Abstract
Biological and molecular properties of six Prunus necrotic ringspot virus (PNRSV) isolates originating from sweet and sour cherry trees were studied. Double-antibody sandwich enzyme-linked immunosorbent assay (DAS-ELISA) showed PNRSV infection in leaf samples from the respective source trees. While one of the infected source trees was symptomless, the other five showed symptoms, ranging from chlorotic to necrotic spots on the leaves. The reaction of greenhouse-grown seedlings of 22 herbaceous test plant species was investigated. The woody indicators Prunus serrulata cv. Kwanzan, wild cherry Prunus avium, P. cerasus, P. cerasifera and P. tomentosa were chip-budded. Chenopodium quinoa, Cucumis sativus cv. Amelia, C. sativus cv. Levina and P. tomentosa appeared as the most suitable test plants for the bioassay of the studied PNRSV isolates. The nucleotide sequences corresponding to the full-length coat (CP) and movement (MP) proteins were obtained. The nucleotide sequence identity among the studied isolates both in CP and MP genes was from 94% to 100%. The CP and MP amino acid sequence identities were from 95% to 100% and from 94% to 100%, respectively. Phylogenetic analyses performed with MP and CP nucleotide sequences showed that three isolates belonged to PV32-I and the other three to PV96-II phylogroups. Comparative amino acid analyses of MP and CP genes, together with the symptoms on naturally infected trees showed a higher affiliation of the studied isolates with the mild type than with the rugose types of isolates. The molecular variability correlated with the symptomatology on the naturally infected trees.
Introduction
Prunus necrotic ringspot virus (PNRSV) is distributed worldwide, infecting most cultivated Prunus species including sweet and sour cherry. The virus is a member of the genus Ilarvirus in the family Bromoviridae, and has a tripartite genome, with RNA1 and RNA2 encoding proteins involved in viral replication and RNA 3 encoding the 3a (putative movement protein, MP) and the 3b (coat protein, CP) [Citation1].
PNRSV is easily transmitted by the vegetative propagation material and by pollen grains and seeds [Citation2,Citation3]. The symptoms caused by PNRSV may depend on the virus isolate, host species and/or the cultivar and climatic conditions. PNRSV infection in cherry can be latent or symptomatic consisting of smaller leaves, diffused chlorotic rings and/or spots, necrotic lesions or spots, and the ‘tattered’ leaves [Citation4,Citation5]. The existence of different PNRSV isolates in sweet cherry trees, causing mild mottle and rugose mosaic symptoms was described [Citation3,Citation6].
Many PNRSV isolates have been biologically [Citation6–10], serologically [Citation11–13] and molecularly characterized. Based on the CP gene of RNA3 PNRSV isolates from different Prunus species and geographical regions have been phylogenetically grouped into the four subgroups PV32-I, PV96-II, PE5-III and CH30 [Citation8,Citation14–18]. The same phylogroups PV32-I, PV96-II and PE5-III, referred to as PG1, PG2 and PGIII have been reported based on the putative MP gene [Citation19].
Sweet cherry is an important fruit crop in Bulgaria. A study of Ilarvirus occurrence in sweet and sour cherry in Bulgaria has shown 19.2% rate of infection with PNRSV and Prune dwarf virus (PDV) and the absence of Apple mosaic virus (ApMV) [Citation20]. Investigation of the reaction of woody and herbaceous test plants to five Bulgarian isolates of PNRSV from different Prunus species has revealed the presence of virulent, moderate and mild isolates [Citation21]. Recently, 25 isolates from sweet and sour cherry were sequenced in the CP gene and phylogenetically defined to belong to PV32-I and PV96-II phylogroups [Citation22].
The objective of the present study was to compare biological properties, as host range and symptomatology of six PNRSV isolates from sweet and sour cherry trees with their molecular variability based on the sequences of CP and MP genes.
Materials and methods
Virus material and serological detection
The following PNRSV isolates were studied: -PN1, -PN7, -PV8, -PN10, -PN11 and -PN15. Their source hosts and the symptoms observed on naturally infected trees are shown in .
Table 1. List of biologically and molecularly studied PNRSV isolates.
Leaf samples from source trees were collected in early spring and tested for PNRSV, PDV, ApMV, Apple chlorotic leafspot virus (ACLSV), Plum pox virus (PPV) and Raspberry ringspot virus (RpRSV) by double-antibody sandwich enzyme-linked immunosorbent assay (DAS-ELISA) assay [Citation23] following the manufacturer’s protocol (Loewe Phytodiagnostica GmbH). The photometric measurement was done at 405 nm after 2 h. Samples were considered as positive if their absorbance values were more than three times higher than the negative control.
Host range study
Infected young leaves from the source trees were ground in mortar with 0.05 mol/L sodium-phosphate buffer, pH 7.4 and used for inoculation of Chenopodium quinoa and cucumber plants (Cucumis sativus cvs. Levina/Tessa). Then the cultures were passed through Cucumis sativus cvs. Levina/Tessa from which systemically developed leaves were used for further mechanical inoculations of greenhouse-grown seedlings of the following species of test plants: Cucumis sativus L. cv. Levina, C. sativus L. cv. Amelia F1, C. sativus L. cv. Tessa F1, C. sativus L. cv. Aikon, C. sativus L. cv. Gergana, C. sativus L. cv. Titus F1, C. sativus L. cv. Aliaga F1, Cucurbita maxima cv. Byala Plovdivska 48/4, Cucurbita maxima cv. Turkish Turban, Cucurbita moschata cv. Smooth Butternut, C. moschata cv. Viola, Cucurbita pepo cv. Izobilna F1, C. pepo Falcon, C. pepo cv. Black Beauty, Chenopodium quinoa Wild., Chenopodium foetidum Lam., Vigna unguiculata L., Zinnia elegans, Lagenaria siceraria, Helianthus annuus L., Petunia hybrida and Vinca rosea L. Different plant numbers but not less than four of the respective species/cultivar were used per each PNRSV isolate. Inoculated plants were grown in a green house at 22–26 °C. Local and systemic disease symptoms were recorded from the 5th to the 21–24th days after inoculation.
The woody indicators Prunus serrulata cv. Kwanzan, wild cherry Prunus avium, P. cerasus, P. cerasifera and P. tomentosa were chip-budded. For each isolate, three plants per species were used. The symptoms on the trees were evaluated during two seasons. Inoculated herbaceous and woody plants were tested by DAS-ELISA.
cDNA synthesis and PCR amplification
Total nucleic acid was extracted from 100 mg fresh and fully developed leaves of the respective source tree using RNeasy Plant Mini extraction kit (Qiagen, Germany). All RNA samples were stored at −80 °C.
Synthesis of cDNA was carried out in a 20 µL reaction mixture, containing 2 µL (about 1 µg) of template RNA, 1 µL (10 mmol/L) of the specific antisense primer, 2.5 µL (2.5 mmol/L) deoxynucleoside triphosphates (dNTPs, containing 2.5 mmol/L of each dATP, dGTP, dCTP and dTTP) and sterile distilled water to a final volume of 12.5 µL. The mixture was heated to 65 °C for 5 min and quickly chilled on ice. Then 4 µL of 5× first strand buffer, 2 µL of 0.1 mol/L DDT (both, supplied with the kit), 0.5 µL of RNaseOUT (40 units/mL) and 1 µL smart Reverse Transcriptase (200 U/µL) were added, mixed gently and incubated at 50 °C for 30 min. The reaction was terminated by heating at 85 °C for 5 min.
The CP and MP genes were amplified in a Quanta Biotech thermal cycler. The used RT-PCR sense and antisense primers for CP gene, respectively, were as follows: F3: 5′-ATGGTTTGCCGAATTTGCAATCAT-3′ and R4: 5′-AGTGTGCTTATCTCACTCTAG-3′ [Citation10]. The expected products were 700 bp amplicon, which is a fragment of the RNA3 CP coding sequence. The 900 bp fragment of the MP gene was amplified using the sense primer: 5′-GTTGGTTGAATAGTGTTTCAGTATGGCC-3′ and the antisense primer: 5′-AGCGTGGGTATGATTGCAAATTCGG-3′ [Citation14].
PCR amplifications were performed, in which 1 µL of cDNA was mixed with 24 µL of the amplification mixture containing 2.5 µL of 10× reaction buffer (200 mmol/L Tris-HCL pH 8.4, 500 mmol/L KCL), 1.5 µL of MgCl2 (25 mmol/L), 2.5 µL dNTPs (2.5 mmol/L), 0.5 µL of each primer (10 mmol/L), 0.25 µL Taq DNA polymerase (5 U/µL; EURx, Ltd.) and 16.25 µL RNase-free sterile water.
Thermocycling for the CP gene was carried out as follows: initial denaturation at 94°С for 2 min, then 35 cycles of 94 °C for 10 s, 53 °C for 30 s and 72 °C for 40 s. The final polymerization step was for 5 min at 72 °C. PCR for the MP gene was performed for 2 min at 94 °C, followed by 40 cycles at 94 °C for 10 s, 62 °C for 30 s and 72 °C for 90 s and final extension for 10 min at 72 °C.
The amplified products (5 µL each) were electrophoresed at 80–140 V for 1–1.5 h in 1.2% agarose gels in 40 mmol/L Tris-acetate and 1 mmol/L EDTA, pH 8.0 (TAE) and stained with GelRed dye (stock solution 10,000×) (Biotium).
Nucleotide sequencing and phylogenetic analyses
The obtained PCR products were purified using IllustraTM GFXTM PCR DNA purification system (Healthcare, UK) and custom sequenced in both directions with the same oligonucleotides used for PCR (Eurofins, Germany). The nucleotide and deduced amino sequences were deposited in the GenBank database (https://www.ncbi.nlm.nih.gov/genbank) (accession no. in ). Multiple nucleotide alignments were conducted using ClustalW 1.8 [Citation24]. Phylogenetic trees were generated from nucleotide alignments of complete CP and MP sequences of PNRSV isolates by the neighbor-joining (NJ) method with Kimura two-parameter model using Molecular Evolutionary Genetics Analysis (MEGA) software program version 6 [Citation25]. The statistical significance of the clusters and branches of the phylogenetic tree was tested by ‘bootstrap’ analyses with 1000 replications. Bootstrap values below 70% were collapsed. Corresponding sequences of representative PNRSV isolates available from the GenBank database were used as references and their accession numbers are as follows: PV0096 (S78312.1), PE5 (L38823) [Citation26], PV32 (Y07568) [Citation27], CH30 (AF034994), CH9 (AF034992), CH38 (AF034991), CH39 (AF034990), CH57 (AF034993), CH61 (F034989), CH71 (AF034995) [Citation7] and ChrIt.bla 1 [AJ133210 (CP); AJ306821 (MP)] [Citation15].
Results and discussion
Virus isolates and symptomatology
Two of the studied isolates (PN1 and PN10) originated from sour cherry, and the other four isolates PN7, PV8, PN11 and PN15 from sweet cherry trees (). In DAS-ELISA tests, all samples from the source trees reacted positive for PNRSV and negative for PDV, ApMV, ACLSV, PPV and RpRSV (data not shown).
The trees from which the respective isolate originated showed chlorotic (PN1and PN11) or necrotic spots (PN7 and PN10) on the leaves. No symptoms on the leaves of the source tree of PV8 isolate were observed. In general, the leaf symptoms were more pronounced in early spring, soon after foliation. In addition to the leaf symptoms, deformation and narrow leaves were observed on the source trees of PN11 and PN15 isolates. The typical symptoms induced by PNRSV include chlorosis, necrosis, leaf deformation and growth defects [Citation28]. Depending on the isolate, the reaction of PNRSV-infected cherry trees is from symptomless to a rugose mosaic disease [Citation3].
Host range studies
The results of herbaceous host range trials are shown in . Chenopodium foetidum Lam., Helianthus annuus L., Lagenaria siceraria, Petunia hybrida, Vigna unguiculata L., Zinnia elegans and Vinca rosea L. did not develop any symptoms and proved not to be infected upon DAS-ELISA tests (data not shown). Unlike our results symptoms on the leaves of Vigna unguiculata L., Zinnia elegans and Helianthus annuus L., induced by PNRSV isolate from peach have been reported [Citation9].
Table 2. Symptoms on herbaceous test plants induced by six PNRSV isolates from sweet and sour cherry trees. Virus infection was confirmed by ELISA assay.
Five isolates were able to infect Chenopodium quinoa (except PN11), as PN1 induced chlorotic spots on inoculated leaves and PN7, PV8, PN10 and PN15 induced also systemic chloric mottling on the tip leaves (). Such reaction of Ch. quinoa to PNRSV isolate from peach has been described [Citation29]. All isolates produced local chlorotic spots on the cotyledons of Cucumis sativus cvs. Levina and Tessa 5–6 days after inoculation, and later mosaic on the growing leaves (excluding isolate PN1 on cv. Levina and PN11 on cv. Tessa). C. sativus cv. Amelia reacted to four isolates with abundant local and systemic chlorotic spots (), as the plants inoculated with PN10 reacted also with systemic necrosis (). Along with the described symptoms, C. sativum cv. Levina and C. sativum cv. Amelia reacted also with stunted growth to PN10 and PN15 in comparison with the uninfected controls. The tested cultivars of Cucurbitaceae varied in their reactions to the studied PNRSV isolates. The most sensitive was C. pepo Black Beauty, which reacted to four isolates with local chlorotic spots and systemic peripheral necrosis on the leaves (). Cucurbita moschata cv. Smooth Butternut and C. pepo cv. Falcons were less sensitive, showing local or systemic symptoms to only PV8 and PN7, respectively. Both in quantitative and qualitative aspects the herbaceous host range study outlined PNRSV-PN7, -PV8, -PN10 and -PN15 as more infectious in comparison with PNRSV-PN1 and -PN11 isolates. The first four isolates induced symptoms on 10 (9 for PN15) out of 22 inoculated plant species, as the local and/or systemic symptoms on C. sativus cultivars, varied from mild (cv. Aikon), to severe and very severe (cvs. Amelia and Levina). On the basis of clearly expressed symptoms and the higher number of infected plants, we could recommend C. quinoa, C. sativus cv. Amelia and C. sativus cv. Levina as the most suitable test plants for bioassays of the studied PNRSV isolates. In a biological characterization of PNRSV isolates from rose C. sativus cv. Wisconsin, Cucurbita maxima cv. Butercup, C. pepo Melonowa Zolta, Chenopodium amaranticolor and C. quinoa were reported as useful for differentiation of isolate-specific pathogenicity [Citation10].
Figure 1. Symptoms on test plant species induced by PNRSV isolates; (A) local and systemic infection on Chenopodium quinoa (isolate PN15); (B) local and systemic symptoms on the leaves of Cucumis sativus cv. Amelia (isolate PV8); (C) systemic necrosis on the leaves of Cucumis sativus cv. Amelia (isolate PN10); (D) and (E) local and systemic peripheral necrosis, respectively, on the leaves of Cucurbita pepo cv. Black Beauty (isolate PN7) (original A. B.).
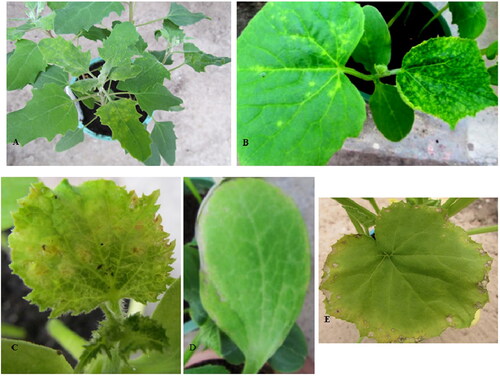
The first symptoms of viral infection on the leaves of the woody plants appeared approximately 30–50 days after their graft inoculation with chip buds. All isolates were able to infect P. tomentosa, as the plants showed the clearest and more distinctive symptoms expressed as interveinal chlorosis, marginal necrosis, chlorotic and necrotic spots (). In comparison with the healthy controls, all studied isolates caused apparent stunted growth (). The strongest manifestation of the described symptoms was noted on the plants inoculated with isolate PN15 (). Following the described shock reaction the leaves that developed later in the season remained symptomless. The symptoms caused by the studied isolates on P. tomentosa were similar to those described by Damsteegt et al. [Citation30], who reported it as a useful species for PNRSV biological indexing. Prunus avium, P. cerasus, P cerasifera and P. serrulata cv. Kwanzan were inoculated only with PN1, PN7 and PV8 isolates. The following types of symptoms on the plants of P. avium were observed: chlorotic ring spots (isolate PN1), necrotic spots with shot holes (isolate PN7) and ‘tattered’ leaf (isolate PV8). P. cerasus and P. cerasifera reacted only to PN7 isolate with chlorotic and necrotic ring spots on single leaves and with small light yellow ring spots, respectively. The plants from P. serrulata cv. Kwanzan reacted to grafted buds of PN1 and PN7 isolates with local necrosis around grafted buds and occasional gum flow. P. avium clone F12/1 was reported as one of the best woody indicator for biological indexing of PNRSV variants [Citation31]. Based on symptom expression on P. avium clone F12/1, P. mahaleb and P. cerasifera PNRSV isolates from different Prunus species were divided into the acute, moderate and mild types [Citation32]. Presently we are not able to outline some differences among studied isolates based on the reaction of the used woody indicator species due to the lack of data on the reaction of Prunus avium, P. cerasus, and P. serrulata cv. Kwanzan to PN10, PN11 and PN15 isolates.
Comparison of PNRSV sequences encoding CP and MP
The CP and MP genes of studied isolates were sequenced and deposited into GenBank (). The obtained nucleotide sequences were compiled and trimmed to correspond to the complete CP gene. The CP sequences of PN1, PN10 and PN11 isolates were 681 nucleotides (nt) in length, translated to 227 amino acids (aa), and that of PN7, PV8 and PN15 isolates were 675 bp-long translated to 225 amino acids. Six more nucleotides (CTAGGA) in PN1 and PN10 and (ATAGGA) in PN11 isolates were absent in the sequences of PN7, PV8 and PN15 isolates and corresponded to the two extra amino acids NR (Asn-Arg). The presence of GTAGGA extra nucleotides translating SR (Ser-Arg) amino acid residues was reported as group-specific for isolates belonging to the PV32-I phylogroup [Citation8,Citation15]. The identity among the studied isolates in the CP gene was from 94% to 100% and from 95% to 100%, at the nucleotide and amino acid levels, respectively.
The obtained nucleotide sequences of the MP gene were 852 nucleotides in length translated to 284 amino acids and shared both 94–100% nucleotide and amino acid identities.
Phylogenetic analyses of CP and MP sequences
In order to determine the phylogenetic position of the studied isolates, their nucleotide sequences were compared with the sequences of other isolates, representatives of the reported PV32-I, PV96-II, PE5-III and CH30 phylogenetic groups [Citation15–18]. The phylogenetic tree generated from the nucleotide sequences of the CP gene revealed four main groups (PV32-I, PV96-II, PE5-III and CH30), which were highly supported by the bootstrap values (). In the CP-based tree, three isolates clustered into PV32-I and the other three into PV96-II phylogroups, thus supporting our finding that the PNRSV isolates from sweet and sour cherry belonged to these two groups [Citation22].
Figure 3. Phylogenetic analyses of studied Prunus necrotic ringspot virus (PNRSV) isolates and isolates available in GenBank database based on the nucleotide sequences of their coat protein (A) and movement protein (B) genes. The trees were constructed with MEGA6 using the neighbor-joining method. The numbers at the nodes indicate the percentage of 1000 replicates, as the values below 70% are not shown. All Bulgarian isolates are marked in bold. The underlined isolates are the representatives of the respective phylogroup. The bar represents 0.01 substitutions per site.
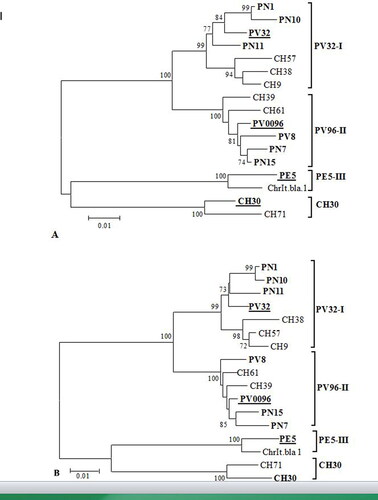
Similar phylogenetic relationships (the same topology) were observed in the MP-based tree (). As in the CP tree, the PN1, PN10 and PN11 isolates from the PV32-I phylogroup clustered together with the apple isolate PV32 forming a cluster separated from the cluster of the CH57, CH9 and CH38 isolates from sweet cherry in the USA reported as rugose pathotypes of the virus [Citation33]. The nucleotide and amino acid identities among the studied and rugose-type isolates within CP were 96–97% and 93–95%, respectively, and those within the MP gene were 96–97% and 96–98%, respectively.
The other studied isolates PN7, PV8 and PN15 belonging to the PV96-II group were closely related to the PV0096 isolate from P. mahaleb and the two sweet cherry isolates CH39 and CH61 from the USA reported as mild pathotypes of PNRSV [Citation11,Citation33]. The overall nucleotide and amino acid identities between the studied and mild-type isolates from PV96-II phylogroup in CP gene were 97–98% and 96%, respectively, and that in the MP gene 98% and 97–98%, respectively.
None of the studied isolates belonged to PE5-III group reported to consist of several severe cherry isolates from the USA and two peach isolates, of which one mild and one latent [Citation8]. CH30 group proposed by Glasa et al. [Citation18] was composed of only two referent cherry isolates, CH30 and CH71, described as rugose and mild pathotype isolates, respectively [Citation7,Citation11].
The comparison of CP amino acid sequences revealed that the studied isolates in each of the two phylogroups (PV32-I and PV96-II) had phylogroup-conserved amino acid residues. The amino acid substitutions I5/F5, G24/D24, A46/46, T50-L50/V50/I50 (in PN1, PN10 and PN11, respectively), and E139/D139 () were observed between the isolates analyzed in the present study and PV96-II- and PV32-I-type isolates, respectively. In addition, the following four substitutions, A53/M53/T53, V60/A60, P72/H72 and N83/S83 were noted between PN1, PN10 and PN11 isolates and severe rugose mosaic type isolates CH9, CH38 and CH57 from PV32-I phylogroup. Presently, the role of these amino acid substitutions in the biological properties of the above-mentioned isolates is not clear. The amino acid substitutions T50/I and F79/Y in the CP gene were reported to differentiate the mild- from the rugose-type isolates, respectively, of the CH9 serotype [Citation7]. Comparison of amino acid sequences of PN1, PN10 PN11 isolates with those of CH61 (mild) and CH9 (rugose) showed that at the mentioned positions only isolate PN11 had the amino acid residue I50 present in the rugose-type CH9, while isolates PN1 and PN10 had the amino acid residues L50 and V50, respectively. In respect to the F79/Y amino acid substitution, all of the studied isolates, both from PV96-II and PV32-I phylogroups contained F (phenylalanine, phe) amino acid residue. Having in mind the relatively mild symptoms observed on the leaves of infected source trees of the PN1, PN10 and PN11 isolates, as well their grouping in a cluster separated from CH9 rugose-type isolates we suggest the affiliation of these isolates to the mild-type isolates of PNRSV. According to Hammond and Crosslin [Citation7], the CH9 serotype isolates represent two major groups of biological variants, severe rugose and mild viruses. The PNRSV isolates from CH9 serotype may cause a range of symptoms [Citation11].
Figure 4. Multiple alignments of amino acid sequences of the coat protein of studied PNRSV isolates and cherry isolates representatives of the mild and rugose pathotypes from PV96-II and PV32-I phylogroups. Dots indicated identical amino acid residues among all isolates.
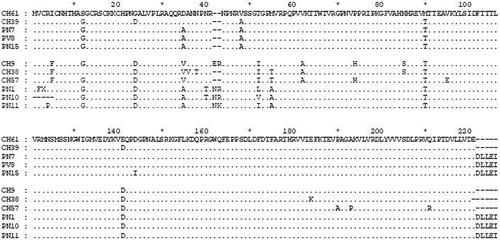
The alignment of the predicted MP amino acid sequences of the studied isolates (data not shown) belonging to PV32-I and PV96-II phylogroups revealed the following common amino acid substitutions K80/N80, D256/E256, V257/D257 and L260/T260, respectively. The amino acid residues C24/S24, F50/L50, A126/E126, T228/N228, I253/V253, D256/E256, V257/D257 and L260/T260 were reported as conservative and differentiating rugose from the mild type of the CH9 serotype isolates [Citation7]. From the amino acid substitutions characteristic of rugose-type isolates only D256, V257 and L260 were present in PN1, PN10 and PN11 from PV32-I phylogroup, while at all other above-mentioned positions, the isolates analyzed in this study contained the residues of mild-type isolates.
In general, the comparison of amino acid sequences of both CP and MP genes together with the symptoms observed on naturally infected trees showed a higher connectivity of studied isolates with the mild than with the rugose types of isolates. Our results confirmed the reported correlation between symptom severity on naturally infected trees and the primary sequence of the gene for CP and MP for PNRSV isolates from cherry [Citation7]. Simultaneously, however, four of the PNRSV isolates studied herein (PN7, PV8, PN10 and PN15) compared to the other two isolates (PN1 and PN11) showed some differences in their pathogenicity in respect to the used herbaceous test plants. As pointed out [Citation34], a large variety of viral proteins can contribute to viral pathogenesis. The molecular mechanism by which these proteins contribute to symptom definition is largely unknown.
Conclusions
This is the first comparative study of biological and molecular properties of PNRSV isolates from sweet and sour cherry trees in Bulgaria. On the basis of sequence homology in the CP and MP, the studied PNRSV isolates were classified into PV32-I and PV96-II phylogroups. While no large difference in the symptoms on naturally infected trees was observed, the host range study revealed some differences among the studied isolates both in a quantitative and qualitative aspect. A correlation was found between the mild symptoms on naturally infected trees and the sequences of MP and CP genes of their representative isolates, which is valid for PNRSV isolates from cherry and not valid for isolates from different Prunus species. No association could be outlined between the biological properties of the analyzed isolates in respect to the severity of the expressed symptoms on herbaceous and woody test plants and their source trees.
Ethical approval
This article does not contain any studies with human participants or animals performed by any of the authors.
Disclosure statement
The authors report no conflict of interest.
Funding
Data availability
All data that support the findings reported in this study are available from the corresponding author upon reasonable request.
References
- Murphy FA, Fauquet CM, Bishop DHL, et al. Virus taxonomy: classification and nomenclature of viruses. Arch Virol Suppl. 1995;10:450–457.
- George J, Davidson TR. Pollen transmission of necrotic ringspot virus and sour cherry yellows viruses from tree to tree. Can J Plant Sci. 1963;43(3):276–378.
- Howell WE, Mink GI. Natural spread of cherry rugose mosaic disease and two Prunus necrotic ringspot virus biotypes in a central Washington sweet cherry orchard. Plant Dis. 1988;72(7):636–640.
- Uyemoto JK, Scott SW. Important diseases of Prunus caused by viruses and other graft-transmissible pathogens in California and South Carolina. Plant Dis. 1992;76(1):5–11.
- Jones AL, Sutton TB. Prunus necrotic ringspot. In: Diseases of tree fruits in the east. East Lansing (MI): Michigan State University Publishing; 1996. p. 92–93.
- Crosslin JM, Mink GI. Biophysical differences among Prunus necrotic ringspot Ilarviruses. Phytopathology. 1992;82(2):200–206.
- Hammond RW, Crosslin JM. Virulence and molecular polymorphism of Prunus necrotic ringspot virus isolates. J Gen Virol. 1998;79(7):1815–1823.
- Hammond RW. Phylogeny of isolates of Prunus necrotic ring spot virus from the Ilarvirus ringtest and identification of group specific features. Arch Virol. 2003;148(6):1195–1210.
- Salem N, Mansour A, Al-Musa A, et al. Identification and partial characterization of Prunus necrotic ringspot virus on stone fruits in Jordan. J Plant Pathol. 2004;86(1):85–90.
- Paduch-Cichal E, Sala-Rejczak K. Biological and molecular characterization of Prunus necrotic ringspot virus isolates from three rose cultivars. Acta Physiol Plant. 2011;33(6):2349–2354.
- Mink GI, Howell WE, Cole A, et al. Three serotypes of Prunus necrotic ringspot virus isolated from rugose mosaic-diseased sweet cherry trees in Washington. Plant Dis. 1987;71(1):91–93.
- Mink GI. Prunus necrotic ring spot virus. In: Kumer J, Chaube HS, Singh US, Mukhadpadhyay AN, editors. Plant diseases of international importance. Vol. III. New York: Prentice Hall; 1992. p. 335–356.
- Myrta A, Di Terlizzi B, Boscia D, et al. Serological characterization of Mediterranean Prunus necrotic ringspot virus isolates. J Plant Pathol. 2002;83:45–49.
- Scott SW, Zimmerman MT, Ge X, et al. The coat proteins and putative movement proteins of isolates of Prunus necrotic ringspot virus from different host species and geographic origins are extensively conserved. Eur J Plant Pathol. 1998;104(2):155–161.
- Aparicio F, Myrta A, Di Terlizzi B, et al. Molecular variability among isolates of Prunus necrotic ringspot virus from different Prunus spp. Phytopathology. 1999;89(11):991–999.
- Vaskova D, Petrzik K, Karesova R. Variability and molecular typing of the woody-tree infecting Prunus necrotic ringspot Ilarvirus. Arch Virol. 2000;144:699–709.
- Aparicio F, Pallás V. The molecular variability analysis of the RNA 3 of fifteen isolates of Prunus necrotic ringspot virus sheds light on the minimal requirements for the synthesis of its subgenomic RNA. Virus Genes. 2002;25(1):75–84.
- Glasa M, Betinová E, Kúdela O, et al. Biological and molecular characterization of Prunus necrotic ringspot virus isolates and possible approaches to their phylogenetic typing. Ann Appl Biol. 2002;140(3):279–283.
- Kinoti WM, Constable FE, Nancarrow N, et al. Analysis of intra-host genetic diversity of Prunus necrotic ringspot virus (PNRSV) using amplicon next generation sequencing. PLoS ONE. 2017;12(6):e0179284.
- Kamenova I, Borisova A, Popov A. Occurrence of Ilarviruses in sweet and sour cherry in Bulgaria. Bulg J Agric Sci. 2020;26(3):590–597.
- Borisova A. Investigation of biological properties of Prunus necrotic ringspot virus isolates from different stone fruit species. Plant Sci. 2015;LII(3):3–7.
- Kamenova I, Borisova A. Molecular variability of the coat protein gene of Prunus necrotic ring spot virus on sweet and sour cherry in Bulgaria. J Plant Pathol. 2021;103(1):97–104.
- Clark MF, Adams AN. Characteristics of the microplate method of enzyme-linked immunosorbent assay for the detection of plant viruses. J Gen Virol. 1977;34(3):475–483.
- Higgins D, Thompson J, Gibson T. CLUSTAL W: improving the sensitivity of progressive multiple sequence alignment through sequence weighting, position-specific gap penalties and weight matrix choice. Nucleic Acids Res. 1994;22(22):4673–4680.
- Tamura K, Stecher G, Peterson D, et al. MEGA6: molecular evolutionary genetics analysis version 6.0. Mol Biol Evol. 2013;30(12):2725–2729.
- Guo D, Maiss E, Adam G, et al. Prunus necrotic ringspot Ilarvirus: nucleotide sequence of RNA3 and the relationship to other Ilarviruses based on coat protein comparison. J Gen Virol. 1995;76(5):1073–1079.
- Sanchez-Navarro JA, Pallas V. Evolutionary relationships in the ilarviruses: nucleotide sequence of prunus necrotic ringspot virus RNA 3. Arch Virol. 1997;142(4):749–763.
- Fiore N, Fajardo TV, Prodan S, et al. Genetic diversity of the movement and coat protein genes of South American isolates of Prunus necrotic ringspot virus. Arch Virol. 2008;153(5):909–919.
- Abdel-Salam AM, Ibrahim AM, Abdelkader HS, et al. Characterization of two isolates of Prunus necrotic ringspot virus (PNRSV) from peach and apricot in Egypt. Arab J Biotech. 2008;11(1):107–124.
- Damsteegt VD, Stone A, Mink GI, et al. The versatility of Prunus tomentosa as a bioindicator of viruses. Acta Hortic. 1998;472(472):143–146.
- Nemeth M, Szalay-Marzs L, Posnette A. Virus, mycoplasma and rickettsia diseases of fruit trees. Dordrecht: Academia Kiado; 1986.
- Paduch-Cichal E, Sala-Rejczak K. The effect of Prunus necrotic ringspot virus on growth and flowering of the three-field-grown rose cultivars. Phytopathol Pol. 2007;44:27–35.
- Hammond RW, Crosslin JM, Pasini R, et al. Differentiation of closely related but biologically distinct cherry isolates of Prunus necrotic ringspot virus by polymerase chain reaction. J Virol Meth. 1999;80(2):203–212.
- Garcia JA, Pallas V. Viral factors involved in plant pathogenesis. Curr Opin Virol. 2015;11:21–30.