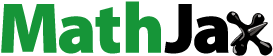
Abstract
Alkaline/neutral invertase (NI) proteins irreversibly break down sucrose into glucose and fructose and play a vital role in carbohydrate and plant growth and development. Currently, a comprehensive understanding of NI genes in Dendrobium catenatum is lacking. After genome-wide identification and prediction, nine DcNI genes were identified and named DcNI1–DcNI9. Through comprehensive analysis of gene structure, amino acid sequence, conserved motif and phylogenetic tree, it was found that the DcNI gene family has a typical conserved region and its amino acid sequence is similar to other plant NI genes. However, DcNI3 lost amino acid sequences at both the N-terminus and C-terminus, while DcNI8 lost amino acids at the C-terminus. Phylogenetic analysis revealed that the DcNI family could be divided into α and β groups. It reveals conservation and divergence of DcNIs. Predictive analysis of cis-acting elements in the promoter region reveals the potential response of DcNI protein to hormones and environmental stress and suggests it to be widely involved in growth and development. DcNIs were differentially expressed in different tissues such as roots, stems and leaves. In particular, DcNI4 was highly expressed in the stem. They also had a differential expression response under drought, cold, P1 fungus infection and jasmonic acid (JA) treatment. To the best of our knowledge, this is the first genome-wide analysis of the DcNI gene family in D. catenatum. This study will provide important and primary information for the functional study of the DcNI gene family and the polysaccharide synthesis pathway in D. catenatum.
Introduction
In higher plants, sucrose is one of the main products of photosynthesis, providing carbohydrates for plant growth [Citation1]. Sucrose metabolism is an important part of the process of polysaccharide synthesis, and current research indicates that three enzymes, sucrose phosphate synthase (SPS), sucrose synthase (SS) and invertase (Inv), are involved in sucrose metabolism. SS catalyzes the reversible conversion of sucrose to UDP-glucose and fructose [Citation2,Citation3], while invertase catalyzes sucrose and irreversibly hydrolyzes it to fructose and glucose [Citation4]. The invertases can be classified according to their optimum pH into acidic and alkaline/neutral invertases (NI). Acid invertases are β-fructosidase with an optimum pH of 4.5–5.0 and are located in cell walls and vesicles. Alkaline/NI are part of a novel family of glucosidases with an optimum pH of 6.5–8.0 and are located in the cytoplasm or organelles [Citation5]. Alkaline/NI require a high degree of substrate specificity, and sucrose appears to be the only substrate for this class of invertases that is not limited by metal ions [Citation6,Citation7]. It is believed that the alkaline/NI originated from cyanobacteria and is closely related to the alkaline/NI of cyanobacteria [Citation8]. Since alkaline/NI are not glycosylated, they are unstable and have low enzymatic activity compared to acid invertases, which leads to a lack of understanding of their function [Citation9]. Alkaline/NI genes have now been identified in a number of species, such as Arabidopsis thaliana [Citation10], Capsicum annuum [Citation11], Populus [Citation12], Camellia sinensis [Citation13], Oryza sativa [Citation14,Citation15], Vitis vinifera [Citation16] and other plants. In addition, plants’ alkaline/NI can be divided into two distinct phylogenetic groups, named α and β groups. The alkaline/NI of the α group are mostly localized in the cytosol, while those of the β group are mostly localized in the cytoplasmic solutes [Citation8]. Alkaline/NI have many physiological functions besides being involved in sucrose metabolism, such as plant growth [Citation17] and responses to environmental stresses in plants [Citation18,Citation19]. Alkaline/NI specifically catalyze only the irreversible decomposition of sucrose and are not limited by heavy metal ions.
Dendrobium catenatum Wall ex Lindl stems are rich in many active substances, for example, polysaccharides, benzyl compounds, amino acids, alkaloids, terpenes, etc., with polysaccharides being the primary active substance [Citation20]. The polysaccharides have significant biological activity and can effectively prevent gastric ulcers, strengthen immunity, lower blood sugar and lipid levels [Citation21,Citation22]. The DoNI1 gene in D. catenatum is highly expressed in the stem, which is consistent with the high polysaccharide content in D. catenatum stems [Citation23], so alkaline/NI is likely to play an important role in D. catenatum polysaccharide synthesis [Citation24]. However, most of the alkaline/NI genes of D. catenatum have not been identified. In this study, members of the alkaline/NI gene family were identified by bioinformatics tools, based on the D. catenatum genome. Protein physicochemical properties, phylogenetic relationships, exon–intron structures, motif distributions were investigated. These results contribute to further investigations into the role of alkaline/NI in the synthesis of D. catenatum polysaccharides.
Materials and methods
Plant materials
D. catenatum cultivar ‘Jingpin No. 1’ (Breed No. Zhe R-SV-DO-015-2014) was from the State Key Laboratory of Subtropical Silviculture in Zhejiang Province, China. Fresh roots, stems and leaves from the plants were collected and frozen in liquid nitrogen for subsequent RNA isolation.
DcNI gene identification and sequence analysis in D. catenatum
Data were downloaded from the TAIR database (<seurld>https://www.arabidopsis.org/</seurld>). To identify the DcNI gene, the alkaline/NI protein sequence from Arabidopsis database was downloaded and the nucleotide sequence in FASTA format. The obtained CDS coding sequences of Arabidopsis were compared with the whole genome protein database sequences of D. catenatum through the BLASTX program of NCBI-BLAST 2.9.0 software to find the homologous protein sequence encoded by the NI gene with an expected value (e-value) of default parameter. The seed file of domain was downloaded from the Pfam database [Citation25], and the candidate NI protein sequence was analysed by HMMER software [Citation26], and NI gene was further screened and renamed as DcNI by HMM hidden Markov model algorithm. Amino acid number, molecular weight (Mw), instability index, grand average of hydropathicity and theoretical isoelectric point (pI) were predicted on the EXPASY server (<seurld>http://www.expasy.org/tools/protparam.html</seurld>). All the resulting protein sequences were confirmed by Pfam (<seurld>http://pfam.xfam.org/search</seurld>) and SMART (<seurld>http://smart.embl-heidelberg.de/</seurld>). The subcellular localization of DcNIs was predicted using TargetP1.1 Server (<seurld>http://www.cbs.dtu.dk/services/TargetP/</seurld>, version 1.1 server). The protein secondary and tertiary structures were predicted by SOPMA (<seurld>http://nhjy.hzau.edu.cn/kech/swxxx/jakj/dian</seurld > zi/Bioinf7/Expasy/Expasy8.htm) and Phyre2 (<seurld>http://www.sbg.bio.ic.ac.uk/phyre2/html/page.cgi?id=index</seurld>). The amino acid sequences of DcNIs were analysed by ClustalX2 and GeneDoc software. The catalytic and subunit-binding residues of DcNI proteins are depicted in the same way as the alkaline/NI proteins of Capsicum annuum [Citation11] and Populus [Citation12].
Phylogenetic analysis
Multiple sequence alignment of protein sequences of 54 NIs genes from A. thaliana, D. catenatum, P. trichocarpa, M. esculenta, P. equestris and O. sativa were analysed using MUSCLE software. We optimized the obtained results and constructed a neighbor-joining (NJ) tree using FastTree version 7.0 + [Citation27]. Bootstrap analysis was used to estimate the reliability of the tree, with 1000 bootstrap replicates.
Exon–intron structure and conserved motif analysis
We downloaded the genome structure annotated GFF file from the D. catenatum database and visualized the DcNIs gene structure map using TBtools software [Citation28]. The MEME online program (<seurld>http://meme-suite.org/tools/meme</seurld>, version 5.1.1) was used to predict the potential motifs of the alkaline/NI protein sequence in D. catenatum. The number of different topics was set at 15; the other parameters were set at the default values.
Cis-acting elements in the promoter analysis
We downloaded the D. catenatum genome from the NCBI database (<seurld>https://www.ncbi.nlm.nih.gov/</seurld>), screened through TBtools to obtain the nucleotide sequence 2000 bp upstream of the start codon and submitted it to the PlantCare (<seurld>http://bioinformatics.psb.ugent.be/webtools/plantcare/html/</seurld>) website [Citation29] to predict cis-acting components and obtain the screening and sorting of the results and visualized them with TBtools.
RNA extraction and analysis of gene expression patterns
For DcNI4 gene expression pattern analysis, roots, stems and leaves were collected from 6-month-old D. catenatum and immediately placed in liquid nitrogen. Total RNA from all samples was extracted using the TaKaRa MiniBEST Plant RNA Extraction Kit. First-strand cDNA synthesis was performed using the PrimeScript™ RT Kit with gDNA Eraser (Perfect Real Time). Real-time quantitative reverse transcription-polymerase chain reaction (PCR) was performed using the CFX96TM Real-Time PCR System. The reaction volume consisted of 5 µL TB Green Premix Ex Taq II (Tli RNaseH Plus) (2X), 0.8 µL cDNA, 0.4 µL upstream primer (10 µM), 0.4 µL downstream primer (10 µmol/L) and 3.4 µL ddH2O (10 µL total). The reaction was performed according to the following cycling procedure: 95 °C for 3 min, 40 cycles of 94 °C for 40 s, 60 °C for 20 s and 72 °C for 20 s. Three technical replicates were performed for each sample. Gene expression levels were calculated following the method described by Livak and Schmittgen [Citation30]. The results were normalized to the actin gene of D. catenatum.
Based on the transcriptome data of D. catenatum provided by our research group, we screened out the expression of the DcNI gene family under drought and low temperature conditions. We drew the heat map by using TBtools Heat map function [Citation28]. The drought treatment was based on the method of Zou et al. [Citation31]. Tissue-cultured D. catenatum plants from seeds were grown in plastic pots (8.0 cm in diameter) filled with a substrate mix of composted pine bark and small stones. The plants were kept in a growth chamber with a photoperiod of 12 h day and 12 h night, a light intensity of 100 µmol m−2s−1, a temperature of 28 °C day and 22 °C night, a relative humidity of 50% day and 70% night, and watering every 2 days at 15:30 in the afternoon. Eight-month-old vigorous plants with a height of 12 cm were selected for subsequent experiments. Irrigation was carried out on the first day, omitted from the second to the seventh day, and restarted on the eighth day. The mature fourth leaf from the apex of each individual was harvested at both 06:30 in the morning and 18:30 in the afternoon (half an hour after light on and off, respectively) on the second (DR5, DR6), seventh (DR7, DR8) and ninth (DR11, DR15) days and at 18:30 in the afternoon on the eighth day (DR10). Each sample time point included only one biological replicate. In the low temperature treatment, the treatment conditions of the control group were 20 °C, while the experimental group were 0 °C, and the culture time was 20 h; each group had three repetitions. P1 treatment was done by spraying Dendrobium zygomycetes on 1-month-old leaves, incubation at 30 °C and humidity greater than 90% for a total of 24 h. The leaves were taken. Jasmonic acid (JA) treatment was done by spraying 100 µmol/L MeJA on 1-month-old Dendrobium leaves, incubation at 30 °C and humidity greater than 90% for a total of 28 h. The leaves were taken. All the above samples were frozen immediately in liquid nitrogen and stored at −80 °C.
Cloning of full-length DcNI4
The full-length cDNA of the DcNI4 gene was cloned by reverse transcription PCR using gene-specific primers: DcNI4-F (5′-CCGCA AGCTTGTCGAATGGAAGG GACCTCATGCATCAG-3′) and DcNI4-R (5′-TTC GAGCTCCGTCGAGCATG TCCAGGAAGTAG ATCTCTTG-3′). Extraction of total RNA was performed as above.
Results
Identification and characterization of the DcNI gene family in D. catenatum
BLASTP search against the D. catenatum genome database was performed based on the sequences of alkaline/NI genes that have been reported for A. thaliana. Nine alkaline/NI genes were identified in the D. catenatum genome; they were named DcNI1 to DcNI9. The deduced size of the DcNI proteins was between 412 (DcNI3) and 651 (DcNI9) amino acids (aa). The Mw varied from 47.05 to 73.21 kDa. Their pI was predicted to be less than 7, so they are acidic proteins. The instability coefficient is a measure of the stability of the protein. When the instability coefficient is greater than 40, the stability is poor. The instability coefficient of the DcNI proteins was calculated to be greater than 40, indicating that the stability of DcNI proteins is poor. From the above, the total average hydrophilic coefficient of DcNI proteins was less than 0, indicating that they are hydrophobic proteins (). The amino acid sequence comparison analysis showed that DcNIs were less similar at the N-terminus than at the C-terminus (). Similar to Nis from Salvia miltiorrhiza, DcNIs contain multiple conserved residues, including catalytic residues (N160, Y161, F165, R167, D168, I243, M307, R310, Y491, H492, Q553 and W555) and residues that may bind to substrates (D3309 and E535; numbers are based on DcNI1).
Figure 1. Amino acid sequence alignment of nine alkaline/neutral invertases in D. catenatum. Dark blue shading, pink shading and light blue shading reflect 100%, 75% and 50% conserved amino acid residues, respectively. The catalytic residues and substrate-binding residues are indicated by blue and red arrows, respectively.
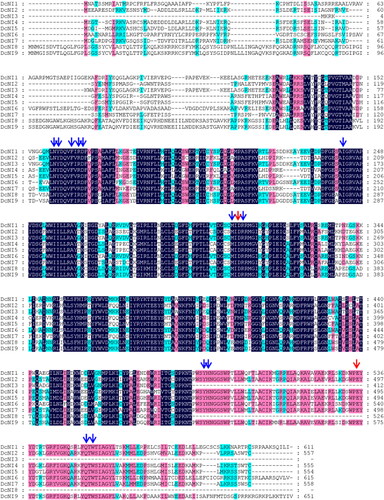
Table 1. Composition and physicochemical character analysis based on the predicted amino acid sequences of alkaline/neutral invertases (DcNI) in D. catenatum.
Prediction and analysis of the secondary structure of DcNI proteins in D. catenatum
The secondary structure of proteins mainly includes α-helix, β-fold, β-turn, random coil and extended strand, which are predicted by the online software SOPMA. The prediction results showed that DcNI proteins were composed of four structures (α-helix, β-fold, random coil and extended strand), and the proportion of each structure was similar. Among them, α-helices and random coils are the main components, and the proportion of β-folds is the smallest ().
Table 2. Predicted proportion of main components in the secondary structure of nine alkaline/neutral invertases in D. catenatum.
Phylogenetic analysis of DcNIs
To determine the evolutionary relationships among alkaline/NI of D. catenatum (DcNI) proteins, we constructed a NJ phylogenetic tree, sequences of 54 NI family members from six species (A. thaliana, D. catenatum, P. trichocarpa, M. esculenta, P. equestris, O. sativa) by using FastTree online software [Citation32]. These protein sequences were divided into two groups (α and β) (). The α group contains DcNI1, DcNI3, DcNI6, DcNI8 and DcNI9. The β group contains DcNI2, DcNI4, DcNI5 and DcNI7. In the α group, DcNI1 and DcNI3 are closely related to each other and are assigned to α1. DcNI6 and OsNI1 are also closely related to each other and are assigned to α1. DcNI8 and 9 have an amino acid similarity of 80.8% and are therefore assigned to α2. In the β group, DcNI4 and DcNI5 were closely related to AT1G35580 and At4G09510 and were assigned to β2. DcNI2 and DcNI7 form a branch, PeNI1 and PeNI2 form a branch, these four proteins are in group β2. DcNI2, DcNI7, PeNI1 and the amino acid sequence after 536 of PeNI2 have 90.4% similarity of amino acids. The other PeNIs in the same branch of DcNIs have similar positions. The great similarity between DcNI and PeNI indicates a high degree of relatedness between the two species, which is consistent with previous reports [Citation23]. The α1 group mainly includes AT1G56560, AT3G05820, AT3G06500, DcNI1, DcNI3 and DcNI6. The function of AT1G56560, AT3G05820 and AT3G06500 has been reported. Shoot growth was severely reduced, but root development was not affected after knock out these genes. Those two genes (AT1G56560, AT3G05820) may regulate the hormonal balance related to the emergence of radicle [Citation32,Citation33]. The α2 group mainly includes AT5G22510, DcNI8 and DcNI9. The AT5G22510 gene regulates plant greening and carbon and nitrogen balance, and sugar metabolism plays an important role in plant development and metabolism regulation [Citation34]. In addition, among AT1G35580, AT4G09510, AT1G7200, AT1G22650, DcNI2, DcNI4, DcNI5 and DcNI7 in β2 group, AT1G35580 and AT4G09510 gene have been reported to support plant regeneration, development [Citation35]. However, AT1G7200 and AT1G22650 play an important role in the resistance to stress and plant growth and development. Enzymes in mitochondria and cytoplasm can catalyze the production of glucose, which contributes to the homeostasis of mitochondrial reactive oxygen species [Citation36].
Figure 2. Phylogenetic analysis of 52 alkaline/neutral invertase (NI) proteins from A. thaliana, D. catenatum, P. trichocarpa, M. esculenta, P. equestris and O. sativa. The phylogenetic tree was constructed by the neighbor-joining method (1000 bootstrap replicates) using FastTree version 7.0 + [Citation27]. Yellow shadows indicate the NIs from D. catenatum.
![Figure 2. Phylogenetic analysis of 52 alkaline/neutral invertase (NI) proteins from A. thaliana, D. catenatum, P. trichocarpa, M. esculenta, P. equestris and O. sativa. The phylogenetic tree was constructed by the neighbor-joining method (1000 bootstrap replicates) using FastTree version 7.0 + [Citation27]. Yellow shadows indicate the NIs from D. catenatum.](/cms/asset/a10fd30c-d70e-4e11-be0d-5a77e4d0724b/tbeq_a_1901610_f0002_c.jpg)
Structure analysis of DcNI family genes
To further understand the evolutionary relationships of DcNIs, the exon–intron structure of each gene in the family was analysed. The number of exons in the DcNI gene is four or six; DcNI2, DcNI3, DcNI4, DcNI5 and DcNI7 have four exons and DcNI1, DcNI6, DcNI8 and DcNI9 have six exons. The fifth exon is the smallest of these ().
Motif distribution of DcNI proteins in D. catenatum
To further investigate the structural features of DcNI proteins in D. catenatum, we analysed the motifs of DcNIs. Their motif distributions were similar (). Most of the motifs were highly conserved. Motifs 1, 2, 3, 4, 5, 6, 7, 9, 10 and 11 were widely distributed among all the analysed NI proteins. Motifs 13, 14 and 15 were specifically distributed in the α group. Within the α group, both DcNI3 and DcNI8 lost motif 8 at the N-terminus; and all the motifs of these three proteins (DcNI1, DcNI3 and DcNI6) were clearly distributed at the C-terminus differently from the other proteins in the α group, in association with their being in different subgroups. In the β group, DcNI7 lost motif 12 at the C-terminus ().
Figure 4. Distribution of conserved motifs of NI proteins from D. catenatum. (A) Sequence identification by MEME resulted in the identification of 15 motifs. (B) Distribution of conserved motifs in DcNIs. The NJ tree was constructed with full-length amino acid sequences of DcNIs using MEGA7 software [Citation37], with 1000 bootstraps. Each motif is indicated and numbered by a coloured box, the size of which indicates the length of the motif.
![Figure 4. Distribution of conserved motifs of NI proteins from D. catenatum. (A) Sequence identification by MEME resulted in the identification of 15 motifs. (B) Distribution of conserved motifs in DcNIs. The NJ tree was constructed with full-length amino acid sequences of DcNIs using MEGA7 software [Citation37], with 1000 bootstraps. Each motif is indicated and numbered by a coloured box, the size of which indicates the length of the motif.](/cms/asset/fd62512f-885c-45a5-afc1-89f6e773460a/tbeq_a_1901610_f0004_c.jpg)
Analysis of cis-acting elements in the promoter of DcNI genes
Cis-acting elements are involved in the regulation of gene expression and do not encode any protein parts. Promoters are important cis-acting elements that control transcription. For this reason, PlantCARE was used to analyse the 2000 bp sequence in the promoter region of the DcNI genes, and the results showed that the promoter regions of the 9 DcNI genes mostly contained multiple cis-acting elements (). These cis-acting elements are roughly divided into eight types: development-related elements, hormone-responsive elements, environmental stress-related elements, light-responsive elements, plant growth and development elements and other elements. All genes are rich in light response elements, a total of 16 types. They also include response elements involved in plant hormone responses, the MeJA-response element (CGTGA-motif), auxin-responsive element (TGA-element), ethylene-responsive element (ERE), abscisic acid-responsive element (ABRE), auxin-responsive element (AuxRR-core), p-box, gibberellins-responsive element (GARE-motif), salicylic acid-responsive element (TCA-element), CGTCA-motif and TGACG-motif. The cis-acting elements also include drought response element (MBS), low-temperature-responsive element (LTR), wound-responsive element (WUN-motif) and anaerobic induction (ARE), so DcNIs may respond to plant hormones and environmental stress. DcNIs are also related to plant growth and development, such as cis-acting regulatory elements related to meristem expression (CAT-box), involved in endosperm expression (GCN4_motif), involved in zein metabolism regulation (O2-site), binding site of AT-rich DNA binding protein (AT-rich element), involved in seed-specific regulation (RY-element), protein binding site (HD-Zip 3).
Expression analysis of DcNI genes for different tissues and developmental stages
DcNI genes play an important role in plant growth and development [Citation1,Citation17,Citation18]. We examined their expression using qRT-PCR to elucidate the expression of DcNIs genes in different tissues (root, stem and leaf). We used 6-month-old D. catenatum seedlings and extracted RNA from root, stem and leaf for qRT-PCR. During this period, we designed three biological replicates and three technical replicates for each sample to ensure the accuracy of the experiment (). The results showed that most of the genes were highly expressed in the stem and leaf, whereas DcNI3 and DcNI5 were highly expressed in the root, and DcNI2, DcNI6 and DcNI9 were much more highly expressed in the leaf than in other genes. DcNI4 was highly expressed in the stem and root.
Figure 6. Relative expression of DcNIs in roots (R), stems (S) and leaves (L) of D. catenatum. The expression level in roots was arbitrarily set to 1 and the levels in other organs were given relative to this.
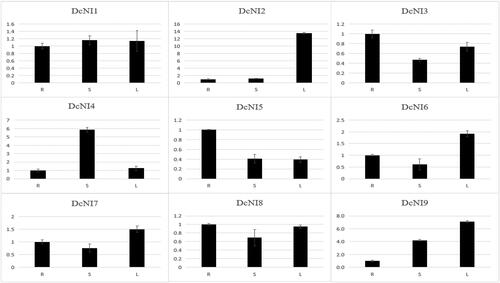
Previous studies have shown that NI genes are involved in plant responses to environmental stresses [Citation18,Citation19]. In order to study the response of DcNIs under stress conditions, the tissue transcript levels following drought, low temperature, P1 fungal infection and JA treatment were obtained from the available transcriptome data (). The expression of DcNI4, DcNI5, DcNI6, DcNI8 and DcNI9 genes was high under drought conditions and remained high at the beginning of rehydration; however, no expression of DcNI7 was detected. Under cold conditions, DcNI1, DcNI3 and DcNI5 were significantly down-regulated, DcNI4 was up-regulated, and DcNI8 and DcNI9 were significantly up-regulated after 20 h of 0 °C treatment compared with the control (20 °C, 20 h). This indicates that DcNIs may perform different functions in the face of environmental stress. Under the treatment of JA and P1 fungus infection, the expression of DcNI1 and DcNI3 was significantly up-regulated, and the expression of DcNI1 and DcNI3 was still high following treatment with JA only.
Figure 7. Heat map showing the expression of DcNI gene family members under different environmental stresses. (A) Expression of DcNI after drought treatment, DR5/DR6: The second-day treatment at 06:30 and 18:30; DR7/DR8: The seventh-day treatment at 06:30 and 18:30; DR10: The eighth-day treatment at 18:30 after watering the seedlings at 15:30; DR11/DR15: The ninth-day treatment at 06:30 and 18:00. (B) Expression of DcNI after low temperature treatment. Control 1 ∼ 3: 20 °C, 20 h; Cold 1 ∼ 3: 0 °C, 20 h. Each group had three repetitions. (C) Expression of DcNI after P1 fungus infection, JA treatment and P1 fungus infection and JA co-treatment.
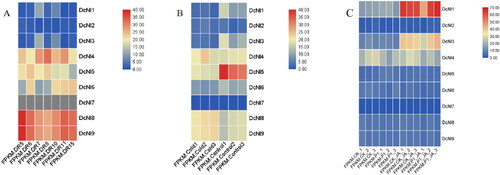
Discussion
Identification and characterization of the DcNI gene family
Alkaline/NI were previously reported to play an important role in sugar metabolism during plant development [Citation24]. However, no more information is available on the D. dendrobium alkaline/NI gene family, and only two genes have been cloned and studied [Citation23]. According to previous studies on other species, there are species differences in the members of the alkaline/NI gene family, such as A. thaliana with nine members [Citation10], Capsicum annuum with seven members [Citation11] and Populus with 12 members [Citation12]. The present study found the identification of nine alkaline/NI (DcNI1–DcNI9) in D. catenatum (). The Mw of DcNIs proteins ranged from 47.05 to 73.21 kDa.
All DcNI proteins contained multiple conserved residues (), which is consistent with the NI reported for other plants. DcNI3 lost amino acid sequences at both the N-terminus and C-terminus, while DcNI8 lost amino acids at the C-terminus. Phylogenetic analysis of the 52 NI proteins from six plants showed that DcNIs can be divided into α and β groups. Only DcNI1 and DcNI6 in the α group are located in chloroplasts and mitochondria (, ), which is consistent with previous studies [Citation11]. Members of the same group have similar exon–intron structures. The exon–intron structures of the α and β groups are significantly different, suggesting that members of the two groups may have evolved from different ancestors [Citation38] (). However, DcNI3 in contrast to other members of the same group is missing amino acid sequences at both the C-terminus and N-terminus, and its exon–intron structure is also missing two UTRs, and it lacks motif 8 at the N-terminus, which may have undergone a natural mutation. In the α2 group, the DcNI8 and DcNI9 motif distributions are almost identical and both specifically contain motifs 13 and 15, whereas the α1 group does not have these two motifs, but DcNI8 lacks motif 8 at the N-terminus. In the β group members, they would lack more motifs (motifs 13, 14 and 15). Studies have shown that AT1G35580 and AT4G09510 play an important role in the development of Arabidopsis roots [Citation39]. DcNI4 and DcNI5 have a close phylogenetic relationship with these two. It can be speculated that DcNI4 and DcNI5 may affect the growth and development of plant roots.
The analysis of cis-acting elements in the promoter shows that DcNIs may be related to plant growth and development, the response to environmental stress and the response to phytohormones.
Differential expression of DcNI in different tissues
In order to understand the function of DcNI gene in the development of D. catenatum, the RNA from roots, stems and leaves of D. catenatum grown for 6 months was extracted, and the expression pattern of the DcNI genes in different tissues of D. catenatum was determined by qRT-PCR (). All DcNI genes were widely expressed. Most DcNI genes were highly expressed in stems and leaves. This may be due to the vigorous growth of D. catenatum for 6 months. The high expression of the DcNI genes provides the sugars needed for the growth of stems and leaves. The expression level of the DcNI4 gene in stems was significantly higher than that in roots and leaves, which is consistent with the traits of high polysaccharide content in D. catenatum stems [Citation40]. We speculate that the DcNI4 gene plays an important role in the synthesis of polysaccharides as previously suggested about an NI gene in cassava [Citation41]. The DcNI2 and DcNI7 expression levels were very low or undetectable. In mature primary wheat leaves, the Ta-A-Inv gene is expressed under cold stress [Citation42]. The expression of DcNI1, DcNI3, DcNI5, DcNI8 and DcNI9 was significantly down-regulated when induced by cold and low temperature, which may be due to plants blocking the decomposition of sucrose to resist the adverse cold environment. Under the treatment of JA and P1 fungus infection, the expression of DcNI1 and DcNI3 was significantly up-regulated, and the expression was still high when only JA treatment was applied. Only after P1 infection, the gene expression level did not change significantly, indicating that the reason for the up-regulation of DcNI1 and DcNI3 was the JA treatment. It is speculated that there are JA-responsive cis-acting elements in these two genes. According to reports, the expression of NI genes SmNINV8 and SmNINV9 in Salvia miltiorrhiza also was up-regulated by MeJA induction [Citation42], while under drought stress conditions, there was no significant change in gene expression after dehydration and rewatering. It is possible that the DcNI genes may not be involved in the regulation under drought conditions.
Conclusions
Nine DcNI genes were identified in D. catenatum, which were divided into two groups: α and β and further divided into subgroups: α1, α2, β1, β2 and β3, probably indicating the functional differentiation of DcNIs. Multiple alignments of amino acids, exon–intron structure and motif analysis suggested that the NI gene family is evolutionarily conserved. The promoter of DcNIs is rich in a variety of cis-acting elements. For example, it was predicted to respond to plant hormones (gibberellin, MeJA, salicylic acid), plays a role under stress (drought, cold, pathogen), and is associated with the regulation of plant growth and development. The qRT-PCR analysis showed that the DcNI genes are expressed in roots, stems and leaves. The expression levels of some genes had significant differences in these three organs. Generally speaking, the expression in stems and leaves was relatively higher than that in roots. The expression analysis showed that most DcNI genes are expressed when they are under drought and cold stress, and they perform different functions. Under drought conditions, DcNI4, DcNI5, DcNI6, DcNI8 and DcNI9 genes were highly expressed. Under cold conditions, DcNI1, DcNI3 and DcNI5 were significantly down-regulated, DcNI4, DcNI8 and DcNI9 were all up-regulated. Our research results provide new and effective information for further understanding of the function of the DcNI gene family and the polysaccharides metabolic pathway in D. catenatum.
Author contributions
Conceptualization, C.L. and L.Z.; methodology, C.L.; formal analysis, C.L.; investigation, C.L.; writing – original draft preparation, C.L.; writing – review and editing, C.L., L.Z. J.S., H.X., X.C., Y.Z. and J.Y.; supervision, L.Z.; funding acquisition, J.S and L.Z. All authors have read and agreed to the published version of the manuscript.
Disclosure statement
The authors declare no conflict of interest.
Data sharing
All data that support the findings reported in this study are available from the corresponding author upon reasonable request.
Additional information
Funding
Funding
References
- Qin G, Zhu Z, Wang W, et al. A tomato vacuolar invertase inhibitor mediates sucrose metabolism and influences fruit ripening. Plant Physiol. 2016;172(3):1596–1611.
- Ruan YL. Sucrose metabolism: gateway to diverse carbon use and sugar signaling. Annu Rev Plant Biol. 2014;65:33–67.
- Coleman HD, Yan J, Mansfield SD. Sucrose synthase affects carbon partitioning to increase cellulose production and altered cell wall ultrastructure. Proc Natl Acad Sci USA. 2009;106(31):13118–13123.
- Sturm A. Invertases. Primary structures, functions, and roles in plant development and sucrose partitioning. Plant Physiol. 1999;121(1):1–7.
- Roitsch T, Gonzalez MC. Function and regulation of plant invertases: sweet sensations. Trends Plant Sci. 2004;9(12):606–613.
- Vargas W, Cumino A, Salerno GL. Cyanobacterial alkaline/neutral invertases. Origin of sucrose hydrolysis in the plant cytosol?Planta. 2002;216(6):951–960.
- Roitsch T, Balibrea ME, Hofmann M, et al. Extracellular invertase: key metabolic enzyme and PR protein. J Exp Bot. 2003;54(382):513–524.
- Vargas WA, Salerno GL. The Cinderella story of sucrose hydrolysis: alkaline/neutral invertases, from cyanobacteria to unforeseen roles in plant cytosol and organelles. Plant Sci. 2010;178(1):1–8.
- Pelleschi S, Rocher J-P, Prioul J-L. Effect of water restriction on carbohydrate metabolism and photosynthesis in mature maize leaves. Plant Cell Environ. 1997;20(4):493–503.
- The Arabidopsis Genome Initiative. Analysis of the genome sequence of the flowering plant Arabidopsis thaliana. Nature. 2000;408(6814):796–815.
- Shen L-B, Yao Y, He H, et al. Genome-wide identification, expression, and functional analysis of the alkaline/neutral invertase gene family in pepper. Int J Mol Sci. 2018;19(1):224–238.
- Chen Z, Gao K, Su X, et al. Genome-wide identification of the invertase gene family in Populus. PLoS One. 2015;10(9):e0138540.
- Qian W, Yue C, Wang Y, et al. Identification of the invertase gene family (INVs) in tea plant and their expression analysis under abiotic stress. Plant Cell Rep. 2016;35(11):2269–2283.
- Murayama S, Handa H. Genes for alkaline/neutral invertase in rice: alkaline/neutral invertases are located in plant mitochondria and also in plastids. Planta. 2007;225(5):1193–1203.
- Ji X, Van den Ende W, Van Laere A, et al. Structure, evolution, and expression of the two invertase gene families of rice. J Mol Evol. 2005;60(5):615–634.
- Nonis A, Ruperti B, Pierasco A, et al. Neutral invertases in grapevine and comparative analysis with Arabidopsis, poplar and rice. Planta. 2008;229(1):129–142.
- Tang G-Q, Lüscher M, Sturm A, et al. Antisense repression of vacuolar and cell wall invertase in transgenic carrot alters early plant development and sucrose partitioning. Plant Cell. 1999;11(2):177–189.
- Vargas WA, Pontis HG, Salerno GL. Differential expression of alkaline and neutral invertases in response to environmental stresses: characterization of an alkaline isoform as a stress-response enzyme in wheat leaves. Planta. 2007;226(6):1535–1545.
- Zhang X, Dong J, Liu H, et al. Transcriptome sequencing in response to salicylic acid in Salvia miltiorrhiza. PLoS One. 2016;11(1):e0147849.
- Ye Z, Dai J-R, Zhang C-G, et al. Chemical differentiation of Dendrobium officinale and Dendrobium devonianum by using HPLC fingerprints, HPLC-ESI-MS, and HPTLC analyses. Evid Based Complement Alternat Med. 2017;2017(13):8647212–8647219.
- Yu Z, He C, Teixeira da Silva JA, et al. The GDP-mannose transporter gene (DoGMT) from Dendrobium officinale is critical for mannan biosynthesis in plant growth and development. Plant Sci. 2018;277:43–54.
- Tang H, Zhao T, Sheng Y, et al. Dendrobium officinale Kimura et Migo: a review on its ethnopharmacology, phytochemistry, pharmacology, and industrialization. Evid Based Complement Alternat Med. 2017;2017:7436259.
- Gao F, Cao XF, Si JP, et al. Characterization of the alkaline/neutral invertase gene in Dendrobium officinale and its relationship with polysaccharide accumulation. Genet Mol Res. 2016;15(2):1–8.
- Li M, Feng F, Cheng L. Expression patterns of genes involved in sugar metabolism and accumulation during apple fruit development. PLoS One. 2012;7(3):e33055.
- El-Gebali S, Mistry J, Bateman A, et al. The Pfam protein families database in 2019. Nucleic Acids Res. 2019;47(D1):D427–D432.
- Finn RD, Clements J, Eddy SR. HMMER web server: interactive sequence similarity searching. Nucleic Acids Res. 2011;39(Web Server issue):W29–W37.
- Price MN, Dehal PS, Arkin AP. FastTree: computing large minimum evolution trees with profiles instead of a distance matrix. Mol Biol Evol. 2009;26(7):1641–1650.
- Chen C, Chen H, Zhang Y, et al. TBtools: an integrative toolkit developed for interactive analyses of big biological data. Mol Plant. 2020;13(8):1194–1202.
- Stephane R, Patrice D, Marc VM, et al. PlantCARE, a plant cis-acting regulatory element database. J Nucleic Acids Res. 1999;27(1):295–296.
- Livak KJ, Schmittgen TD. Analysis of relative gene expression data using real-time quantitative PCR and the method. Methods. 2001;25(4):402–408.
- Zou L-H, Wan X, Deng H, et al. RNA-seq transcriptomic profiling of crassulacean acid metabolism pathway in Dendrobium catenatum. Sci Data. 2018;5:180252.
- Battaglia ME, Martin MV, Lechner L, et al. The riddle of mitochondrial alkaline/neutral invertases: a novel Arabidopsis isoform mainly present in reproductive tissues and involved in root ROS production. PLoS One. 2017;12(9):e0185286.
- Martin ML, Lechner L, Zabaleta EJ, et al. A mitochondrial alkaline/neutral invertase isoform (A/N-InvC) functions in developmental energy-demanding processes in Arabidopsis. Planta. 2013;237(3):813–822.
- Maruta T, Otori K, Tabuchi T, et al. New insights into the regulation of greening and carbon-nitrogen balance by sugar metabolism through a plastidic invertase. Plant Signal Behav. 2010;5(9):1131–1133.
- Siddappaji MH, Scholes DR, Krishnankutty SM, et al. The role of invertases in plant compensatory responses to simulated herbivory. BMC Plant Biol. 2015;15:278–290.
- Xiang L, Le Roy K, Bolouri-Moghaddam M-R, et al. Exploring the neutral invertase-oxidative stress defence connection in Arabidopsis thaliana. J Exp Bot. 2011;62(11):3849–3862.
- Huang K, Li Y, Tao S, et al. Purification, characterization and biological activity of polysaccharides from Dendrobium officinale. Molecules. 2016;21(6):701–718.
- Sturm A, Chrispeels MJ. cDNA cloning of carrot extracellular β-fructosidase and its expression in response to wounding and bacterial infection. Plant Cell Online. 1990;2(11):1107–1119.
- Barratt DHP, Derbyshire P, Findlay K, et al. Normal growth of Arabidopsis requires cytosolic invertase but not sucrose synthase. Proc Natl Acad Sci USA. 2009;106(31):13124–13129.
- Xing X, Cui SW, Nie S, et al. Study on Dendrobium officinale O-acetyl-glucomannan (Dendronan®): part II. Fine structures of O-acetylated residues. Carbohydr Polym. 2015;117:422–433.
- Yao Y, Wu X-H, Geng M-T, et al. Cloning, 3D modeling and expression analysis of three vacuolar invertase genes from cassava (Manihot esculenta Crantz). Molecules. 2014;19(5):6228–6245.
- Zhou H, Li C, Qiu X, et al. Systematic analysis of alkaline/neutral invertase genes reveals the involvement of Smi-miR399 in regulation of SmNINV3 and SmNINV4 in Salvia miltiorrhiza. Plants 2019;8(11):490–511.