Abstract
Coronavirus disease 2019 (COVID-19) is an acute respiratory infection caused by the Severe Acute Respiratory Syndrome Coronavirus 2 (SARS-CoV-2). However, there are still no specific treatments. Recently, traditional Chinese medicine (TCM) was proposed as a potential therapeutic strategy for the prevention and treatment of COVID-19. The present study aims to explore the potential of Hypericum japonicum Thunb. ex Murray against COVID-19 based on network pharmacology and molecular docking methods. Potential targets of H. japonicum were collected from available databases for protein–protein interaction analysis. Further GO and KEGG pathway analysis were performed to reveal the corresponding mechanism. Molecular docking was employed to evaluate the binding energy between the components in H. japonicum and three target proteins (ACE2, Mpro and PLP). Finally, a total of 45 core targets were screened. GO and KEGG pathway analysis revealed that several key pathways were involved in the potential beneficial effects of H. japonicum against COVID-19. Molecular docking results showed that most components in H. japonicum have a binding affinity to ACE2, Mpro and PLP molecules less than −5 kcal/mol, indicating good binding capacity to these target proteins. Vincetoxicoside B, bijaponicaxanthone and quercetin possess the top binding activity to ACE2, Mpro and PLP, respectively. The molecular docking results and the traditionally known possible functions of H. japonicum, including stabilizing blood oxygen environment, preventing inflammatory storms, regulating immune response and inhibiting viral infections, hereby indicate the potential of H. japonicum against COVID-19.
Introduction
Coronavirus disease 2019 (COVID-19) is an acute respiratory infection caused by the Severe Acute Respiratory Syndrome Coronavirus 2 (SARS-CoV-2). Epidemiological investigations revealed that SARS-CoV-2 spread very easily and sustainably between people, leading to a serious global public health crisis since December 2019 [Citation1]. According to real-time statistics from the Worldometer website, as of January 2021, there have been more than 102.5 million confirmed cases and 2.2 million deaths of COVID-19 worldwide (https://www.worldometers.info/coronavirus/). To date, there is still no specific treatment for COVID-19. The vaccine development has just completed clinical trials and been approved for emergency use in some countries and regions. However, even all manufacturers combined, COVID-19 vaccine production capacity is still limited and will not be able to meet global demand in the short term. The prevention and treatment strategies for COVID-19 are mainly to take antiviral and anti-inflammatory drugs, improve lung function, and alleviate acute lung injury. Generally speaking, it would take at least ten years to develop a new drug based on disease-related characteristics and market it, making it difficult to exert direct effects on the control of acute infectious diseases such as COVID-19. In addition, since SARS-CoV-2 is prone to mutations and antibodies persist in the body for a short time, it also adds uncertainty to the vaccines against the disease [Citation2]. Therefore, rapid screening of potential drugs from the existing marketed products is a feasible strategy for the prevention and treatment of COVID-19.
Traditional Chinese medicine (TCM) has played an important role in the treatment of COVID-19 and decreases mortality in China [Citation3,Citation4]. Containing multiple components, TCM preparations probably exert their therapeutic effects by inhibiting inflammation, immune regulation and anti-virus activity through multiple pathways and multiple targets, so as to achieve the purpose of relieving COVID-19 [Citation5–7]. However, the corresponding mechanism has not yet been clarified. Network pharmacology and molecular docking have been proved to be feasible strategies to explore these mechanisms. Network pharmacology is a discipline based on systems biology that conducts network analysis on biological systems and TCM systems, which can be used to clarify the inherent multi-component, multi-target and multi-pathway characteristics of TCM [Citation8]. Molecular docking is to use computer technology to simulate the interaction between drug molecules and disease targets through the geometric structure [Citation9]. It is widely used in drug virtual screening and prediction of drug targets.
Hypericum japonicum Thunb. ex Murray, called Tian-Ji-Huang or Di-Er-Cao in Chinese, is an annual herb mainly distributed in southern China [Citation10]. It was first documented as a herbal medicine in Sheng Cao Yao Xing Bei Yao published in Qing Dynasty, and has been used in alleviating internal heat, jaundice, diarrhea and swelling for a long time. Modern experimental studies revealed that H. japonicum possessed multiple pharmacological effects such as antibacterial, antiviral, anticancer, antioxidation and improving liver damage. Liu et al. [Citation11] evaluated the anti-influenza virus H3N2 effect of H. japonicum in mice at the concentration of 10 g/kg·d for intranasal administration, and found that H. japonicum could markedly inhibit the lung consolidation of mice pneumonia caused by the infection with influenza virus H3N2 and prolonged the survival time. Li et al. [Citation12] revealed that H. japonicum had a significant anti-duck hepatitis B virus effect, and could suppress liver cell degeneration, necrosis and inflammatory cell infiltration. In another study, H. japonicum showed an excellent antioxidant effect, which increased the activity of superoxide dismutase and glutathione peroxidase in the tissue, as well as reduced the content of malondialdehyde [Citation13]. These studies documented that H. japonicum possesses significant antiviral, anti-inflammatory and antioxidant effects, suggesting that H. japonicum could have potential to act against COVID-19. This study aims to explore the targets and mechanisms of H. japonicum against COVID-19 with network pharmacology and molecular docking technology, to provide a research basis for the development of H. japonicum as a complementary therapy for the prevention and treatment of COVID-19.
Materials and methods
Collection of compound-related targets
In our preliminary study, a total of 39 compounds (mainly flavonoids and organic acids) were identified in the extract of H. japonicum using ultra-high performance liquid chromatography-quadrupole-time of flight tandem mass spectrometry (UHPLC-Q-TOF-MS/MS) [Citation14]. Compound descriptions and corresponding PubChem CID are listed in . The related targets of these compounds were searched in Traditional Chinese Medicine Systems Pharmacology Database (TCMSP, http://tcmspw.com/), Comparative Toxicogenomics Database (CTD, https://ctdbase.org/) and Swiss Target Prediction Database (http://www.swisstargetprediction.ch/). In addition, the obtained target protein information was imported into the Uniprot database (https://www.uniprot.org/) to query corresponding gene names with the limitation of species to humans.
Table 1. Chemical constituents in Hypericum japonicum Thunb. ex Murray.
Identification of core targets and construction of herb-components-targets network
Targets information collected from TCMSP, CTD and Swiss Target Prediction database were imported into the String database (https://string-db.org/) for protein–protein interaction (PPI) analysis. The PPI analysis results were stored in TSV format and then imported into Cytoscape software (version 3.7.2) for network topology analysis. The nodes whose degree is greater than twice the median value, as well as the closeness centrality and betweenness centrality greater than their median value, were assigned as core targets [Citation15]. Subsequently, Cytoscape software was used to construct a visualized herb-components-targets network and analyse the topological characteristics of the network.
GO and KEGG pathway enrichment analysis
In order to further understand the function of screened core target and its role in the signalling pathways, the screened core target was imported into the DAVID database (https://david.ncifcrf.gov/) to carry out KEGG (Kyoto Encyclopedia of Genes and Genomes) pathway enrichment analysis and GO (Gene oncology) enrichment analysis.
Molecular docking study
The new coronavirus SARS-CoV-2 enters host cells through its S-protein binding to angiotensin converting enzyme (ACE2) in the human body, and then induces infection [Citation16]. The latest studies revealed that the binding affinity of SARS-CoV-2's S-protein to human ACE2 is much higher than that of SARS-CoV, leading to the stronger infectivity [Citation17]. As an RNA virus, SARS-CoV-2 needs to encode a polymer precursor protein during the replication process, and then hydrolyze to produce functional protein mediated by 3CL protease (Mpro) [Citation18]. In addition, papain-like protease (PLP) is also an important protease in the replication process of coronavirus [Citation19]. Therefore, the chemical components of H. japonicum were docked with the geometric structure of ACE2, Mpro and PLP to explore the potential activity in inhibiting SARS-CoV-2 virus infection and replication. We downloaded the protein structure of ACE2 (PDB ID: 1R4L), Mpro (PDB ID: 6LU7) and PLP (PDB ID: 4OVZ) from the RCSD protein database (https://www.rcsb.org/). We used AutoDock Tools software (version 1.5.6) to delete the water molecules of the target protein, separate the ligand and the receptor, add non-polar hydrogen, calculate the Gasteiger charge, and export to pdbqt format as docking receptors. We selected the original ligand binding location to serve as the docking active pocket. We downloaded the 3 D structure of the compounds in H. japonicum as sdf format from Pubchem database (https://pubchem.ncbi.nlm.nih.gov) or draw the structure through ChemBioDraw software (version 13.0). Open Babel software was used to convert the compound file to pdbqt format, and then import into AutoDock Tools software, add atomic charges, assign atomic types, and export to pdbqt format as a docking ligand. AutoDock Vina software (version 1.1.2) was run for docking, and the docking conformation with the highest score was kept, and finally Discovery studio software was used to visualize the docking results.
Results
Construction of herb-components-targets network
A total of 234 protein targets were collected from the TCMSP, CTD and Swiss Target Prediction databases. The obtained targets information was imported into the String database to perform PPI analysis. The PPI analysis results were imported into Cytoscape software for network topology analysis. As a result, a total of 45 core targets were screened, which related to 37 components from H. japonicum.
On the basis of the screened core targets, a herb-components-targets network was visualized using Cytoscape software. As shown in , the network has 83 nodes (1 herb, 37 components, 45 targets) and 988 edges. Among the 45 core targets, ten targets with top Degree values are PTGS2, TNF, CASP3, ATK1, ALB, INS, IL1B, VEGFA, IL6 and ESR1. Five components of top Degree values are quercetin, gallic acid, rutin, chlorogenic acid and kaempferol. It is easy to find that a single target in the network corresponds to multiple components, while a single component also acts on multiple targets, indicating that the chemical compounds contained in H. japonicum could act on multiple targets.
Pathway enrichment analysis
Through the KEGG pathway enrichment analysis, a total of 98 pathways (p < 0.05) were obtained based on the 45 screened core targets. The top 30 relevant pathways were presented in . These results show that the compounds in H. japonicum could act on multiple pathways, including HIF-1 signalling pathway related to hypoxic response, TNF signalling pathway and T cell receptor signalling pathway related to inflammation and immune regulation, Non-small cell lung cancer and Tuberculosis pathways related to respiratory disease, Hepatitis B and Hepatitis C signalling pathways related to viral diseases, PI3K-AKT signalling pathway related to the replication of viruses.
In addition to KEGG pathway enrichment, this work also performed GO enrichment analysis on the 45 screened core targets, which mainly involved biological processes, cell composition and molecular functions (). In the GO function enrichment analysis, a total of 471 GO items were obtained (p < 0.05). Among these items, the proportions corresponding to biological process (BP), cell composition (CC), molecular function (FF) were 80%, 8% and 12%, respectively ().
Molecular docking study
With the AutoDock Tools software, 39 components derived from H. japonicum were docked with the geometric structure of ACE2, Mpro and PLP. The obtained binding energy data are listed in . In molecular docking, the lower the energy when the ligand binds to the receptor, the higher the stability of the binding and the greater the possibility of interaction. As shown in , most compounds in H. japonicum have a binding affinity to ACE2, Mpro and PLP molecules less than −5kcal/mol, indicating that these compounds possess good binding capacity to these target proteins. The average binding energy of 39 compounds docking with ACE2, Mpro and PLP were −8.5 ± 2.8, −7.2 ± 1.3 and −8.2 ± 2.3 kcal/mol, respectively. Among these metabolites, vincetoxicoside B (−10.7 kcal/mol), bijaponicaxanthone (−10.5 kcal/mol) and quercetin (−10.7 kcal/mol) possess the top binding activity to ACE2, Mpro and PLP, respectively. The visualization results are shown in .
Figure 5. Docking results of Vincetoxicoside B with ACE2 (A), Bijaponicaxanthone with Mpro (B) and Quercitrin with PLP (C).
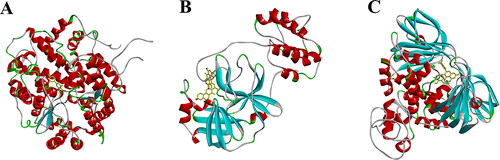
Table 2. Binding energy obtained by docking analysis.
Discussion
In this study, target proteins of 39 components in H. japonicum were collected from TCMSP, CTD and Swiss Target Prediction database. With protein–protein interaction and network topology analysis, core targets were identified and the herb-components-targets network was constructed. Further KEGG and GO pathway enrichment analysis, as well as the molecular docking study, jointly revealed the potential protective effects of H. japonicum against COVID-19.
As shown in , a total of 45 core targets were screened by network pharmacology analysis, such as cyclooxygenase 2 (PTGS2) involved in the inflammatory response of multiple tissues and organs, mitogen-activated protein kinase 3 (MAPK3) related to inflammation, interleukin 6 (IL-6) involved in immune response and anti-infection regulation, tumour necrosis factor (TNF) and interleukin 1β that regulate human immune function.
KEGG pathway enrichment analysis indicated that the chemical compounds of H. japonicum could act on multiple pathways related to the treatment of COVID-19, such as the HIF-1 pathway related to cell hypoxia, the TNF and T cell receptor signalling pathway related to the regulation of immune inflammation. Severe COVID-19 patients often experience dyspnoea and/or hypoxemia one week after the infection. HIF-1α produced by the HIF-1 pathway can mediate hypoxia signals and regulate a series of compensatory responses to hypoxia [Citation20]. In this study, HIF-1 signalling pathway was assigned as the major pathway of H. japonicum acting on SARS-CoV-2, suggesting that H. japonicum could stabilize the blood oxygen saturation in patients with COVID-19. COVID-19 patients have varying degrees of cytokine storms, leading to immune damage to lungs and other organs. There are high concentrations of inflammatory factors such as IL-6, MCP-1, MIP-1A and TNF-α in the plasma of severe COVID-19 patients [Citation21,Citation22]. Compounds in H. japonicum could act on multiple targets and pathways closely related to inflammation regulation, such as PTGS2, TNF-α, IL-6, as well as TNF and T cell receptor signalling pathway, suggesting that its inhibitory effects act on the inflammatory storm caused by COVID-19. In addition, the obtained results showed that H. japonicum could regulate the PI3K-AKT signalling pathway, leading to the inhibition of virus replication [Citation23].
SARS-CoV-2 must combine with the ACE2 protein through S protein to successfully enter the host cell, while Mpro and PLP are the crucial proteins for the SARS-CoV-2 virus to replicate itself in the host cell [Citation16,Citation18,Citation19]. Hence, ACE2, Mpro and PLP are considered as potential targets for screening of anti-SARS-CoV-2 drugs. In this study, several compounds in H. japonicum were identified to have good binding activity with the three mentioned target proteins, indicating the potential of H. japonicum in inhibiting SARS-CoV-2 virus infection and replication. Indeed, some compounds identified in H. japonicum are not unique. However, the content of these compounds varies in different herbs. Vincetoxicoside B (quercetin 7-rhamnoside) was determined as the main component in in H. japonicum [Citation24], and was predicted to possess the top binding activity to ACE2 in the present study. Vincetoxicoside B has been proved to effectively inhibit the replication of porcine epidemic diarrhea virus in the early stage [Citation25], and its antiviral effect did not depend on its antioxidant capacity [Citation26]. Additionally, H. japonicum contains a distinct class of xanthone compounds, such as bijaponicaxanthone, 1,3,5-trihydroxyxanthone, and euxanthone. To our knowledge, bijaponicaxanthone has only been reported in H. japonicum, and showed a strong binding activity to Mpro in the present docking analysis. Euxanthone reportedly showed anti-HIV-1 activities through the inhibition of reverse transcriptase in the syncytium assay using Δ Tat/RevMC99 virus and the 1A2 cell line system [Citation27]. There have been relatively few other studies investigating the bioactivities of these xanthone compounds. Our results suggested that the xanthone compounds in H. japonicum deserve further study, especially for their potential anti-SARS-CoV-2 activity. However, some flavonoid components in H. japonicum, such as quercetin, are also widely found in other herb medicines, which may show potential beneficial effects in the treatment of COVID-19 due to their anti-inflammatory, antioxidant and immunoregulatory abilities [Citation28,Citation29].
In this work, the results were obtained based on network pharmacology and silico molecular docking, which preliminarily reveal the potential of H. japonicum against COVID-19. More high-quality mechanistic investigations and clinical trails are needed to confirm its efficacies in the treatment of COVID-19 in the future.
Conclusions
This study explored the prospects of H. japonicum in relieving COVID-19 through network pharmacology and molecular docking technology. The obtained results revealed that H. japonicum may exert multiple functions in stabilizing blood oxygen environment, preventing inflammatory storms, regulating immune response and inhibiting viral infections, hereby indicating the potential of H. japonicum against COVID-19.
Disclosure statement
No potential conflict of interest was reported by the author(s).
Data availability statement
The data supporting the findings reported in this study are available from the corresponding author upon reasonable request.
Additional information
Funding
References
- Harapan H, Itoh N, Yufika A, et al. Coronavirus disease 2019 (COVID-19): a literature review. J Infect Public Health. 2020; 13(5):667–673.
- Zou Q, Li H, Zeng H. Current status and countermeasures for development of drugs to treat coronavirus disease 2019. J Third Military Med Univ. 2020; 42(9):861–866.
- Liu M, Gao Y, Yuan Y, et al. Efficacy and safety of integrated traditional chinese and western medicine for Corona Virus Disease 2019 (COVID-19): a systematic review and meta-analysis. Pharmacol Res. 2020; 158 :104896.
- Pang B, Zhang J, Lee MS, et al. Enlightenment from clinical trials on Chinese medicine for Coronavirus Disease 2019 (COVID-19). Integr Med Res. 2020; 9(3):100481
- Xiao M, Tian J, Zhou Y, et al. Efficacy of Huoxiang Zhengqi dropping pills and Lianhua Qingwen granules in treatment of COVID-19: a randomized controlled trial. Pharmacol Res . 2020; 161 :105126.
- Zhao Z, Li Y, Zhou L, et al. Prevention and treatment of COVID-19 using traditional Chinese medicine: a review. Phytomedicine. 2020; 153308.
- Lin A, Song E, Lee HW, et al. Herbal medicine for the treatment of Coronavirus Disease 2019 (COVID-19): a systematic review and meta-analysis of randomized controlled trials. J Clin Med. 2020;9(5):1583.
- Li P, Su W. Recent progress in applying network pharmacology to research of Chinese materia medica. Chinese Tradit Herbal Drugs. 2016;47(16):2938–2942.
- Meng XY, Zhang HX, Mezei M, et al. Molecular docking: a powerful approach for structure-based drug discovery. Curr Comput Aided Drug Des. 2011;7(2):146–157.
- Liu LS, Liu MH, He JY. Hypericum japonicum Thunb. ex Murray: phytochemistry, pharmacology, quality control and pharmacokinetics of an important herbal medicine. Molecules. 2014; 19(8):10733–10754.
- Liu N, Hu X, Meng Y, et al. Effect of Anti-influenza Virus H3N2 of Hypericum japonicum in vivo. J Chinese Medic Mater. 2008;31(7):1022–1024.
- Li P, Yang C, Wang Y, et al. Experimental study on anti-duck hepatitis B virus effect of Hypericum japonicum extract. J Chinese Medic Mater. 2011; 34(6):956–958.
- Li P, Yang C, Wang Y, et al. Effect of anti-hepatic fibrosis of Hypericum japonicum in rats. J Chinese Medic Mater. 2011; 34(3):424–428.
- Su W, Wang Y, Yao H, et al. Multidisciplinary combined technology based research on Hypericum japonicum. Guangzhou: Sun Yat-sen University Press, 2020.
- Chen P, Yao H, Yuan Q, et al. Discovery of the possible mechanisms in kouyanqing granule for treatment of oral ulcers based on network pharmacology. BMC Complement Med Ther. 2020;20(1):258
- Xu X, Chen P, Wang J, et al. Evolution of the novel coronavirus from the ongoing Wuhan outbreak and modeling of its spike protein for risk of human transmission. Sci China Life Sci. 2020;63(3):457–460.
- Wrapp D, Wang N, Corbett KS, et al. Cryo-EM structure of the 2019-nCoV spike in the prefusion conformation. Science. 2020;367(6483):1260–1263.
- Zhang Q, Zheng W, Xiao J, et al. Broad spectrum inhibitors of 3C and 3CL proteases: research advances. J Int Pharmaceut Res. 2016;43(3):425–430.
- Yang Y, Sun L, Chen Z. Structure and functions of papain-like protease of severe acute respiratory syndrome (SARS) coronavirus. Chinese J Biochem Molec Biol. 2010; 26(1):15–21.
- Yang M, Zhou N, Wang Z, et al. Research progress on the role of transcription factor HIF-1α and its signal pathway in the pathogenesis. Biotechnol Bullet. 2016; 32(8):8–13.
- Huang C, Wang Y, Li X, et al. Clinical features of patients infected with 2019 novel coronavirus in Wuhan, China. Lancet. 2020; 395(10223):497–506.
- Liu J, Li S, Liu J, et al. Longitudinal characteristics of lymphocyte responses and cytokine profiles in the peripheral blood of SARS-CoV-2 infected patients. EBioMedicine. 2020;55:102763.
- Zhu L, Ding X, Zhu X, et al. Biphasic activation of PI3K/Akt and MAPK/Erk1/2 signaling pathways in bovine herpesvirus type 1 infection of MDBK cells. Vet Res. 2011;42(1):57
- Huang ZQ, Pan C, Su WW, et al. Antioxidant activity and hepatoprotective potential of Quercetin 7-Rhamnoside in vitro and in vivo. Molecules. 2018; 23(5):1188.
- Choi HJ, Kim JH, Lee CH, et al. Antiviral activity of quercetin 7-rhamnoside against porcine epidemic diarrhea virus . Antiviral Res. 2009; 81(1):77–81.
- Song JH, Shim JK, Choi HJ. Quercetin 7-rhamnoside reduces porcine epidemic diarrhea virus replication via independent pathway of viral induced reactive oxygen species. Virol J. 2011; 8 :460
- Reutrakul V, Chanakul W, Pohmakotr M, et al. Anti-HIV-1 constituents from leaves and twigs of Cratoxylum arborescens. Planta Med. 2006; 72(15):1433–1435.
- Chiow KH, Phoon MC, Putti T, et al. Evaluation of antiviral activities of Houttuynia cordata Thunb. extract, quercetin, quercetrin and cinanserin on murine coronavirus and dengue virus infection. Asian Pac J Trop Med. 2016; 9(1):1–7.
- Fan D, Zhou X, Zhao C, et al. Anti-inflammatory, antiviral and quantitative study of quercetin-3-O-β-D-glucuronide in Polygonum perfoliatum L. Fitoterapia. 2011; 82(6):805–810.