Abstract
Anion-exchange chromatography (Hamilton, PRP-X100) with hydride-generation atomic fluorescence spectrometry (HG-AFS) is commonly used for selenium (Se) speciation analysis in environmental and biological samples. However, retentions of Se species are often different with different mobile phases. To address it, this study systematically investigated mobile phases based on ammonium salts to obtain good resolution of Se species. Various mobile phases, including NH4H2PO4, NH4Ac, NH4NO3 and (NH4)2HPO4, were tested. The best result was obtained with a mobile phase containing 60 mmol L−1 (NH4)2HPO4 at pH 6.0, where the separation of Se species, including selenite [Se(IV)], selenate [Se(VI)], selenocystine (SeCys) and selenomethionine (SeMet), was achieved within 10 min with reasonable resolution. In addition, the recoveries of SeCys, Se (IV), SeMet and Se (VI) in the Se-enriched samples were 95.72 ± 1.60%, 106.89 ± 2.20%, 93.28 ± 2.82% and 91.38 ± 1.27%, respectively. Finally, the proposed method was validated for the determination of Se species in Nicotiana tabacum tissues.
Introduction
Selenium (Se) is an essential element for human beings and animals. However, it is toxic once the critical concentration is exceeded. The beneficial or toxic effect of Se on humans and animals is not only dose-dependent, but is also related to the chemical form and the bioavailability of Se [Citation1, Citation2]. In living organisms, Se exists in non-volatile inorganic Se (selenite and selenate) and organic Se (SeMet) forms [Citation3, Citation4]. Hence, the development of analytical methods of Se speciation is required to better understand the Se metabolism in living organisms. A number of analytical techniques are often used for the determination of Se species, including graphite furnace atomic absorption spectrometry [Citation5, Citation6], synchrotron X-ray fluorescence microtomography [Citation7], X-ray spectroscopy [Citation8–10], gas chromatography [Citation11, Citation12], capillary electrophoresis [Citation13, Citation14] and anion exchange HPLC chromatography [Citation15, Citation16].
Ion chromatography with hydride-generation atomic fluorescence spectrometry (HG-AFS) is commonly used for the speciation of Se in environmental and biological samples [Citation17–19]. Anion-exchange chromatography is frequently used for the determination of anionic Se species, where these species are retained on the column by anion exchange and eluted by competitive anions in mobile phases. The anion-exchange columns such as the PRP-X100 column are often used for the separation of anionic species. However, widely different retentions of Se speciation were obtained from the PRP-X100 column as various mobile phases were employed. For example, da Silva et al. [Citation20]developed a method based on a mobile phase containing 25 and 250 mmol L−1 ammonium acetate buffer at pH 5.17, and used it to determine the Se speciation in plankton, Brazil nut and human urine. Sánchez-Martínez et al. [Citation15] proposed a method using a mobile phase containing 10 mmol L−1 ammonium citrate at pH 5 for the determination of Se in yeast. Sanchez-Rodas et al. [Citation21] utilized 80 mmol L−1 KH2PO4/K2HPO4 buffer pH 6.0 as the mobile phase for the separation of Se species in algae. Three selenium species including selenocystine (SeCys), selenomethionine (SeMet) and selenite Se(IV), were separated on an anion-exchange column (PRP-X100) with eluent of 30 mmol L−1 NH4H2PO4 [Citation22]. Chen et al. [Citation23] proposed that ammonium salts could be determined using the ICP-MS detection of Se speciation, which decomposed to volatile substances under the plasma conditions and therefore there was no deposition of salts and no erosion of sampler and skimmer cones after an operation period of several days. However, a high resolution of Se speciation is required and hence a systematic study on the suitable mobile phase for Se speciation is conducted to elucidate the relationship between Se retention and various mobile phases based on ammonium salts.
For these reasons, the objective of this study was to systematically investigate the relationship between Se retention and the eluent based on ammonium salt to address the limitations of current determination of Se speciation in plant tissues. To achieve the objective, the following aspects were considered: (1) to explore the relation between Se retention and the mobile phases using a PRP-X100 column, (2) to reduce the residue left on the sampler and skimmer cones of HPLC-AFS using an ammonium salt as the eluent, and (3) to use the proposed method for the analysis of Se speciation in real Nicotiana tabacum samples.
Materials a]nd methods
Experimental materials
The seeds of N. tabacum (cultivar: K326) were provided by Tobacco Scientific Research Institute of Hubei, China, and the soil (yellow brown) was taken from Xiangyang, Hubei province, China. Soil samples were air-dried at room temperature for 2 weeks, and passed through a 2-mm sieve prior to laboratory analysis. Soil pH (1:2.5 soil: water ratio), total organic C (the Walkley and Black method), total nitrogen (the Kjeldahl method), total P (the colorimetric method), total K (atomic absorption spectrometry), hydrolyzable N (alkali diffusion solution), extractable P (the Olsen’s method), and available K (with ammonium acetate) were determined following procedures described in Dai et al. [Citation24]. Main physical and chemical properties of the soil were as follows: pH 6.95 (soil water ratio of 1:2.5), organic matter 18.08 g kg−1, total N 0.21 g kg−1, total P 0.45 g kg−1, total K 2.68 g kg−1, alkaline hydrolysis N 29.17 mg kg−1, Olsen-P 7.71 mg kg−1, available K 86.06 mg kg−1 and total Se 0.288 mg kg−1.
Experimental design
A soil pot experiment was conducted in the greenhouse of the Micro-element Research Center in Huazhong Agricultural University in Wuhan, China (N 31°28’26”, E 114°20’15”). Two levels of Se (sodium selenite) treatment, i.e. 2.2 and 22.2 mg kg−1 Se, were performed in the experiment with 3 replicates. The size of the pots was 30 cm × 30 cm and each pot was filled with 10 kg of air-dried and 2 mm-sieved soil. The mixtures of 0.24 g kg−1 N, 0.36 g kg−1 P2O5, 0.6 g kg−1 K2O [in the form of CO(NH2)2, KH2PO4 and K2SO4, respectively] and 1 mL Arnon’s microelement solution for each kilogram soil were applied as basal dressing at 14 days before transplanting.
After sterilization with 2%(V/V)NaOCl for 10 min, the seeds of tobacco were thoroughly rinsed in tap water and then sown in floating nursery. Tobacco seedlings (at the four-leaf stage) were transplanted into pots, with one plant for each pot. Two months after transplanting, the leaves and roots of flue-cured tobacco were collected for the determination of Se speciation.
Plant Se speciation
The leaves and roots of flue-cured tobacco were thoroughly rinsed with Milli-Q water. After the water on the surface was dried up with gauze, the leaves and roots were firstly immersed in liquid N2 and then triturated with an agate mortar and pestle and freeze dried, finally stored at −80 °C until the extraction of Se species.
The Se species were extracted from 0.2 g ground leaves or roots by using 10 mL 1:2 methanol water and shaking for 15 min with ultrasonic wave at room temperature. The extracts were centrifuged at 5000 g for 30 min and the supernatant was collected in eggplant shaped flasks. The methanol in solution was evaporated by a rotary evaporation instrument (40 °C). Finally, the supernatant was filtered through 0.22 µm Millipore filters before the analysis by high performance liquid chromatography-ultraviolet treatment-hydride generation atomic fluorescence spectrometry (HPLC-UV-HG-AFS) [Citation25, Citation26].
The separation of SeIV, SeVI, SeMet and SeCys in the green parts of flue-cured tobacco was made by anion exchange chromatography in which the column was connected on-line to an ultraviolet treatment-hydride generation atomic fluorescence spectrometry (UV-HG-AFS) detection system [Citation18]. The HPLC system consisted of a SHIMADZU 10ATvp Plus liquid chromatography pump (SHIMADZU, Tokyo, Japan), a Rheodyne 7725i injector (Rheodyne, Cotati, USA) and a Hamilton PRP-X100 column (Hamilton, Reno, NV). The mobile phase for HPLC was 60 mmol L−1 (NH4)2HPO4 (pH 6.0, 1.0 mL min−1). For the HG part, reducing agents were 2.0% KBH4 (m/v) + 0.35% KOH (m/v) and the carrier solution was 10% HCl (v/v) and then passed through the UV unit. The detection part was AFS8220: Se hollow cathode lamp current (General Research Institute for Nonferrous Metals, Beijing, China) was 80 mA; the negative high voltage of photomultiplier tube was 300 V; the flow rate of the carrier gas was 400 mL min−1 and the flow rate of the makeup gas was 600 mL min−1 ().
Table 1. Optimized operational conditions for HG-AFS.
The standards solutions were prepared as follows: selenomethionine (SeMet) (Sigma, USA) and selenocysteine (SeCys) (Sigma, USA) were dissolved with Milli-Q water (Millipore, USA), and then 3% hydrochloric acid was added so that SeCys and SeMet were better dissolved. Selenite radical (GBW10032; 42.9 µg g−1 Se) and selenate radical (GBW10033; 41.5 µg g−1 Se) were provided by National Institute of Metrology P. R. China.
Statistical analysis
Data were represented as mean values with standard deviation (±SD). Statistical analysis was performed using sigmaplot 10.0. One-way analysis of variance (ANOVA) with multi-comparisons by Duncan’s test was employed to compare the means among different treatments at p < 0.05 in the SAS 8.1 statistical package.
Results
Optimized separation conditions
Selection of mobile phase
Only SeCys, SeMet and Se (IV) were found, whereas Se (VI) was not observed in the chromatograms obtained from the mobile phase containing 40 mmol L−1 NH4H2PO4 at pH 4.48 (). However, with a 40 mmol L−1 NH4Ac at pH 6.94 as the mobile phase, only Se (IV) was detected (). The chromatogram obtained from the mobile phase containing 40 mmol L−1 NH4NO3 at pH 5.18 showed that SeCys and Se (IV) were eluted, but SeMet and Se (VI) were retained in the column (). A mobile phase containing 40 mmol L−1 (NH4)2HPO4 at pH 6.00 was also used as shown in , where all the Se species were eluted, and a good resolution between Se species was achieved. Therefore, (NH4)2HPO4 as the mobile phase was researched further.
Figure 1. Typical chromatogram obtained when using eluent containing 40 mmol L−1 NH4H2PO4 pH 4.48 (A), 40 mmol L−1 NH4Ac at pH 6.94 (B), 40 mmol L−1 NH4NO3 at pH 5.18 (C) and 40 mmol L−1 (NH4)2HPO4 at pH 6.00 (D). The mixed standard concentration of SeCys 118.2 μg L−1, Se(IV)85.8 μg L−1, SeMet 88.7 μg L−1, Se(VI) 83.0 μg L−1 determined by HPLC-HG-UV-AFS. The optimized operational conditions for HG-AFS as described in . Note: HPLC, high-performance liquid chromatography; HG-AFS, hydride generation atomic fluorescence spectrometry.
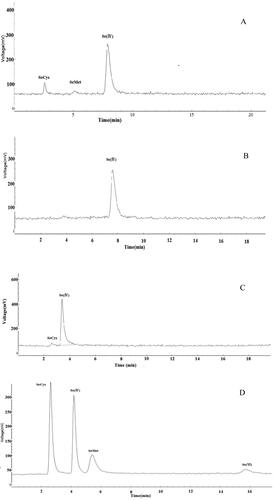
Selection of mobile phase concentration
With (NH4)2HPO4 as the mobile phase, the pH value was adjusted to 6.0. In this study, the separation of four selenium compounds was investigated within the mobile phase concentration range from 30 to 70 mmol L−1. The results showed that the mobile phase concentration had a great influence on the elution of inorganic selenium (). With the increase of the phosphate concentrations, the retention time of Se (VI) reduced significantly, from 21.9 min at 30 mmol L−1 (NH4)2HPO4 to 8.1 min at 70 mmol L−1 (NH4)2HPO4, while the retention times of SeCys, SeMet and Se (IV) slightly altered. However, when the concentration was 70 mmol L−1, the tailing of SeCys was observed and SeCys and Se (IV) could not be separated well. From the point of view of the four Se species separation, the mobile phase containing 60 mmol L−1 (NH4)2HPO4 was used in the subsequent steps of this study.
Selection of the eluent pH
The relationship between the mobile phase pH and the retentions of Se species using a mobile phase containing 60 mmol L−1 (NH4)2HPO4 is shown in . With increasing eluent pH, the retentions of SeCys and SeMet slightly increased. In contrast, the retention of Se (IV) slightly decreased, but the retention of Se (VI) decreased significantly. However, poor separation and peaks were observed at pH 6.5. Considering both the separation and the retention time, pH of 6.0 was optimal, where the four Se species could be well separated, and the peak shape was symmetric. Thus, 60 mmol L−1 (NH4)2HPO4 at pH 6.0 was used as an optimum mobile phase.
Calibration curves and recovery by the proposed method
Linear calibrations were obtained over a concentration range of 0–236.35 μg L−1 with correlation coefficients ranging from 0.9991 to 0.9996 when 100 μL was injected (). This was the basis for quantitative analysis of Se species, so the established method could be applied to Se speciation in plants.
Table 2. Standard curves of four Se species (n = 3).
In 1:2 methanol water extract conditions, the recoveries of SeCys, Se (IV), SeMet and Se (IV) in the Se-enriched samples were 95.72 ± 1.60%, 106.89 ± 2.20%, 93.28 ± 2.82% and 91.38 ± 1.27%, respectively. The results showed that Se speciation remained stable in the extraction analysis process. Therefore, the proposed method could be used for the determination of Se species in contaminated plants.
Analysis of Se species in the leaves and roots of flue-cured tobacco
The proposed method was used for the determination of Se species in Se-enriched flue-cured tobacco. Only SeCys, Se(IV) and Se(VI) were detected at low Se levels, while four Se species were all detected in the leaves and roots of flue-cured tobacco at high levels of Se treatment (). Furthermore, some unknown Se species appeared in the chromatogram of the flue-cured tobacco roots treated with 22.2 mg kg−1 Se ().
Figure 4. Typical chromatogram of the flue-cured tobacco roots treated with 22.2 mg kg−1 Se obtained when using an eluent containing 60 mmol L−1 (NH4)2HPO4 at pH 6.00. The optimized operational conditions for HG-AFS as described in . The retention times of the unknown species were 135 s (2’15") and 492 s (8’12") in . The unknown species were not identified due to the lack of Se standards in this method.
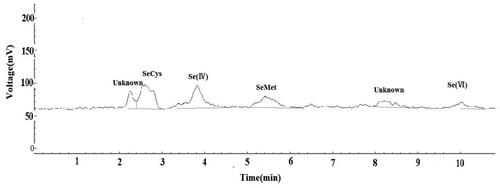
Table 3. Se species in the leaves and roots of flue-cured tobacco after applying the proposed extraction procedure and measurement with UG-AFS.
The result showed that the predominant Se species were Se (VI) (82.95–85.34%) in flue-cured tobacco leaves, followed by SeCys (4.74–14.66%), but Se (IV) (2.50%) and SeMet (9.80%) were only detected at high Se level treatment. However, the organic Se (SeCys and SeMet) were the main Se species in flue-cured tobacco roots, accounting for 46.44–60.21% of the sum of 4 Se species; and Se (IV) only accounted for 19.37–39.79%; but SeMet and Se(VI) were only detected at high Se level treatment.
Discussion
Ion-exchange chromatography is based on the exchange equilibrium between charged solute ions and the oppositely charged site on the stationary phase with ion exchange resin [Citation23, Citation27]. The retention of the target anion is determined by the nature of the competing ion and its concentration as well as the mobile phase pH [Citation28].
The working standard solutions of SeCys, SeMet, Se (IV) and Se (VI) were separated well using a Hamilton PRP X-100 with a mobile phase containing 40 mmol L−1 (NH4)2HPO4 at pH 6.00 as shown in . However, when the mobile phase was 40 mmol L−1 NH4H2PO4 at pH 4.48, only SeCys, SeMet and Se (IV) were detected (); and only SeCys and Se (IV) was separated using 40 mmol L−1 NH4Ac at pH 6.94 and 40 mmol L−1 NH4NO3 at pH 5.18 as the mobile phase as shown in and . The Se (VI) were not eluted because of their higher charge on the species under the eluent pH used, leading to their strong retention on the column [Citation29, Citation30]. On the other hand, competing anions such as Ac- and NO3- were too weak to elute SeMet and Se(VI) from the column [Citation23].
With (NH4)2HPO4 as the mobile phase and the pH value adjusted to 6.0, the retention times of inorganic Se [Se (IV) and Se (VI)] decreased, while the retention times of organic Se (SeCys and SeMet) changed smoothly with the increase of (NH4)2HPO4 concentration (). Similar results were reported by Mazej et al. [Citation31], where the best separation of SeCys, Se (IV), SeMet and Se (VI) were obtained using 40 mmol L−1 phosphate buffer (pH6.0) as mobile phase. However, the retention times of the Se species were prolonged due to a low concentration of phosphate. In contrast, the mobile phase containing a high concentration of phosphates led to overlapping of the peaks. Nevertheless, the Se(VI) retention time of 9.8 min in our study with the optimal mobile phase containing 60 mmol L−1 (NH4)2HPO4 (pH6.0 were far less than the retention time of 30–35min in the study of Mazej et al. [Citation31] using 40 mmol L−1 phosphate buffer (pH 6.0) and the retention time of 15 min in the study of Chen et al. [Citation31] using 40 mmol/L diammonium phosphate (pH 5.0) and 60 mmol/L diammonium phosphate (pH 6.0) as the mobile phase with gradient elution. In addition, the higher the phosphate in the mobile phase, the more effectively the eluent displaces selenium ions from the stationary phase and thus the more rapidly selenium ions are eluted from the column, so the shorter the retention times of Se species, especially Se(VI). These results were in accord with the findings of the previous study [Citation23, Citation32]. Consequently, considering both separation and retention time, the optimal concentration of (NH4)2HPO4 was 60 mmol L−1 as mobile phase, where four Se species were appropriately separated, and the peaks showed normal distribution, such as: the retention time of Se (VI) decreased to 9.8 min, greatly reducing the analytical time.
When 60 mmol L−1 (NH4)2HPO4 served as the mobile phase, the retention times of organic Se (SeCys and SeMet) slightly increased with the increase in pH, while the retention times of inorganic selenium [Se (IV) and Se (VI)] were reduced, and the retention time of Se (VI) was reduced greatly (). This was in agreement with the findings of previous studies [Citation31, Citation32], the retention time of Se (VI) decreased with the increase of pH using 40 mmol L−1 phosphate buffer as the mobile phase [Citation30], while only Se(IV) and Se(VI) were detected with pH up to 8, and the chromatographic peaks of SeCys2, MeSeCys and SeMet were not found with 40 mmol L−1 (NH4)2HPO4 as the mobile phase [Citation32]. In addition, pH also influenced the peaks of various Se species. Four Se species could be well separated, and good peak shape was obtained at pH 6.0.
The proposed method was used for the determination of Se species in the leaves and roots of Se-enriched flue-cured tobacco. It showed that the predominant Se species was Se(VI) in flue-cured tobacco leaves(). The result was in accord with the findings of the previous study on leek (Allium ampeloprasum) [Citation33] and rice [Citation34]. When 3.8 mg Se kg- 1 (sodium selenite) was added to soil, Se(VI) was the major Se species in leek [Citation33]. And when the soil Se addition exceeded 5 mg kg−1, inorganic Se was present in brown rice (Oryza sativa L.), mainly as Se(VI) [Citation34]. Compared to the Se form in leaves, the proportion of organic Se species (SeCys and SeMet) increased in the roots of flue-cured tobacco, while Se(VI) was only detected at 22.2 mg kg- 1 Se. The similar results showed that the major Se species were SeMet in Solanum tuberosum L. [Citation35], SeCys2 in Cardamine violifolia [Citation36] and SeCys2 and SeMet in Cordyceps militaris [Citation37]. In addition, some unidentified peaks were observed in the chromatograms of the roots of flue-cured tobacco (), and the unidentified peaks were also observed at 545 s in C. militaris [Citation37]. The unknown species were not identified due to the lack of Se standards in this method.
Conclusions
A mobile phase containing 60 mmol L−1 (NH4)2HPO4 at pH 6.0 is found to be the most suitable for the separation of Se species by the PRP-X100 anion exchange column coupled with AFS detection. The retention of the target anion is affected by the competing ion and its concentration as well as the mobile phase pH. Furthermore, the proposed method for the determination of Se species has been demonstrated in a number of plant tissues, and it shows that HPLC-UV-HG-AFS provides the advantage of fast separation, reasonable resolution and low detection limits at μg L−1 level, and the identification and quantification of the target ion.
Data availability
All data that support the findings reported in this study are available from the corresponding author upon reasonable request.
Disclosure statement
The authors confirm that they have no conflict of interest to report.
Funding
This study was supported by the National Natural Science Foundation of China, China (Grant No. 30870365); the Projects of Science and Technology Department, Henan Province, China (Grant No. 212102110066) and the Key Scientific Research Projects of colleges, Henan Province, China (Grant No. 20A210020).
References
- Thiry C, Ruttens A, Temmerman LD, et al. Current knowledge in species-related bioavailability of selenium in food. Food Chem. 2012; 130(4):767–784.
- Kuras R, Janasik B, Stanislawska M, et al. Revision of reciprocal action of mercury and selenium. Int J Occup Med Environ Health. 2018; 31(5):575–592.
- Zhu YG, Pilon-Smits EAH, Zhao FJ, et al. Selenium in higher plants: understanding mechanisms for biofortification and phytoremediation. Trends Plant Sci. 2009; 14(8):436–442.
- Zhou F, Yang WH, Wang MK, et al. Effects of selenium application on Se content and speciation in Lentinula edodes. Food Chem. 2018; 265:182–188.
- Sun M, Liu GJ, Wu QH. Speciation of organic and inorganic selenium in selenium-enriched rice by graphite furnace atomic absorption spectrometry after cloud point extraction. Food Chem. 2013; 141(1):66–71.
- Ali ZR, Mustafa T, Yar KM. Determination of selenium and arsenic ions in edible mushroom samples by novel chloride-oxalic acid deep eutectic solvent extraction using graphite furnace-atomic absorption spectrometry. J AOAC Int. 2018; 101(2):593–600.
- Carey AM, Scheckel KG, Lombi E, et al. Grain accumulation of selenium species in rice (Oryza sativa L.). Environ Sci Technol. 2012; 46(10):5557–5564.
- Valdez Barillas JR, Quinn CF, Freeman JL, et al. Selenium distribution and speciation in the hyperaccumulator astragalus bisulcatus and associated ecological partners. Plant Physiol. 2012; 159(4):1834–1844.
- Lin JR, Chen N, Feng RF, et al. Sequestration of selenite and selenate in gypsum (CaSO4·2H2O): Insights from the single-crystal electron paramagnetic resonance spectroscopy and synchrotron X-ray absorption spectroscopy study. Environ Sci Technol. 2020; 54(6):3169–3180.
- Li YX, Zhu NL, Liang XJ, et al. A comparative study on the accumulation, translocation and transformation of selenite, selenate, and SeNPs in a hydroponic-plant system. Ecotoxicol Environ Saf. 2020; 189:109955.
- Lenz M, Van Hullebusch ED, Farges F, et al. Combined speciation analysis by X-ray absorption near-edge structure spectroscopy, ion chromatography, and solid-phase microextraction gas chromatography-mass spectrometry to evaluate biotreatment of concentrated selenium wastewaters. Environ Sci Technol. 2011; 45(3):1067–1073.
- Gionfriddo E, Naccarato A, Sindona G, et al. A reliable solid phase microextraction-gas chromatography-triple quadrupole mass spectrometry method for the assay of selenomethionine and selenomethylselenocysteine in aqueous extracts: difference between selenized and not-enriched selenium potatoes . Anal Chim Acta. 2012; 747:58–66.
- Zhao YQ, Zheng JP, Yang MW, et al. Speciation analysis of selenium in rice samples by using capillary electrophoresis-inductively coupled plasma mass spectrometry. Talanta. 2011; 84(3):983–988.
- Liu LH, Yun ZJ, He B, et al. Efficient interface for online coupling of capillary electrophoresis with inductively coupled plasma-mass spectrometry and its application in simultaneous speciation analysis of arsenic and selenium. Anal Chem. 2014; 86(16):8167–8175.
- Sánchez-Martínez M, Silva EGPD, Pérez-Corona T, et al. Selenite biotransformation during brewing. Evaluation by HPLC-ICP-MSTalanta. 2012; 88:272–276.
- Gao HH, Chen MX, Hu XQ, et al. Separation of selenium species and their sensitive determination in rice samples by ion-pairing reversed-phase liquid chromatography with inductively coupled plasma tandem mass spectrometry. J Sep Sci. 2018; 41(2):432–439.
- Chan Q, Afton SE, Caruso JA. Selenium speciation profiles in selenite-enriched soybean (Glycine Max) by HPLC-ICPMS and ESI-ITMS. Metallomics. 2010; 2(2):147–153.
- Floor GH, Iglesías M, Román-Ross G, et al. Selenium speciation in acidic environmental samples: Application to acid rain-soil interaction at Mount Etna volcano. Chemosphere. 2011; 84(11):1664–1670.
- Lenz M, Floor GH, Winkel LHE, et al. Online Preconcentration-IC-ICP-MS for Selenium Quantification and Speciation at Ultratraces. Environ Sci Technol. 2012; 46(21):11988–11994.
- Da Silva EG, Verola Mataveli LR, Zezzi Arruda MA. Speciation analysis of selenium in plankton, Brazil nut and human urine samples by HPLC-ICP-MS. Talanta. 2013;110:53–57.
- Sanchez-Rodas D, Mellano F, Morales E, et al. A simplified method for inorganic selenium and selenoaminoacids speciation based on HPLC-TR-HG-AFS. Talanta. 2013; 106(106C):298–304.
- Xie XX, Feng CC, Ye MD, et al. Speciation determination of selenium in seafood by high-performance ion-exchange chromatography-hydride generation-atomic fluorescence spectrometry. Food Anal Methods. 2015; 8(7):1739–1745.
- Chen ZL, Akter KF, Rahman MM, et al. The separation of arsenic species in soils and plant tissues by anion-exchange chromatography with inductively coupled mass spectrometry using various mobile phases. Microchem J. 2008; 89(1):20–28.
- Dai XQ, Zhang HY, Spiertz JHJ, et al. Crop response of aerobic rice and winter wheat to nitrogen, phosphorus and potassium in a double cropping system. Nutr Cycl Agroecosyst. 2010; 86(3):301–315.
- Han D, Li XH, Xiong SL, et al. Selenium uptake, speciation and stressed response of Nicotiana tabacum L. Environ Exp Bot. 2013; 95(1):6–14.
- Han D, Xiong SL, Tu SX, et al. Interactive effects of selenium and arsenic on growth, antioxidant system, arsenic and selenium species of Nicotiana tabacum L. Environ Exp Bot. 2015; 117:12–19.
- Leblanc KL, Kawamoto MS, Le PM, et al. Quantitation of selenomethionine in multivitamins and selenium supplements by high performance liquid chromatography inductively-coupled plasma mass spectrometry. Food Anal Methods. 2019;12(6):1316–1326.
- Herath I, Kumarathilaka P, Bundschuh J, et al. A fast analytical protocol for simultaneous speciation of arsenic by Ultra-High Performance Liquid Chromatography (UHPLC) hyphenated to Inductively Coupled Plasma Mass Spectrometry (ICP-MS) as a modern advancement in liquid chromatography approaches. Talanta. 2020; 208:120457.
- Chen BB, He M, Mao XJ, et al. Ionic liquids improved reversed-phase HPLC on-line coupled with ICP-MS for selenium speciation. Talanta. 2011; 83(3):724–731.
- Nozohour YM, Yamini Y. Inorganic selenium speciation in water and biological samples by three phase hollow fiber-based liquid phase microextraction coupled with HPLC-UV. New J Chem. 2017; 41(6):2378–2385.
- Mazej D, Falnoga I, Veber M, et al. Determination of selenium species in plant leaves by HPLC-UV-HG-AFS. Talanta. 2006; 68(3):558–568.
- Chen SZ, Liu LP, Tang DJ. Determination of total and inorganic selenium in selenium-enriched rice, tea, and garlic by high-performance liquid chromatography-inductively coupled plasma mass spectrometry (HPLC-ICP-MS). Anal. Lett. 2020; 53:1–17.
- Lavu RVS, Du Laing G, Tom VDW, et al. Fertilizing soil with selenium fertilizers: impact on concentration, speciation, and bioaccessibility of selenium in leek (Allium ampeloprasum). J Agric Food Chem. 2012; 60(44):10930–10935.
- Dai ZH, Imtiaz M, Rizwan M, et al. Dynamics of selenium uptake, speciation, and antioxidant response in rice at different panicle initiation stages. Sci Total Environ. 2019; 691(15):827–834.
- Zhang HQ, Zhao ZQ, Zhang X, et al. Effects of foliar application of selenate and selenite at different growth stages on Selenium accumulation and speciation in potato (Solanum tuberosum L.). Food Chem. 2019; 286:550–556.
- Wu MR, Cong X, Li M, et al. Effects of different exogenous selenium on Se accumulation, nutrition quality, elements uptake, and antioxidant response in the hyperaccumulation plant Cardamine violifolia. Ecotox. Environ. Safe. 2020; 204:111045.
- Hu T, Liu LP, Chen SZ, et al. Determination of selenium species in Cordyceps militaris by high-performance liquid chromatography coupled to hydride generation atomic fluorescence spectrometry. Anal. Lett. 2018; 51(14):2316–2330.