ripening stage; Ls1, leaf sheath at four-leaf stage; Ls2, leaf sheath from plants with four tillers; Rt1, root at four-leaf stage; Rt2, root from plants with four tillers; St1, stem from plants with four tillers; St2, stem at ripening stage; An, 1.2–1.5 mm anther; Pi, pistil from 10–14 cm inflorescence; Em1, embryo at 7 days after flowering; Em2, embryo at 28 days after flowering; En1, endosperm at 7 days after flowering; En2, endosperm at 28 days after flowering. Note: The rice Actin1 transcript levels were used as internal controls. Error bars indicate standard error of the means based on three biological replicates.
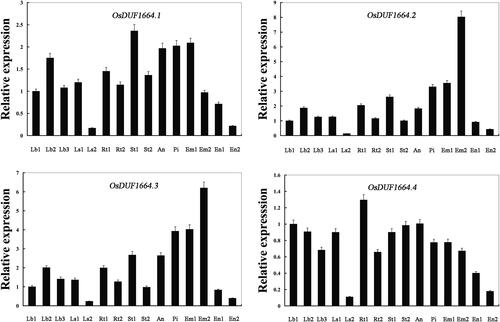
The rice genome includes 10 duplicated blocks accounting for 45% of its total size [Citation25]. Both OsDUF1664.1 and OsDUF1664.2 are located on chromosome 1, and both OsDUF1664.3 and OsDUF1664.4 are located on chromosome 5. It is possible that these genes of OsDUF1664 family members were the results of a segmental duplication event in the evolution of the genome, similar to that previously suggested for OsDUF866, OsDUF946, OsDUF936, OsDUF829 and OsDUF1191 family members [Citation21, Citation26–29].
Expression patterns of OsDUF1664 family members
So far, few studies have been done about the gene expression patterns of OsDUF1664 genes. Therefore, we used real-time PCR to investigate the expression patterns of OsDUF1664 family genes in 15 samples from different tissues or stages (). The results showed that the expression profiles of OsDUF1664.2 and OsDUF1664.3 are quite similar: the highest expression in Em2 (embryo at 28 days after flowering) while the lowest in Ls2 (leaf sheath from plants with four tillers). For OsDUF1664.1, the highest expression was in the St1 (stem from plants with four tillers), however the lowest expression was in the Ls2 (leaf sheath from plants with four tillers). For OsDUF1664.4, the expression level in the Rt1 (root at four-leaf stage) was the highest, whereas the expression level in the Ls2 (leaf sheath from plants with four tillers) was the lowest.
Real-time PCR was performed to examine the expression of OsDUF1664 family genes under various stress conditions and ABA treatment (). The ABA-signaling pathway is central to drought- and salt-stress responses in plants. In addition, ABA is required for freezing tolerance, which also involves the induction of dehydration tolerance genes [Citation30]. So ABA treatment was added when rice seedlings were subjected to various stresses. The expression of OsDUF1664.1 was significantly (P < 0.01) downregulated at the mRNA level under drought conditions. For OsDUF1664.2, the expression remained unchanged under drought, salt, cold, heat stress conditions and ABA treatment. Under drought and salt conditions, the expression of OsDUF1664.3 was significantly (P < 0.01) upregulated. For OsDUF1664.4, the expression was significantly (P < 0.01) upregulated under drought treatment, but significantly (P < 0.05) downregulated under salt conditions.
Figure 3. Relative expression levels of OsDUF1664 genes in Nipponbare rice seedlings at the emergence of the fourth leaf under various stress conditions and ABA treatment detected by real time PCR. D, drought; S, salt; C; cold; H, heat; A, ABA. Note: For salt stress and drought stress, seedlings were sampled at 0, 4, 8 and 16 h. For ABA, heat and cold stress, seedlings were sampled at 0, 1, 3, 8 h. The rice Actin1 transcript levels were used as internal controls. Error bars indicate standard error of the means based on three biological replicates. *P < 0.05 (Student’s t test). **P < 0.01 (Student’s t test).
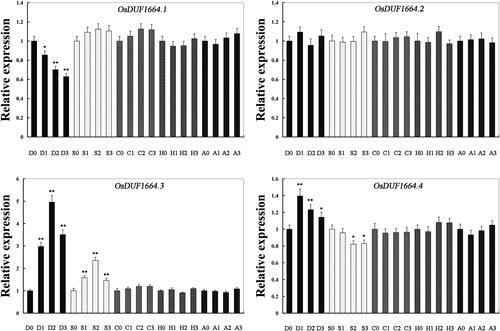
In general, gene family members display similar increase or decrease under various stresses conditions [Citation31–33]. In this study, the expression of OsDUF1664 family under abiotic stresses conditions and ABA treatment was examined, and surprisingly, we found the four members of OsDUF1164 family in rice displayed greatly distinct expression patterns under various stresses conditions and ABA treatment (). For OsDUF1164.2 and OsDUF1164.3 especially, although they had similar sequence and expression profiles in 15 samples from different tissues or stages, their coping strategies were completely different when dealing with abiotic stresses. We thus suggest that OsDUF1664 family members may play different roles in response to various stresses.
OsDUF1664.3 improved drought stress resistance in transgenic E. coli
Since the expression levels of OsDUF1664.3 were significantly elevated under drought conditions, we hypothesized that this gene may be involved in response to drought stress. We overexpressed the gene in E. coli and then examined the resistance of recombinant bacteria to drought stress. Under normal conditions, there was no evident difference in the number of colonies between transgenic lines (E. coli transformed with pET32a-OsDUF1664.3) and the control (E. coli transformed with pET-32a). Under drought conditions, the growth rate in E. coli transformed with pET32a-OsDUF1664.3 was much higher than that of the control from 4 to 18 h (). This result indicated that the overexpression of OsDUF1664.3 in E. coli may significantly improve the resistance to drought.
OsDUF1664.3 improves the antioxidant defenses of E. coli transformants grown under drought stress conditions
In order to investigate the effects of OsDUF1664.3 on the intracellular antioxidant ability, we determined catalase (CAT), superoxide dismutase (SOD) and peroxidase (POD) activities of E. coli transformants and control bacteria. Under normal conditions, there was no evident difference in CAT, SOD and POD activities between the transgenic line and the control. Under drought conditions, CAT and POD activities of E. coli transformed with pET32a-OsDUF1664.3 were significantly (P < 0.01) higher than those of the control from 4 to 8 h, while there was no significant difference in SOD activity (). These results indicated that the improvement in tolerance to stress in E. coli OsDUF1664.3 transformants may be related to the increase in the activities of CAT and POD under drought stress conditions.
Figure 5. Antioxidant enzyme activities in E. coli transformants overexpressing OsDUF1664.3 under drought (mimic) stress. Note: After E. coli transformants were exposed to drought conditions (300 mmol/L mannitol treatment) for 0, 4, and 8 h, respectively, catalase (CAT), superoxide dismutase (SOD) and peroxidase (POD) activities were determined. Error bars indicate standard error of the means based on three biological replicates. **P < 0.01 (Student’s t test).
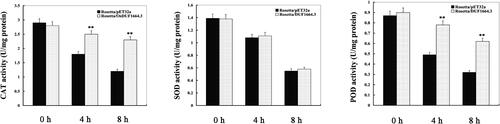
Production of reactive oxygen species (ROS) is a frequent event in organisms when they suffer from abiotic stresses, including drought [Citation34–36]. ROS production can cause oxidative damage on membrane lipids and perturbation of cell membrane function [Citation37]. It is therefore of high importance to maintain the balance between ROS generation and ROS scavenging in cells subjected to abiotic stresses. In this study, we observed an increase in the activities of CAT and POD in transgenic E. coli. This increase possibly indicates that the extent of damage to the E. coli transformed with pET32a-OsDUF1664.3 exposed to drought stress triggered a more pronounced antioxidant defense response than that in the control bacteria exposed to the same stress.
At present, the specific mechanism by which drought tolerance is enhanced in E. coli recombinants transformed with OsDUF1664.3 remains unclear. Under drought conditions, it is possible that the drought stress signal is enhanced in E. coli recombinants transformed with OsDUF1664.3, which, in turn, promotes the expression of downstream stress-related genes, including genes encoding ROS scavenging enzymes, leading to better improvement in drought tolerance in transgenic E. coli.
In our previous study, OsDUF866.1 [Citation21], OsDUF872.2 [Citation38], OsDUF1191.4 [Citation29] improved heat stress resistance, while OsDUF829.2, OsDUF829.4 [Citation28] and OsDUF936.6 [Citation27] improved the tolerance to salt in transgenic E. coli. In addition, OsDUF810.7 [Citation39] and OsDUF946.4 improved both salt and drought stress resistance in transgenic E. coli. In this study, OsDUF1664.3 improved the drought resistance in transgenic E. coli. In order to improve plant abiotic stress resistance, we can consider the DUF family members as a genetic resource of high potential.
Conclusions
In summary, this study describes sequence information regarding the OsDUF1664 family members and the expression patterns of OsDUF1664 family members in different tissues, and under various abiotic stresses and ABA. OsDUF1664.3 could enhance the activity of ROS scavenging enzymes and improve the resistance to drought of transgenic E. coli. These data can provide important reference for further studies of the functions of the OsDUF1664 family.
Supplemental Material
Download PDF (350.7 KB)Disclosure statement
No potential conflict of interest was reported by the authors.
Additional information
Funding
References
- Nadeem SM, Ahmad M, Zahir ZA, et al. The role of mycorrhizae and plant growth promoting rhizobacteria (PGPR) in improving crop productivity under stressful environments. Biotechnol Adv. 2014;32(2):429–448.
- Baillo EH, Kimotho RN, Zhang ZB, et al. Transcription factors associated with abiotic and biotic stress tolerance and their potential for crops improvement. Genes. 2019;10(10):771.
- Boyer JS. Plant productivity and environment. Science. 1982;218(4571):443–448.
- Taylor NL, Tan YF, Jacoby RP, et al. Abiotic environmental stress induced changes in the Arabidopsis thaliana chloroplast, mitochondria and peroxisome proteomes. J Proteomics. 2009;72(3):367–378.
- Hu Y, Wu QY, Peng Z, et al. Silencing of OsGRXS17 in rice improves drought stress tolerance by modulating ROS accumulation and stomatal closure. Sci Rep. 2017;7(1):15950.
- Golldack D, Luking I, Yang O. Plant tolerance to drought and salinity: stress regulating transcription factors and their functional significance in the cellular transcriptional network. Plant Cell Rep. 2011;30(8):1383–1391.
- Bateman A, Coggill P, Finn RD. DUFs: families in search of function. Acta Crystallogr Sect F Struct Biol Cryst Commun. 2010;66(Pt 10):1148–1152.
- Goodacre NF, Gerloff DL, Uetz P. Protein domains of unknown function are essential in bacteria. MBio. 2013;5(1):e00744-13.
- Bischoff V, Nita S, Neumetzler L, et al. TRICHOME BIREFRINGENCE and its homolog AT5G01360 encode plant-specific DUF231 proteins required for cellulose biosynthesis in Arabidopsis. Plant Physiol. 2010;153(2):590–602.
- Cao X, Yang KZ, Xia C, et al. Characterization of DUF724 gene family in Arabidopsis thaliana. Plant Mol Biol. 2010;72(1–2):61–73.
- Mewalal R, Mizrachi E, Coetzee B, et al. The Arabidopsis domain of unknown function 1218 (DUF1218) containing proteins, MODIFYING WALL LIGNIN-1 and 2 (At1g31720/MWL-1 and At4g19370/MWL-2) function redundantly to alter secondary cell wall lignin content. PLoS One. 2016;11(3):e0150254.
- He X, Hou X, Shen Y, et al. TaSRG, a wheat transcription factor, significantly affects salt tolerance in transgenic rice and Arabidopsis. FEBS Lett. 2011;585(8):1231–1237.
- Kim SJ, Ryu MY, Kim WT. Suppression of Arabidopsis RING-DUF1117 E3 ubiquitin ligases, AtRDUF1 and AtRDUF2, reduces tolerance to ABA-mediated drought stress. Biochem Biophys Res Commun. 2012;420(1):141–147.
- Luo C, Guo C, Wang W, et al. Overexpression of a new stress-repressive gene OsDSR2 encoding a protein with a DUF966 domain increases salt and simulated drought stress sensitivities and reduces ABA sensitivity in rice. Plant Cell Rep. 2014;33(2):323–336.
- Wang L, Shen R, Chen LT, et al. Characterization of a novel DUF1618 gene family in rice. J Integr Plant Biol. 2014;56(2):151–158.
- Guo C, Luo C, Guo L, et al. OsSIDP366, a DUF1644 gene, positively regulates responses to drought and salt stresses in rice. J Integr Plant Biol. 2016;58(5):492–502.
- Letunic I, Doerks T, Bork P. SMART: recent updates, new developments and status in 2015. Nucleic Acids Res. 2014;43:257–260.
- Emanuelsson O, Brunak S, von Heijne G, et al. Locating proteins in the cell using TargetP, SignalP and related tools. Nat Protoc. 2007;2(4):953–971.
- Tamura K, Dudley J, Nei M, et al. MEGA4: molecular evolutionary genetics analysis (MEGA) software version 4.0. Mol Biol Evol. 2007;24(8):1596–1599.
- Li L, Liu C, Lian X. Gene expression profiles in rice roots under low phosphorus stress. Plant Mol Biol. 2010;72(4–5):423–432.
- Li L, Xie C, Ye T, et al. Molecular characterization, expression pattern, and function analysis of the rice OsDUF866 family. Biotechnol Biotechnol Equip. 2017;31(2):243–249.
- Livak KJ, Schmittgen TD. Analysis of relative gene expression data using real-time quantitative PCR and the 2(-Delta Delta C(T)) Method. Methods. 2001;25(4):402–408.
- LaVallie ER, DiBlasio EA, Kovacic S, et al. A thioredoxin gene fusion expression system that circumvents inclusion body formation in the E. coli cytoplasm. Biotechnology (N Y). 1993;11:187–193.
- Liang Y, Chen Q, Liu Q, et al. Exogenous silicon (Si) increases antioxidant enzyme activity and reduces lipid peroxidation in roots of salt-stressed barley (Hordeum vulgare L.). J Plant Physiol. 2003;160(10):1157–1164.
- Wang X, Shi X, Hao B, et al. Duplication and DNA segmental loss in the rice genome: implications for diploidization. New Phytol. 2005;165(3):937–946.
- Li L, Ye T, Xu J, et al. Molecular characterization and function analysis of the rice OsDUF946 family. Biotechnol Biotechnol Equip. 2017;31(3):477–485.
- Li L, Ye T, Guan Y, et al. Genome-wide identification and analyses of the rice OsDUF936 family. Biotechnol Biotechnol Equip. 2018;32(2):309–315.
- Li L, Lv M, Zhao L, et al. Molecular characterization and function analysis of the rice OsDUF829 family. Biotechnol Biotechnol Equip. 2018;32(3):550–557.
- Lv M, Hou D, Zhang L, et al. Molecular characterization and function analysis of the rice OsDUF1191 family. Biotechnol Biotechnol Equip. 2019;33(1):1608–1615.
- Zhu JK. Abiotic stress signaling and responses in plants. Cell. 2016;167(2):313–324.
- Dubouzet JG, Sakuma Y, Ito Y, et al. OsDREB genes in rice, Oryza sativa L., encode transcription activators that function in drought-, high-salt- and cold-responsive gene expression. Plant J. 2003;33(4):751–763.
- Ito Y, Katsura K, Maruyama K, et al. Functional analysis of rice DREB1/CBF-type transcription factors involved in cold-responsive gene expression in transgenic rice. Plant Cell Physiol. 2006;47(1):141–153.
- Su C, Wang Y, Hsieh TH, et al. A novel MYBS3-dependent pathway confers cold tolerance in rice. Plant Physiol. 2010;153(1):145–158.
- Miller G, Shulaev V, Mittler R. Reactive oxygen signaling and abiotic stress. Physiol Plant. 2008;133(3):481–489.
- Mittler R. Oxidative stress, antioxidants and stress tolerance. Trends Plant Sci. 2002;7(9):405–410.
- Gechev TS, Van Breusegem F, Stone JM, et al. Reactive oxygen species as signals that modulate plant stress responses and programmed cell death. Bioessays. 2006;28(11):1091–1101.
- Mittler R, Vanderauwera S, Gollery M, et al. Reactive oxygen gene network of plants. Trends Plant Sci. 2004;9(10):490–498.
- Li L, Lv M, Li J, et al. Molecular characterisation and function analysis of the rice OsDUF872 family. Biotechnol Biotechnol Equip. 2018;32(4):881–887.
- Li L, Lv M, Li X, et al. The rice OsDUF810 family: OsDUF810.7 may be involved in the tolerance to salt and drought. Mol Biol. 2018;52(4):489–496.