Abstract
Cataract is a multifactorial disease with increasing prevalence with age. Adult diabetics develop cataract earlier. Lens epithelial cells (LECs) exposed to oxidative stress (ROS), increased calcium deposit and membrane damage, undergo apoptosis, which results in lens opacification. Remaining LECs post-surgery leads to posterior capsular opacification (PCO). This study’s aim was to investigate the physiological characteristics of LECs from cataractous diabetic and non-diabetic lenses. Leader cells migration from age-related cataracts started on day 5–7 and from type-2 diabetics on day 8–10. Differences were found in the collective migratory activity and colony formation. On day 22, the colonies formed by LECs from age-related cataracts were three times more, than those formed by diabetic LECs. DNA synthesis and FOXM1 expression occurred in 55.76% of age-related cataract LECs, but only in 33.45% of diabetic LECs. The highest level of reactive oxygen species (ROS) was found in diabetic LECs. Extracellular matrix calcification followed the same pattern as ROS. Among the main reasons for the development of age-related and diabetic cataracts is lens damage due to ROS release and elevated calcium levels. Diabetic LECs experience significantly lower in vitro migration and proliferative activities, compared to LECs from age-related cataracts. This is the first study of its kind in Bulgaria, contributing to the elucidation of the mechanisms of primary and secondary cataractogenesis in diabetic and non-diabetic adults.
Introduction
Cataract, lens opacification, is a leading cause for decreased vision worldwide. It is a multifactorial disease with increasing prevalence with age [Citation1–3]. Adult diabetic patients develop cataract at earlier age compared to non-diabetic patients; however, there is no morphological difference between the age-related cataract and the cataract in adult diabetic patients [Citation3,Citation4]. Oxidative stress, induced by reactive oxygen species (ROS), leads to lens opacification, as the antioxidant activity in the lens declines with age, as well as in diabetic patients [Citation5–8]. Lens epithelial cells (LECs) exposed to oxidative stress, increased calcium deposit and membrane damage, undergo apoptosis [Citation8]. Osmotic stress associated to sorbitol accumulation in the lens of diabetic patients also results in apoptosis [Citation7]. As LECs play a crucial role in maintaining the transparency of the lens, apoptosis of LECs results in lens opacification [Citation8].
LECs are also responsible for the formation of posterior capsular opacification (PCO). Secondary cataract or PCO is caused by proliferation, migration and transdifferentiation of remaining LECs in the capsular bag and it is among the most common complications following cataract surgery [Citation9–11]. There are two main opacification types: regeneratory-capsular pearls, known as ‘Elschnig`s pearls’, presenting migrating and proliferating epithelial cells; and fibrous form, presenting epithelial cells that undergo fibrous metaplasia [Citation12–14]. PCO not only leads to reduced vision, but also makes ophthalmoscopy and laser photocoagulation difficult or even impossible. Hereof, the transparency of the posterior capsule is in great importance especially in diabetic patients. PCO formation among diabetics compared to non-diabetics is a highly controversial subject. Many ophthalmologists have the clinical impression that diabetic patients develop more severe PCO than non-diabetic ones [Citation9,Citation15–17]. Conversely, other studies show similar or even lower incidence of PCO formation among diabetics in comparison to non-diabetics [Citation11,Citation18,Citation19].
The aim of our study was to investigate and compare the fundamental physiological characteristics of LECs from cataractous lenses of non-diabetic and diabetic patients. This contributes to the elucidation of the mechanisms of cataractogenesis and the molecular mechanisms that determine the subsequent occurrence of secondary cataract in diabetic patients.
Subjects and methods
Ethics statement
All patients gave their informed consent. The protocol was approved by the Ethics Committee of The Medical University of Sofia (Bulgaria). The present study adhered to the tenets of the Declaration of Helsinki.
Patients
Trypan blue 0.06% stained and unstained explants of anterior lens capsules (with approximate size of 5.0 mm) and adherent LECs were obtained during cataract surgery by continuous curvilinear capsulorhexis in 33 patients (33 eyes), aged from 55 to 75 years of either sex, having age-related cataract (17 eyes) or type 2 diabetes induced cataract (16 eyes) (). Additional exposure of the internal surface of 6 explants to trypan blue 0.06% was performed in vitro.
Table 1. Number of explants and staining procedure.
Obtaining primary LECs from an anterior capsule
The explants were placed with the epithelial cells at the bottom of 12-well plates (Greiner Bio-One GmbH, Frickenhausen, Germany) in a complete nutrient medium - Dulbecco's Modified Eagle Medium (DMEM, AppliChem GmbH, Darmstadt, Germany), containing 20% fetal bovine serum (FBS, Sigma-Aldrich, USA) and 2 mmol/L glutamine, and incubated at 37 °C and 5% CO2. The complete culture medium was changed every third day of the in vitro cultivation of the explants. Upon reaching about 60% of cell confluency of the primary LECs cultures, the cells were prepared for subsequent studies of colony formation and cell cycle.
Phase-contrast light microscopic observation of LECs
Capsular explants were monitored every 24 h with a 10X lens, Brightfield illumination from Acquisition Mode of the Automated cellular and subcellular imaging system IN Cell Analyzer 60,005% CO2 (GE Healthcare Life Sciences). Capsular explants and the primary LECs that migrated from them were imaged at various time intervals.
Cytochemical labeling of primary LECs with crystal violet
Exponentially growing LECs were washed with PBS, fixed for 10 min at room temperature with 1 vol acetic acid/7 vol methanol (Sigma-Aldrich, USA) and incubated with 0.5% crystal violet solution (crystal violet powder/H20/methanol, Sigma-Aldrich, USA) for 1 h at room temperature, washed vigorously with H2O and allowed to air dry for 2 days. Cytochemically labeled Crystal violet primary LECs were analyzed on an Automated cellular and subcellular imaging system IN Cell Analyzer 6000 - 10X lens and/or 20X lens, Brightfield illumination from Acquisition Mode.
Labeling with 5-bromodeoxyuridine (BrdU) of primary LECs
Exponentially growing LECs were incubated with complete medium containing 10 μmol/L BrdU (Santa Cruz Biotechnology, USA) for 2 h at 37 °C and 5% CO2.
Immunofluorescence labeling of BrdU-labeled primary LECs
After 2 h of incubation with BrdU, cells were washed three times with PBS, fixed for 30 min at room temperature with 2% paraformaldehyde, permeabilized for 10 min at room temperature with 0.5% TrytonX-100, washed three times with PBS, incubated with 2 N HCl for 10 min at room temperature (DNA salting) and with blocking buffer (PBS, 3% BSA, 0.02% Tween20) for 1 h at room temperature. After washing three times with PBS, the cells were incubated for 2 h at room temperature with anti-human BrdU antibody (sc-51,514), washed three times with PBS and incubated for 1 h at room temperature, in the dark, with IgG-FITC antibody (sc-2990). After washing three times with PBS, the cells were incubated for 2 h at room temperature with anti-human FOXM1 antibody (sc-271,746), washed three times with PBS and incubated for 1 h at room temperature, in the dark, with IgG-CFL 555 antibody (sc-362,267). All antibodies used were manufactured by Santa Cruz Biotechnology, USA. After washing three times with PBS, cell nuclei were labeled with DAPI (Thermo Fisher Scientific, USA) for 15 min at room temperature, in the dark. Immunofluorescent-labeled cells were analyzed on an Automated cellular and subcellular imaging system IN Cell Analyzer 6000.
Fluorescent staining of living primary LECs, visualizing calcium deposition in the extracellular matrix and visualizing intracellular oxidative stress (ROS)
Living primary LECs were incubated over night at 37.0 °C in DMEM culture medium containing 20 µmol/L xylenol orange (XO). Incubation was carried out for 45 min at 37.0 °C in DMEM culture medium containing 2 µg/mL DCFDA (2′,7′-dichlorofluorescein diacetate). The samples underwent fixation for 20 min at room temperature with 2% paraformaldehyde in the dark and were stained with Hoechst for 10 min at room temperature in the dark. LECs were washed three times for 10 min with PBS. Stained LECs were analyzed on an Automated cellular and subcellular imaging system IN Cell Analyzer 6000.
Image-based high content screening analysis
Microscopic images were obtained with a 20× lens and 405-nm, 561-nm, 488-nm lasers excited by DAPI-labeled nuclei, IgG-CFL 555-labeled FOXM1 and, respectively, IgG-FITC-labeled BrdU, which was incorporated into the DNA nuclei.
The area of the whole well, for each of the experimental conditions, was microscopically analyzed by Image-based high content screening: an algorithm was applied to calculate the 555-, 488- and 405-fluorescent radiation of the cells occupying the area.
Statistical analysis
Data obtained from Image-Based High Content Screening analyzes were analyzed by Student's test and one-way analysis of variance (ANOVA) conducted on SigmaPlot Version 12.0 (Systat Software, San Jose, CA, USA). Differences were considered statistically significant at p < 0.05 (*).
Results and discussion
LECs migratory, proliferative and differentiation activities
Migration of primary LECs from all explants was observed (), but the latent period (lag phase) after which migration began was different in LECs isolated from type 2 diabetic patients and LECs isolated from patients with age-related cataract. The migration of the first cells (leader cells) from the explants of patients with age-related cataracts was found on day 5–7 of the in vitro culturing (mean = 5.875, n = 8), and on day 8–10 (mean = 8.875, n = 8) from the in vitro cultivation of explants from type 2 diabetic patients. This difference in the latent period between the 2 groups (diabetic and non-diabetic) was not affected by the in vivo staining with trypan blue.
Figure 1. Primary LECs cultures isolated from anterior lens capsule from type 2 diabetic cataract (A) and from age-related cataract (B).
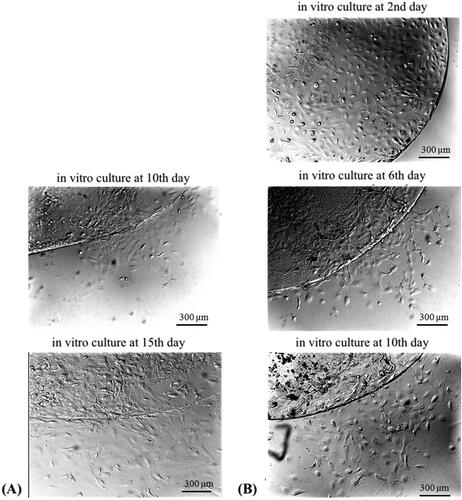
The migration of the first cells from the explants from patients with age-related cataracts, found on or about the 6th day of the in vitro cultivation (), is a confirmation of the existing literature data [Citation14]. To our knowledge, this is the first report of the initial in vitro migration activity of leader cells from the anterior capsule of type 2 diabetic cataract patients. The obtained results enrich the knowledge of the cell-physiologically based mechanisms of capsular opacification.
The extracellular matrix (ECM) is essential for normal cell function like migration, adhesion, differentiation, as well for tissue organization and structure [Citation20,Citation21]. Single cell and collective cell migrations are dependent on ECM integrity [Citation22]. Diabetes mellitus negatively remodulates the ECM and compromises ECM-cell and cell-to-cell communications [Citation21,Citation23].
Trypan blue is a hydrophilic dye which usually cannot penetrate through intact cell membranes and is used to stain the anterior lens capsule during cataract surgery [Citation24]. Some authors report, that trypan blue is cytotoxic to LECs, therefore it can reduce the occurrence of PCO [Citation25,Citation26]. According to our study, the lack of differences in the migration activities of leader cells that migrated from in vivo outer surface trypan blue stained 0.06% and unstained explants suggests that the outer surface intraoperative staining with trypan blue 0.06% of the anterior capsule does not affect the epithelial capacity of LECs.
Subsequent collective migratory activity (pro-collective movement) and proliferative activity (colony formation) were observed every 24 h, and the culture time required to reach 60% cellular confluence of primary LECs was determined (). Differences were found in the collective migratory activity and in the colony formation of age-related cataract LECs and type 2 diabetes LECs. On day 22 of in vitro culturing of the patients’ explants, the number of colonies formed by LECs from age-related cataracts was three times greater than the number of colonies formed by LECs from type 2 diabetic patients. Along with significantly lower colony formation, a morphological change in LECs from type 2 diabetic cataract was observed. This morphological change was expressed in an altered cytoplasm/nucleus ratio.
Figure 2. Crystal violet labeled colonies on day 22 from in vitro culturing of LECs isolated from anterior lens capsule of age-related cataract (A) and type 2 diabetic cataract (B).
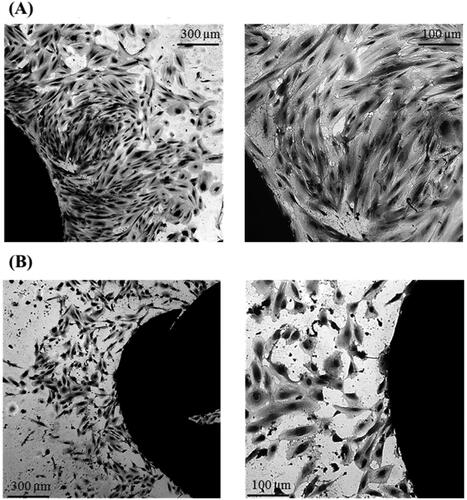
The observed difference in colony formation () could be attributed to different proliferative and migratory activities of LECs isolated from the two types of cataracts. This was demonstrated by the immunofluorescence study of BrdU incorporation into newly synthesized DNA and the expression of the transcription factor FOXM1 (). Image-based high content screening analyzes showed that, on average, 55.76% (n = 4, p < 0.05) of age-related cataract LECs contained newly synthesized DNA and expressed FOXM1, whereas only 33.45% on average (n = 4, p < 0.05) of LECs of type 2 diabetic patients were proliferatively active (). FOXM1 regulates the expression of genes encoding proteins in the mitotic spindle. This transcription factor is a reliable marker for cell proliferation, as it promotes G1/S- and G2/M-transitions [Citation27].
Oxidative stress analysis
Based on the triple fluorescence study (), a quantitative analysis was performed to calculate the mean statistical extracellular calcium deposit and intracellular oxidative stress relative to a single cell ().
Figure 4. Triple fluorescence study of LECs from internal surface trypan blue stained anterior lens capsule in a patient with age-related cataract (A); LECs from internal surface trypan blue stained anterior lens capsule in a type 2 diabetic cataract patient (B); LECs from outer surface trypan blue stained anterior lens capsule in a patient with age-related cataract (C); LECs from outer surface trypan blue stained anterior lens capsule in a type 2 diabetic cataract patient (D).
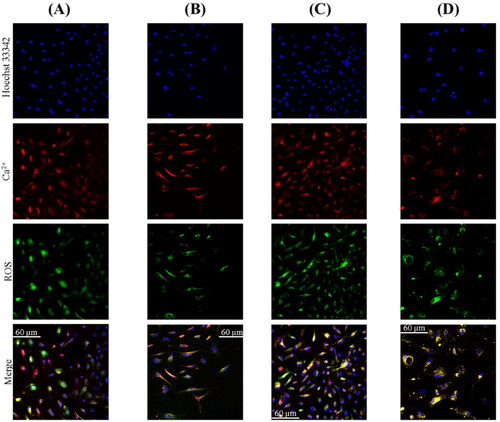
Figure 5. Relative intracellular ROS levels and extracellular matrix calcification of living LECs isolated from anterior lens capsules of LECs from internal surface trypan blue stained anterior lens capsule in type 2 diabetic cataract patients (A); LECs from internal surface trypan blue stained anterior lens capsule in patients with age-related cataract (B); LECs from outer surface trypan blue stained anterior lens capsule in patients with age-related cataract (C); LECs from outer surface trypan blue stained anterior lens capsule in type 2 diabetic cataract patients (D); LECs from outer surface trypan blue stained anterior lens capsule in patient with colon cancer (E).
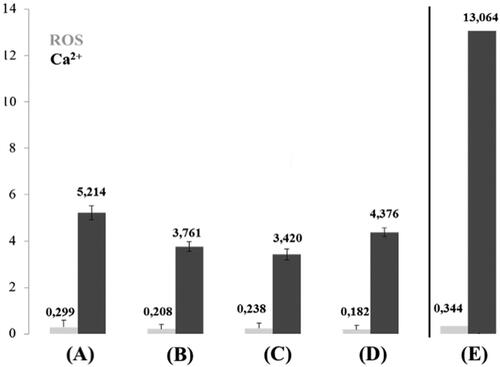
The highest level of ROS (excluding the colon cancer patient mentioned below) was found in LECs from internal surface trypan blue stained anterior lens capsule in type 2 diabetic patients: 0.299. Lower level of ROS (0.208) was found in LECs of internal surface trypan blue stained anterior lens capsule in patients with age-related cataracts. ROS in LECs from outer surface trypan blue stained anterior lens capsule in patients with age-related cataract was 0.238, and in LECs from outer surface trypan blue stained anterior lens capsule in type 2 diabetic patients 0.182. The level of extracellular matrix calcification followed the same pattern as ROS: internal surface trypan blue stained anterior lens capsule in type 2 diabetic patients was 5.214; in LECs from outer surface trypan blue stained anterior lens capsule in patients with age-related cataract 3.420, in LECs from internal surface trypan blue stained anterior lens capsule in patients with age-related cataract 3.761; in LECs of outer surface trypan blue stained anterior lens capsule in patients with type 2 diabetes 4.376. Extremely high level of ROS and ECM calcification was observed in a patient with colon cancer ().
ROS play a significant role in inducing apoptosis in physiological conditions and in pathology. Mitochondria are the main source and target structure of ROS [Citation28]. Oxidative stress through ROS and induced apoptosis, together with reduced antioxidant activity, are responsible for the development of various types of cataracts [Citation8]. Calcium concentration and deposition rise with an increase in oxidative stress and reduction in antioxidant status, resulting in apoptosis. Regarding the patient with colon cancer (), the high ROS and ECM calcification might be associated with the carcinogenesis [Citation29]. Physiological ECM calcium deposition is observed only in the skeletal system [Citation30], thus ECM calcification of LECs is pathological. Higher reduction in antioxidant activity is reported in diabetic cataract patients compared to patients with age-related cataract [Citation5,Citation31]. The highest calcium deposition levels in LECs in the present study were detected exactly in the group of diabetic patients. Furthermore, higher calcium deposition levels were found in the internal surface trypan blue stained explants, compared to only outer surface stained group, both from diabetic patients. Direct trypan blue staining of LECs (explants internal surface) in the group of age-related cataract patients also led to increased calcium deposition compared to only outer surface stained explants. However, the ROS levels were similar. We speculate that lipid peroxidation damage to plasma membranes of diabetic LECs makes them partially permeable to trypan blue. According to this finding and recently published data [Citation32], direct exposure of LECs to trypan blue might have negative effect on their physiological integrity.
The observed increased oxidative stress among diabetics leads to cellular and ECM damage, which is a possible cause for the reduced proliferative and migratory activities, as well as for the reduced colony formation. This in vitro finding might explain the thesis for reduced incidence of PCO among diabetic patients [Citation11,Citation18,Citation19].
Our study has some limitations. First, in included a small cohort of patients. Second, the diabetic status of the patients was not evaluated. Within the limitations of this study, our results showed that type 2 diabetes negatively affects cell physiology, contributing to cataract formation.
Conclusions
One of the main reasons for the development of age-related and diabetic cataracts is lens damage due to the release of ROS and the associated elevated levels of calcium. In this study, LECs of type 2 diabetic cataract patients were characterized by significantly lower in vitro migration and proliferative activities as compared to the in vitro activities of LECs from age-related cataracts. LECs migratory characteristics were not affected by in vivo outer surface trypan blue 0.06% staining. However, the higher levels of ROS in direct exposure of LECs to trypan blue, especially in diabetic patients, might damage them.
The observations reported here could contribute to the elucidation of the mechanisms of primary and secondary cataractogenesis in diabetic and non-diabetic adults.
Data availability statement
The data supporting the findings of this study are available from the authors upon reasonable request.
Disclosure statement
The authors have no conflicts of interest to declare.
Additional information
Funding
References
- Nemet AY, Vinker S, Levartovsky S, et al. Is cataract associated with cardiovascular morbidity? Eye (Lond)). 2010;24(8):1352–1358.
- Pessoa R, Lira C, Nascimento MA, et al. Incidence of preoperative high blood pressure in cataract surgery among hypertensive and normotensive patients. Indian J Ophthalmol. 2010; 58(6):493–495.
- Prokofyeva E, Wegener A, Zrenner E. Cataract prevalence and prevention in Europe: a literature review. Acta Ophthalmol. 2013;91(5):395–405.
- Bron AJ, Sparrow J, Brown NAP, et al. The lens in diabetes. Eye. 1993;7(2):260–275.
- Deepa K, Goud BM, Suma M, et al. Antioxidant and calcium levels in mature and immature diabetic cataract lens. Int J Life Sci. 2012; 6(1):56–60.
- Nita M, Grzybowski A. The role of the reactive oxygen species and oxidative stress in the pathomechanism of the age-related ocular diseases and other pathologies of the anterior and posterior eye segments in adults. Oxid Med Cell Longev. 2016;2016:3164734–3164723.
- Pollreisz A, Schmidt-Erfurth U. Diabetic cataract-pathogenesis, epidemiology and treatment. J Ophthalmol. 2010;2010:608751.
- Sreelakshmi V, Abraham A. Age related or senile cataract: pathology, mechanism and management. Austin J Clin Ophthalmol. 2016;3(2):1067.
- Ebihara Y, Kato S, Oshika T, et al. Posterior capsule opacification after cataract surgery in patients with diabetes mellitus. J Cataract Refract Surg. 2006; 32(7):1184–1187.
- Nibourg LM, Gelens E, Kuijer R, et al. Prevention of posterior capsular opacification. Exp Eye Res. 2015;136:100–115.
- Praveen MR, Vasavada AR, Shah GD, et al. A prospective evaluation of posterior capsule opacification in eyes with diabetes mellitus: a case–control study. Eye. 2014;28(6):720–727.
- Apple DJ, Solomon KD, Tetz MR, et al. Posterior capsule opacification. Surv Ophthalmol. 1992; 37(2):73–116.(92)90073-3.
- Pandey SK, Apple DJ, Werner L, et al. Posterior capsule opacification: a review of the aetiopathogenesis. Indian J Ophthalmol. 2004;52(2):99–112.
- Sundelin K, Petersen A, Soltanpour Y, et al. In vitro growth of lens epithelial cells from cataract patients—association with possible risk factors for posterior capsule opacification. Open Ophthalmol J. 2014;8:19–23.
- Hayashi K, Hayashi H, Nakao F, et al. Posterior capsule opacification after cataract surgery in patients with diabetes mellitus. Am J Ophthalmol. 2002;134(1):10–16.
- Ionides A, Dowler JGF, Hykin PG, et al. Posterior capsule opacification following diabetic extracapsular cataract extraction. Eye. 1994;8(5):535–537.
- Wu S, Tong N, Pan L, et al. Retrospective analyses of potential risk factors for posterior capsule opacification after cataract surgery. J Ophthalmol. 2018; 2018:1–7.
- Elgohary MA, Dowler JG. Incidence and risk factors of Nd:YAG capsulotomy after phacoemulsification in non-diabetic and diabetic patients. Clin Exp Ophthalmol. 2006;0(0):060721075142001–060721075142034.
- Zaczek A, Zetterström C. Posterior capsule opacification after phacoemulsification in patients with diabetes mellitus. J Cataract Refract Surg. 1999;25(2):233–237.
- Islam Md. S., editor. Advances in experimental medicine and biology. Calcium signaling. Cham: Springer International Publishing; 2020. Vol. 1131.
- Law B, Fowlkes V, Goldsmith JG, et al. Diabetes-induced alterations in the extracellular matrix and their impact on myocardial function. Microsc Microanal. 2012;18(1):22–34.
- Trepat X, Chen Z, Jacobson K. Cell migration. In Terjung R, editor. Comprehensive physiology. Hoboken, NJ, USA: John Wiley & Sons, Inc.; 2012. p. c110012.
- Huang Y, Kyriakides TR. The role of extracellular matrix in the pathophysiology of diabetic wounds. Matrix Biol Plus. 2020;6–7:100037.
- Badaro E, Novais EA, Penha FM, et al. Vital dyes in ophthalmology: a chemical perspective. Curr Eye Res. 2014; 39(7):649–658.
- Melendez RF, Kumar N, Maswadi SM, et al. Photodynamic actions of Indocyanine Green and Trypan Blue on human lens epithelial cells in vitro. Am J Ophthalmol. 2005;140(1):132–134.
- Nanavaty MA, Johar K, Sivasankaran MA, et al. Effect of trypan blue staining on the density and viability of lens epithelial cells in white cataract. J Cataract Refract Surg. 2006;32(9):1483–1488.
- Wierstra I, Alves J. FOXM1, a typical proliferation-associated transcription factor. Biol Chem. 2007;388(12):1257–1274. doi: 10.1515/BC.2007.159.PMID:18020943.
- Simon H-U, Haj-Yehia A, Levi-Schaffer F. Role of reactive oxygen species (ROS) in apoptosis induction. Apoptosis. 2000;5(5):415–418.
- Perše M. Oxidative stress in the pathogenesis of colorectal cancer: cause or consequence? Biomed Res Int. 2013;2013:725710–725719.
- Schinke T, McKee MD, Karsenty G. Extracellular matrix calcification: where is the action? Nat Genet. 1999; 21(2):150–151.
- Görlach A, Bertram K, Hudecova S, et al. Calcium and ROS: a mutual interplay. Redox Biol. 2015;6:260–271.
- Sinumol S, Jayalakshmy PS, Sreedharan T, et al. Effect of intraoperative trypan blue on lens epithelial cells—histomorphological analysis. Saudi J Ophthalmol. 2019; 33(1):18–23.