Abstract
Avenin-like storage proteins affect the rheological properties and processing quality of common wheat. Triticum urartu, the A-genome donor of polyploid wheat, shows abundant variation in its glutenin subunits. In this study, the variability in avenin-like b genes was evaluated in 74 T. urartu accessions. High levels of polymorphism were demonstrated in the analyzed sequence, resulting in the discovery of 18 novel TuALPb7A alleles (TuALPb7A-A–TuALPb7A-R). There were nucleotide sequence differences in 18 locations, which, due to synonymous mutations, resulted in amino acid changes. The polymorphic sites were located in the N-terminus, repetitive region (Left), repetitive region (Right) and C-terminus domains. The position of some cysteine residues was different in T. urartu and Aegilops tauschii accessions. Phylogenetic analysis divided the avenin-like b (ALPb) genes into two groups. The ALPb-7A alleles, containing 18 TuALPb7As, were distributed in the first group, whereas the ALPb-4A alleles were present in another. Avenin-like proteins encoded by four alleles (TuALPb7A-G, TuALPb7A-O, TuALPb7A-K and TuALPb7A-C) were expressed on a large scale and purified to estimate the effect on dough mixing properties. Mixograph testing demonstrated that these proteins could improve the functional properties of dough, including the mixing time, peak dough resistance and breakdown in resistance. The avenin-like b genes hold rich genetic alleles in T. urartu, and can improve the functional properties of dough. The new favourable alleles discovered will provide breeding materials and a theoretical basis for wheat quality improvement.
Introduction
Avenin-like proteins are a sub-family of the wheat prolamin superfamily and have two isoforms, type-a and type-b. The avenin-like gene family were studied in detail by Kan et al. [Citation1]. Generally, avenin-like proteins are associated with the type-b isoform [Citation1]. Type-b avenin-like genes are from a multigene family [Citation2,Citation3]. Using proteomics, type-b avenin-like protein was found in the glutenin fraction of durum wheat [Citation4] and the same researchers suggested that this protein may be integrated in the glutenin polymer due to its high content of cysteine (cys) residues. The type-b protein contains 284 amino acid residues with 18 (b-2) or 19 (b-1, b-3 and b-4) cys residues. Cys residues are more conserved in the repetitive region than at the N- or C-terminals [Citation1].
Recently, three avenin-like b protein-encoding genes, TaALP-4A, TaALP-7A and TaALP-7D, were isolated and mapped to chromosomes 4AL, 7AS and 7DS [Citation3]. Wheat grain avenin-like proteins have positive effects on dough quality and antifungal activities [Citation3,Citation5–7]. The allelic effects of TaALP-7A were estimated in some commercial wheat cultivars and TaALP-7A1, as a positive allele, was useful in improving the quality of wheat dough [Citation3]. A total of 13 TaALPb7Ds were identified and analysed in 108 Aegilopstauschii accessions to reveal the evolutionary relationships and the possibility of using avenin-like b genes for wheat improvement in our lab [Citation8]. There are several approaches to estimating the function of a single glutenin subunit in wheat, including genetic transformation [Citation5,Citation9], development of recombinant inbred lines [Citation10] and near-isogenic lines [Citation11] containing the glutenin subunit and micro mixograph tests of glutenin produced in a bacterial expression system [Citation12–14]. The latter method is more time-consuming and labor-intensive than the other techniques. However, incorporation of purified avenin-like b proteins, produced in vitro, into wheat flour showed a positive correlation between avenin-like b proteins and dough strength using 2 g mixograph tests [Citation12]. Additionally, the overexpression of avenin-like b proteins demonstrated their value in improving dough elasticity, flour mixing properties, resistance to extension and overall quality [Citation5]. Consequently, the identification and cloning of novel avenin-like b genes will not only enrich the avenin-like b protein types, but also help to improve wheat quality.
Diploid Triticum, as a gene pool, not only harbours tremendous genetic variability for broadening the genetic basis of common wheat, but also provides good genetic resources for scientific research and breeding in wheat. Triticum urartu (AA, 2n = 2x = 14) is the A-genome progenitor of emmer and common wheats and has a wide geographical distribution and large genetic variation [Citation15]. Recently, the majority of genes and alleles were identified in T. urartu [Citation16,Citation17]. Consequently, some new genetic resources were discovered and these genes now play a vital role in genetic exploitation and the evaluation of genetic relationships. However, there was no information forthcoming about TuALPb7A variations and functional properties in T. urartu. In this study, 74 T. urartu accessions were chosen to analyze the variation and functional properties of TuALPb7As, to detect a beneficial TuALPb7As gene for wheat improvement.
Materials and methods
Plant materials
A total of 74 T. urartu accessions were obtained from the United States Department of Agriculture (http://www.ars-grin.gov/). These accessions had been collected from six countries covering the area of natural distribution for T. urartu. The longitude, latitude and altitude data about the collection sites of these accessions were downloaded from http://www.ars-grin.gov/ (). Flour from the wheat cultivar Gaoyuan 448 was selected as the base flour.
Table 1. Effects of purified avenin-like proteins on the mixograph parameters.
Isolation of avenin-like b genes
Genomic DNA from the 74 T. urartu accessions was isolated from young leaves of 10-day-old seedlings using the cetyltrimethylammonium bromide (CTAB) extraction method [Citation18]. Three pairs of primers, TaALP (Ave-F: 5′-ATGAAGGTCTTCATCCTGGCTC-3′; Ave-R:5′-CTAGCACGCACCACCAGT-3′), TaALPb7A (Ave7A-F:5′-ATGCCAACATCAACAACCG-3′; Ave7A-R: 5′-TAGTAC GCACCACCAGGGTAA-3′) and TaALPb4A (Ave4A-F: 5′-TCGGACAATACCAA CAACAG-3′; Ave4A-R: 5′-TCTAGCATGCACCACTAGTGC-3′) [Citation3], were used to amplify full-length avenin-like b genes and identify TuALP7A and TuALP4A loci from different accessions by polymerase chain raction (PCR). PCR amplifications were conducted in 20 μL reactions using 200 ng of template DNA, 0.2 mmol/L of each dNTP, l μmol/L of each of primer, 2 μL of 5 × PCR buffer and 2.5 U of high fidelity phusion DNA polymerase (Thermo Fisher Scientific, Waltham, MA, USA). The reaction mixture was heated to 94 °C for 5 min to denature the DNA, cycled 35 times at 94 °C for 50 s, 58 °C for 45 s and 72 °C for 2 min, then extended for 10 min at 72 °C (Applied Biosystems thermal cycler; 2720 Mini AMP).
The PCR products were confirmed by electrophoresis in 1.0% agarose gel and the bands of expected size were purified from the gel using an Easy Pure Quick Gel Extraction Kit. The targeted DNA fragments were ligated into PGEM-T easy vectors (Promega, Madison, WI, USA), which were transformed into Escherichia coli DH 5a competent cells. The positive clones were picked out and sent to a commercial company (Huada Gene, China) for sequencing.
Expression and purification of avenin-like b protein
The TuALPb7As were cloned using the expression primers (Aveex-F: 5′-CGCGGATCCCAGTTGGAAACCACATGT-3′ and Aveex-R: 5′-CCCAAGCTTC TAGCACGCACCACCAGT-3′). The PCR product was purified and digested with CpoI and NotI, before being ligated into pET-SUMO vectors that were linearized with CpoI and NotI. The identity of the recombinant pET-SUMO/avenin-like plasmid was tested by sequencing.pET-SUMO/avenin-like plasmids were transferred into E. coli BL21 (DE3) pLysS (Merck, USA) cells and then inoculated into LB liquid medium with ampicillin (100 mg/mL) according to the volume ratio of culture to LB liquid medium of 1:100. The cells were cultivated at 37 °C on a shaker until the A600 reached 0.6 and then the expression of avenin-like protein was induced with isopropyl-b-D-thiogalactoside (IPTG, 1 mmol/L) at 37 °C for 3–4 h. Cells were harvested after centrifugation and resuspended in NTA-0 (pH = 8.0, 0.02 mol/L Tris, 0.5 mol/L NaCl, 10% glycerin) and incubated on ice for 30 min. The thallus precipitate was disrupted by sonification and the precipitate was collected after centrifugation. Avenin-like proteins were identified by sodium dodecyl sulfate polyacrylamide gel electrophoresis (SDS-PAGE).
Inclusion bodies were purified using the following steps. The precipitate collected in the last step was resuspended in NTA-0 containing dithiothreitol (DTT) and disrupted by sonification until the supernatant became transparent. The precipitate was recollected and resuspended in PBS buffer (140 mmol/L NaCl, 2.68 mmol/L KCl, 10.14 mmol/L Na2HPO4, 1.76 mmol/L KH2PO4) and disrupted by sonification. The precipitate was collected for a third time and resuspended in 6 mol/L guanidine hydrochloride solution (pH = 9.0) with DTT and vibrated at 37 °C for 3 h. The supernatants were collected and loaded into a dialysis bag. The bag was soaked in PBS buffer overnight. Finally, the precipitate remaining in the dialysis bag was successively passed through a 0.22 mm filter and a Ni-NTA column with a flow velocity of 1 mL/min. The Ni-NTA column was washed with NTA-0 buffer, 20 mmol/L imidazole, 60 mmol/L imidazole, 200 mmol/L imidazole and 500 mmol/L imidazole in succession. The eluants were collected separately and tested with G250 solution (0.05% ethyl alcohol, 0.1% phosphoric acid, 0.12 mmol/L Xylene brilliant cyaninG). The purified protein was freeze dried and stored at −20 °C until use.
Incorporation and 10 gram mixograph tests
A reversible reduction/oxidation process was employed to incorporate polypeptides into glutenin [Citation9]. Base flour (10 g; Gaoyuan 448) and avenin-like protein (125 mg) were mixed with 2 mL water containing reducing agent (50 µg/mL DTT) for 1 min and the dough was allowed to react for 4 min. Next, 100 µL of oxidizing agent (200 μg/mL KIO3) was added to the dough. The dough was mixed for 1 min and left to react for 5 min. Finally, the dough was mixed for a further 11 min. The same procedure was carried out in another independent experiment in which water, reducing and oxidizing agents were added to 10.125 g Gaoyuan 448. All the mixing tests were carried out three times.
Bioinformatics analysis
The assembly and alignments of avenin-like b gene and protein sequences were conducted using the DNAMAN version 6.0 software package. The phylogenetic tree of nucleotides was constructed with MEGA5 software using the neighbor-joining method [Citation19]. The geographic distribution of avenin-like b genes was visualized with a Venn diagram (http://bioinfogp.cnb.csic.es/tools/venny_old/index.html) and the Meteorological Data Information System [Citation20].
Results and discussion
Characterizationof avenin-like b geneTuALPb7As in T. urartu accessions
In total, 18 novel avenin-like b gene TuALPb7A alleles (TuALPb7A-A–R) were isolated from 74 T. urartu accessions (Supplemental Table S2). Alleles TuALPb7A-B, TuALPb7A-A and TuALPb7A-N were observed in 24, 19 and 7 T. urartu accessions, respectively. The remaining alleles were identified in no more than three T. urartu accessions each. Among these alleles, base mutations and deletions arose from nucleotide sequence differences (Supplemental Table S3). TuALPb7A-C and TaALPb7A-M–R had three-base deletions at 107–109 bp compared with other alleles (Supplemental Table S3). Single-nucleotide substitutions existed at the rest of the polymorphic loci. These alleles were all located on chromosome 7A using the specific markers (Ave7A-F/Ave7A-R and Ave4A-F/Ave4A-R) exploited by Chen et al. [Citation3]. All of the alleles encoded 284 amino acid residues, except TuALPb7A-C and TaALPb7A-M–R (). The 18 TuALPb7A alleles encoded 14 proteins with alleles TuALPb7A-A and TuALPb7A-H, TuALPb7A-B and TuALPb7A-J, TuALPb7A-M andTuALPb7A-R and TuALPb7A-N and TuALPb7A-Q encoding the same proteins, respectively. The polymorphic loci were not all located in the conserved cys residues and N-terminal domain. The position and quantity of cys residues of TuALPb7A proteins were conserved in T. urartu. All proteins contained 18 cys residues, which were located in the N-terminal domain (position 24), Rep-region L domain (positions 31, 49, 57, 75, 82, 83, 95, 137, 145, 166 and 174), Rep-region R domain (positions 192, 199, 200 and 212) and C-terminal domain (positions 257 and 265).
The position of cys residues in T. urartu and Ae. tauschii
The position of cys residues was different among T. urartu, Ae. tauschii and T. aestivum, while the total number was the same. The cys residue positions were in the N-terminal domain (position 24), Rep-region L domain (positions 49, 57, 75, 82, 83, 95, 137, 145, 166 and 174), Rep-region R domain (positions 192, 199, 200 and 212) and C-terminal domain (positions 257, 265 and 284) in the Ae. tauschii accession [Citation8]. The cys in the N-terminal domain was located in position 31 in T. urartu, while the position was taken by serine in the Rep-region L domain. Position 284 was tyrosine in T. urartu, whereas cys was in that position in the C-terminal domain of Ae. tauschii and T. aestivum (). Site-directed mutagenesis was employed to get a mutant in which one tyrosine codon at the C-terminal is substituted by a cysteine codon of avenin-like b gene, and the specific cysteine residues could improve the flour quality [Citation21]. In this study, the position of the cys residues was different in T. urartu and in Ae. tauschii, which probably has a good impact on flour quality.
In this study, 18 novel TuALPb7A alleles (TaALPb7A-A–R) were characterized in wild populations of T. urartu. All of the alleles encoded proteins with 284 or 283 amino acids. Single nucleotide polymorphisms (SNPs) at 24 sites and one trinucleotide deletion existed in these alleles. They were detected within the signal, Repetitive region (left), Repetitive region (right) and C-terminal domains, but outside the cys and N-terminal domains. These SNPs and the trinucleotide deletion could cause partial single amino acid polymorphisms, which can lead to some amino acid changes. Glutamine was deleted in TuALPb7A-C, TuALPb7A-M–R and this could affect the protein folding and influence the flour quality [Citation22,Citation23]. Previous studies predicted that cys residues could form seven pairs of inter-molecular disulfide bonds, which are thought to create a continuous network in dough and participate in the formation of glutenin polymers, thus having a positive effect on wheat processing quality [Citation2,Citation12,Citation23]. In this study, all alleles contained 18 cys residues, but the positions of these (position 31, 284) varied between T. urartu and Ae. tauschii, while the number of residues remained the same. The next study will focus on whether these positional differences affect wheat dough quality. Finally, these novel alleles identified in the present work further enriched our knowledge of avenin-like b gene types and may be used in work to improve wheat quality.
Phylogenetic relationships and geographical distribution of TuALPb7As
To achieve a better understanding of the evolutionary relationship among the 18 TuALPb7As isolated from T. urartu in this study, a phylogenetic tree was constructed from the base sequences of the alleles and avenin-like b genes from Triticum aestivum and Triticum monococcum. These alleles could be clustered into two groups in the phylogenetic tree. TuALPb7As of T. urartu were present in the same group that contained all the avenin-like b alleles of T. monococcum and ALPb-7A of T. aestivum (). While the avenin-like b gene ALPb-4A of T. aestivum were in the other clades. The geographic distribution of TuALPb7A genes was analyzed to better exploit and conserve the T. urartu germplasm. TuALPb7A-A–R were all distributed in the accessions from the Middle East and West Asian countries (; ). TuALPb7A-A was the most predominant and widely distributed allele (), in contrast to the other alleles. Turkey represented the region of greatest diversity with 11 of 18 novel TuALPb7A alleles observed there. Moreover, TuALPb7A-C, D, E, G and H were unique to Turkey ().
Function verification of TuALPb7A alleles
The recombinant vector, pET-SUMO/avenin-like plasmid, was constructed and transformed into E. coli BL21 (DE3). In view of synonymous mutations and cluster resultsfrom amino acid sequencing, we selected avenin-like proteins encoded by four TuALPb7A alleles (TuALPb7A-G, TuALPb7A-O, TuALPb7A-K and TuALPb7A-C) for large scale expression induced by IPTG. The SUMO tag was approximately 16 kDa in size and the molecular masses of avenin-like proteins encoded by these alleles were about 32 kDa. Therefore, the molecular mass of recombinant proteins containing the SUMO tag were approximately 48 kDa, which matched the results of this study (). Then, these recombinant proteins were purified to estimate the effect on flour quality. In addition, TaALPb-7A1 had been isolated from bread wheat [Citation3]. In this study, the recombinant protein containing TaALPb-7A1 was also purified and the allelic effect was compared with the four alleles TuALPb7A-G, TuALPb7A-O, TuALPb7A-K and TuALPb7A-C.
Figure 4. Identification of expression of avenin-like b protein and purified protein by SDS-PAGE. Lane M: Marker (116.0/66.2/45.0/35.0/25.0 kDa, Shanghai Dingjie Biotechnology Co., LTD); Lane 1: expression of supernatant protein induced by IPTG; Lane 2: expression of recombinant protein induced by IPTG; Lane 3: supernatant protein after purification; Lane 4: recombinant protein after purification; Lane 5: Renatured avenin-like b protein before purification; Lane 6: Renatured avenin-like b protein after purification.
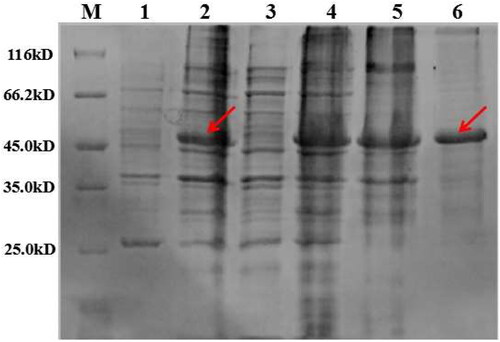
Mixograph analyses were conducted with the base flour (Gaoyuan 448, control) and the base flour containing avenin-like b proteins (). When DTT (reducing agent) and KIO3 (oxidizing agent) were added to the base flour, the mixograph parameters were not significantly different from the control (). When five avenin-like proteins were incorporated into the base flour, significant differences from the control were measured for mixing time (MT), dough resistance (PR) and resistance breakdown (RBD). However, there were no significant differences among the five alleles.
To estimate the functional properties of glutenin subunits in wheat flour, a large expression system was used to produce enough purified protein and a reconstitution experiment that incorporated glutenin into flour using reducing and oxidizing agents were employed [Citation24,Citation25]. This method has also been used to evaluate the dough mixing characteristics of cys-rich avenin-like proteins [Citation12]. In this study, mixograph tests showed that avenin-like proteins could incur a favourable effect on the properties of dough. The result was consistent with a previous study [Citation12]. The conclusions were proven by the genetic transformation method [Citation5]. One important reason for the improvement in dough characteristics was the rich presence of cys residues in avenin-like proteins [Citation5,Citation12]. In addition, TaALP-7A1 has a significant association with better dough quality in 102 Chinese wheat cultivars [Citation3]. Using a mixograph test, the contribution to dough mixing properties of four alleles identified in this study was the same as that of TaALP-7A1. Thus, avenin-like proteins are significant to the mixing properties of wheat dough and could further be used to improve wheat processing practices.
Conclusions
In this study, genetic diversity analysis of avenin-like b protein in 74 T. urartu was carried out, and 18 novel alleles were detected. Their differences mainly occurred in single base mutation and tribase deletion. The position of Cys was changed, which would affect the adding quality of wheat flour. When the four alleles were expressed in large quantities, the mixed experiment showed that these proteins could improve the dough properties. This result can provide materials and theoretical basis for wheat quality breeding.
Supplemental Material
Download PDF (253.4 KB)Disclosure statement
No potential conflict of interest was reported by the authors.
Data availability statement
The data that support this study are available from the corresponding authors upon reasonable request.
Additional information
Funding
References
- Kan Y, Wan Y, Beaudoin F, et al. Transcriptome analysis reveals differentially expressed storage protein transcripts in seeds of Aegilops and wheat. J Cereal Sci. 2006;44(1):75–85.
- Chen P, Wang C, Li K, et al. Cloning, expression and characterization of novel avenin-like genes in wheat and related species. J Cereal Sci. 2008;48(3):734–740.
- Chen XY, Cao XY, Zhang YJ, et al. Genetic characterization of cysteine-rich type-b avenin-like protein coding genes in common wheat. Sci Rep. 2016; 6:30692
- De Caro S, Ferranti P, Addeo F, et al. Isolation and characterization of Avenin-like protein type-B from durum wheat. J Cereal Sci. 2010;52(3):426–431.,
- Ma F, Li M, Yu L, et al. Transformation of common wheat (Triticum aestivum L.) with avenin-like b gene improves flour mixing properties. Mol Breed. 2013; 32:853–865.
- Zhang YJ, Hu X, Islam S, She MY, et al. New insights into the evolution of wheat avenin-like proteins in wild emmer wheat (Triticum dicoccoides). Proc Natl Acad Sci U S A. 2018;115(52):13312–13317.
- Zhang YJ, Hu X, Juhasz A, et al. Characterising avenin-like proteins (ALPs) from albumin/globulin fraction of wheat grains by RP-HPLC, SDS-PAGE, and MS/MS peptides sequencing. BMC Plant Biol. 2020;20(1):45
- Cao D, Wang HX, Zhang B, Liu BL, et al. Genetic diversity of avenin-like b genes in Aegilops tauschii Coss. Genetica. 2018;146(1):45–51.
- Blechl A, Lin J, Nguyen S, et al. Transgenic wheats with elevated levels of dx5 and/or dy10 high-molecular-weight glutenin subunits yield doughs with increased mixing strength and tolerance. J Cereal Sci. 2007; 45(2):172–183.
- Nieto-Taladriz MT, Perretant MR, Rousset M. Effect of gliadins and hmw and lmw subunits of glutenin on dough properties in the f 6, recombinant inbred lines from a bread wheat cross. Theor Appl Genet. 1994; 88:81–88.
- Yamauchi H, Ito M, Nishio Z, et al. Effect of high- molecular-weight glutenin subunits on the texture of yellow alkaline noodles using near-isogenic lines. FSTR. 2007;13(3):227–234.
- Chen P, Li R, Zhou R, et al. Heterologous expression and dough mixing studies of a novel cysteine-rich avenin-like protein. Cereal Res Commun. 2010;38(3):406–418.
- Ferrante P, Gianibelli MC, Larroque O, et al. Considerations about the effect of incorporation of two rare LMW-GS in durum wheat in comparison to bread wheat doughs. Options Mediterraneennes A Seminaires Mediterraneens. 2008;81:23–27.
- Tamás L, Shewry PR. Heterologous expression and protein engineering of wheat gluten proteins. J. Cereal Sci. 2006;43(3):259–274.
- Takumi S, Nasuda S, Liu YG, et al. Wheat phylogeny determined by RFLP analysis of nuclear DNA.1. Einkorn wheat. Jpn J Genet. 1993;68(1):73–79.
- Li W, Fu BB, Li Z, et al. Characterization of the waxy gene in diploid Triticum L. and Aegilops L. species and its geographic distribution. Genet Resour Crop Evol. 2016;63(6):987–1002.
- Luo GB, Zhang XF, Zhang YL, et al. Composition, variation, expression and evolution of low-molecular-weight glutenin subunit genes in Triticum urartu. BMC Plant Biol. 2015; 15:68
- Yan ZH, Wan YF, Liu KF, et al. Identification of a novel HMW glutenin subunit and comparison of its amino acid sequence with those of homologous subunits. Chinese Sci Bull. 2002;47(3):220–225.
- Tamura K, Peterson D, Peterson N, et al. MEGA5: molecular evolutionary genetics analysis using maximum likelihood, evolutionary distance, and maximum parsimony methods. Mol Biol Evol. 2011;28(10):2731–2739.
- Wang YQ. MeteoInfo: GIS software for meteorological data visualization and analysis. Met Apps. 2014;21(2):360–368.
- Wang YQ, Li M, Guan YB, et al. Effects of an additional cysteine residue of avenin-like b protein by site-directed mutagenesis on dough properties in wheat (Triticum aestivum L.). J Agric Food Chem. 2019;67(31):8559–8572.
- Belton PS. Mini review: on the elasticity of wheat gluten. J Cereal Sci. 1999;29(2):103–107.
- Tatham AS, Shewry PR. Comparative structures and properties of elastic proteins. Philos Trans R Soc Lond B Biol Sci. 2002;357(1418):229–234.
- Ferrante P, Gianibelli MC, Larroque O, et al. Effect of incorporation of an i-type low-molecular-weight glutenin subunit and a modified γ-gliadin in durum and in bread wheat doughs as measured by micro-mixographic analyses. J Cereal Sci. 2006;44(2):194–202.
- Xu H, Wang RJ, Shen X, et al. Functional properties of a new low-molecular-weight glutenin subunit gene from a bread wheat cultivar. Theor Appl Genet. 2006;113(7):1295–1303.