Abstract
As the largest organ in the human body, the skin should be protected from various harmful chemicals and pollutants from the surrounding environment and ultraviolet (UVA/UVB) radiation emitted from sunlight. This has prompted formulation scientists to embark on new technology to formulate cosmetics free of synthetic chemicals using natural plant-based extracts as the replacement. Natural phytoanti-oxidants, viz. catechin, quercetin and gallic acid, are polyphenols whose therapeutic values (antioxidative, antityrosinase, antiviral and antimicrobial) are underutilized. Plant-based nanoemulsions offer an efficient and safe topical delivery system for improving the skin and regenerative treatment. The bioavailability of the phytoanti-oxidants for molecular-level skin repair is enhanced by better permeation of the nanoemulsions’ nano-sized particles through the stratum corneum. This review highlights several phytoconstituent-containing nanoemulsions and their bioactivities for cosmetic applications. The mechanisms of skin improvement for anti-aging skin are also presented in detail. In short, nanoemulsion technology is a powerful tool for an effective topical delivery system of potent skin-protecting and rejuvenating plant-based extracts. With increasing demand from consumers worldwide for nano-formulated phytohormones or phytoextractives, nanoemulsions will see a new dimension with better future prospects.
Introduction
The skin is the largest organ of the human body whose proper function is to protect against external factors, for instance, invading microorganisms, temperature, ultra-violet (UV) rays, and preventing our body from water and electrolyte loss. This organ contributes to approximately 16% of the total human body weight, which is the equivalent of 9 kg and covers a surface area of 1.8 m2 [Citation1–3]. The outermost layer of the skin structure is the stratum corneum, a crucial and formidable barrier to be overcome by any topical or transdermal applications due to the complicated physiology of the human skin. The densely packed lipid mortar and protein bricks in the stratum corneum acts as the primary barrier limiting the permeability of active ingredients or drugs [Citation4]. The human skin undergoes several changes when exposed to the sun, including the formation of wrinkles, alongside the physiological and appearance of skin layers associated with skin aging [Citation5,Citation6]. Therefore, any damage to the skin barrier function is manifested by a change in the stratum corneum integrity followed by an increase in transepidermal water loss and decreased skin hydration [Citation7,Citation8]. The stratum corneum of the human skin is illustrated in .
The human skin has three layers, viz. epidermis, dermis and hypodermis (), with each layer differing in 1.5 mm thickness. The epidermis comprises the stratum corneum, stratum lucidum, stratum granulosum, stratum spinosum and stratum basale. Keratinocytes and melanocytes are largely produced in this outer layer, responsible for protecting skin against UV rays, microorganisms, air and chemical pollutants. While collagens, elastic fibers, sweat glands, hair follicles, connective tissues, touch receptors, blood capillaries exist in the dermis. The hypodermis is the last layer of skin structure, primarily constructed of areolar and adipose tissues and large blood vessels [Citation9,Citation10]. Noteworthily, skin pigmentation/hyperpigmentation is one of the aging processes occurring at the epidermis layer caused by UV-induced radiation. Excessive melanin is produced by melanocytes in melanosomes during melanogenesis in response to UV exposure. The melanosomes are then transported to the keratinocytes around the melanocytes. Likewise, elastin and collagen degradation in the dermis cause wrinkles and skin elasticity loss [Citation11]. Hence, sun protection, skin repair treatment, and rejuvenation are carried out at the skin’s epidermis layer to avoid this circumstance [Citation12].
There are treatments by which the human skin can be regenerated, and the current trend in cosmeceutical products has seen nano-sized emulsions playing a larger role in this industry. Nanotechnology is a science that manipulates and reduces materials to the nanometer scale (1–100 nm), bringing about new properties that can regulate the different aspects that control the diffusion of ingredients through the skin [Citation13]. The colloidal dispersion system in a nanoemulsion consists of oil, water, and surfactant. The >500 nm mean droplet size of the particles can augment the dermal and transdermal effectiveness of the incorporated bioactive ingredients [Citation14,Citation15]. Two categories of nanoemulsions exist: the ‘water-based’ (O/W) and the oil-based (W/O). The former has water as the continuous phase and oil as the dispersed phase, whereas the phases are inversed in the latter’s colloidal dispersion system [Citation16]. represents the basic structures of a) water-in-oil (W/O) and b) oil-in-water (O/W) nanoemulsions, comprising of oil, water and surfactant as the major compositions. The water-in-oil depicts the formation of nano-sized water droplets in which the hydrophilic head of the surfactant orientates towards the water, and the hydrophobic tail faces the oil. Contrariwise, the o/w system arranges the hydrophobic tail towards the oil droplets and the hydrophilic head to water [Citation17]. More complex dispersion systems include double nanoemulsion systems (oil-water-oil or water-oil-water) [Citation18]. The above-said colloidal systems exist by emulsification with a specific hydrophile-lipophile balance (HLB) value. In this respect, surfactants are prudently selected to avert physical instability in these nano-systems. Surfactants and sometimes co-surfactants used during the nano emulsification step are mostly non-ionic. The rule of thumb, surfactants of a final HLB value between 4–6 are ideal for preparing W/O nanoemulsions, whereas a higher HLB value (8-16) is suitable for oil-in-water systems.
Colloidal nano-formulations can be prepared via low energy and high energy methods where the latter requires significant energy input to break the larger particles into nano-sized droplets [Citation20]. Examples of the high energy methods viz. ultrasonication, microfluidization, and high-pressure homogenization depend on mechanical devices such as ultrasonicators, microfluidizer, and high-pressure homogenizers for disruptive forces to obtain the smallest particles size in the system. High energy is also required to initiate the stress level to exceed the Laplace pressure with pressures between 10–100 atm to transform microemulsions to nanoemulsions. This outcome is achieved by rupturing the droplets [Citation21]. Conversely, low energy nano-emulsification methods through spontaneous emulsification, phase inversion composition and phase inversion do not involve mechanical forces to break down the droplets [Citation22]. Pertinently, transforming the microemulsion system to nano ones greatly improves the bioavailability of active ingredients to the targeted cells and facilitates their diffusion through the skin [Citation23,Citation24]. This is due to the uniform deposition of the active ingredients and their better permeability or penetration through the skin surface [Citation25]. Nanoemulsions are also described to be exceptionally stable against colloidal destabilizing factors, for instance, sedimentation, flocculation, coalescence or creaming [Citation26,Citation27].
Plant extracts from seeds, flowers, leaves, roots, and fruits were popular beauty ingredients in China and Egypt during ancient times [Citation28]. The use of plant-based bioactive compounds in topical skincare products is considered far more efficient, safe and cost-effective than synthetic ones [Citation29]. Plants are rich in valuable secondary metabolites, namely, phenolic acids, flavonoids, polyphenols, terpenoids and amino acids, all of which are well-known UV blockers or UV filters skincare products [Citation30]. Conversely, synthetic chemicals, such as preservatives and fragrances, may be detrimental to human health, possibly triggering severe side effects, including cancer, mutation, reproductive toxicity and endocrine disruption [Citation31]. Plant-based bioactive compounds’ bioavailability to target areas can be boosted by topical application assisted by nanotechnology-based carriers or simply called nano-carriers. The minute droplet topical delivery systems (TDS) encapsulate the active plant ingredients with low water or lipid solubility. The system also simultaneously protects the components from physical and chemical degradation, enable their controlled and sustained release at the target site.
Moreover, product skin spreadability is also improved by nano-carriers, making the cosmetic formulations more enjoyable, thus enhancing public adherence [Citation32]. The TDS circumvent issues with the product’s administration, including drastic pH changes, deleterious presence of food and enzymes, and the liver’s first-pass effect. TDS is also non-invasive and suitable for needle phobes and can be self-administered with minimal side effects. However, TDS is less apt for delivering large hydrophilic molecules due to the very low skin permeation rates of the system. The continuous stratum corneum (the outer layer of skin) poses a significant challenge for the active ingredients to penetrate deeper into the skin layers [Citation33].
Phytobased cosmeceuticals are set to play a highly significant role in the near future in enhancing protection and skin beauty, as well as other health benefits. The safety of over-the-counter cosmeceuticals is often dubious since most are without any commercial approval. The increasing awareness among consumers has seen a higher preference for plant-based cosmeceuticals since the ingredients are generally accepted as safe. In this regard, this review focuses on several plant phytochemicals and their bioactivities for topical applications. The selection of plant-based bioactive compounds in nanoemulsions used in clinical skin improvement studies, and their mechanisms of action for skin repair, namely for melanogenesis, collagenesis and anti-aging through the anti-oxidant process, are also discussed.
Plant-based bioactive compounds in cosmeceuticals for skin therapies
Cosmeceuticals are a niche of cosmetic products in which active ingredients impart their cosmetic benefits, evidence therapeutic effects capable of changing skin function and structure. Cosmeceuticals are basically functionalized cosmetics with beauty and health applications [Citation32,Citation34]. On the other hand, cosmetics are products used to care for and clean the human skin, to make it more attractive and beautiful, and promote well-being without affecting body organs and parts or functions [Citation31,Citation35]. Plant-based bioactive compounds are highly sought-after active ingredients in the cosmeceuticals industry due to their therapeutic effects, such as moisturizing, rejuvenating, anti-aging, UV protection and prevention of skin-related diseases. Natural bioactive plant compounds are preferable for being eco-friendly and compatible with different skin types, without the artificial aspects of synthetic chemical ingredients that often cause skin irritation [Citation32,Citation36]. Several types of nano-carrier systems such as nanoemulsion, liposomes, solid lipid nanoparticles, hydrogels, dendrimers and smaller-sized nanoparticles have been developed to deliver plant-based bioactive compounds in cosmetics products [Citation10,Citation37]. Some of the plant-based bioactive compounds and their association with bioactivities for skin repair or improvement are listed in . illustrates the structures of several popular plant-based bioactive compounds.
Figure 3. Structures of plant-based bioactive compounds (a) catechin, (b) quercetin, (c) gallic acid (d) kaempferol, (e) gallocatechin, (f) epicatechin, (g) epigallocatechin, (h) catechin gallate, (i) gallocatechin gallate, (j) epicatechin gallate, (k) epigallocatechin gallate, (l) curcumin, (m) resveratrol and (n) lycopene
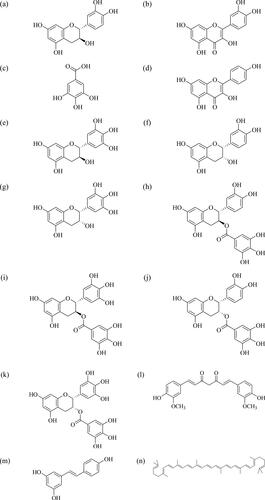
Table 1. Bioactive compounds, plant origin, particle size, PDI and bioactivities in nanoemulsions.
Quercetin
Quercetin (C15H10O7) is a well-known plant pigment and polyphenolic flavonoid found in leafy vegetables, citrus fruits, berries, rich in anti-oxidants [Citation48]. Chemically, quercetin features a catechol B-ring and three additional hydroxyls on carbons 3, 5 and 7. Previous studies had demonstrated that the anti-oxidative property of quercetin is enhanced by higher concentrations of hydroxyl groups in conjunction with the adjacent hydroxyl groups in a benzene ring-C, which forms a resonance stabilized quinone structure. It is the third most active flavonoid after epicatechin gallate and epigallocatechin gallate [Citation49]. Generally, quercetin exhibits anti-inflammatory action by reducing edema, leukocyte formation and irritation and helps tissue regeneration by promoting new collagen fibers and producing ground substances for skin restoration [Citation50,Citation51]. Also, quercetin inhibits various inflammatory mediators such as interleukins (IL), prostaglandins (PGs), produced by COX, LOX, LPS. Quercetin is responsible for reducing the expression of matrix metalloproteinase-1, which is vital for skin wrinkling and loss of elasticity in healthy and photoaged skin, at both the mRNA and protein levels [Citation52]. Basically, quercetin partakes in inhibiting all the mediators, which leads to oxidative stress, thus producing the anti-oxidant effect [Citation53] and inhibits the secretion of the mast cell, which contributes to the anti-allergic effect of the compound [Citation54]
Despite this, quercetin has poor water solubility and low skin permeability, which somewhat hinder its use in cosmetics products. Notwithstanding the low bioavailability of quercetin, it produces several therapeutic benefits and constitutes an essential intermediary of its bio-actives [Citation48]. The issue can be overcome by formulating quercetin in nano-carriers, i.e. nanoemulsions, to improve bioavailability. Fasolo et al. [Citation42] formulated an O/W topical nanoemulsion containing quercetin extracted from Achyrocline satureioides Lam D.C. The low solubility of lipophilic quercetin was circumvented through an O/W nanoemulsion, as confirmed by the higher quercetin recovery. The spontaneously emulsified quercetin nanoformulation demonstrated excellent antiviral activity, reduced the infectivity of herpes simplex virus type-1 and the intracellular virus replication. The nanoemulsion showed sizes lower than 200 nm, with flavonoid contents recovery at 0.991 ± 0.006 at the skin extracts [Citation38,Citation42] performed a similar experiment for topical nanoemulsions containing flavonoids, namely quercetin, luteolin, and 3-O-methylquercetin extracted via maceration of the A. satureioides extract. The nanoemulsion was formulated by spontaneous emulsification in which medium chain triglycerides (7.5% w/w), egg-lecithin (2.0% w/w), A. satureioides extract (1.0% w/w), vitamin E (0.5% w/w), polysorbate 80 (1.0% w/w) and finally made up with water to a total of 100% w/w. Higher amounts of the flavonoids through the upper and deeper layers of porcine esophageal mucosa indicated their higher permeability through the tissue [Citation38]. Karadag et al. [Citation55] optimized the preparation of coarse stable nanoemulsions of quercetin, which contained limonene oil, a mixture of Tween 80 and Span 20 (1:1 weight ratio) as the emulsifier, and a water phase using high-speed homogenization. The optimum formulation required a 17% oil content, 13% mixed emulsifiers, and a homogenization pressure of 70 MPa [Citation56]. produced a stable topical quercetin nanoemulsion by chemometrics approach, using a lower energy method of spontaneous emulsification using simple ingredients of lecithin, water, and Tween 80.
Catechin
Catechins are a group of flavan-3-ols from the flavonoid chemical family. The name is derived from ‘catechu’ from the extract of Acacia catechu L., which is a 3,3′,4′,5,7-pentahydroxyflavan having two steric forms of (+)-catechin and (−)-catechin. Catechins and the analogs endowed galloyl moiety such as propyl gallate have significantly demonstrated exceptionally high inhibition capacity of free radicals. Researchers have identified and confirmed that the galloyl moiety of green tea catechins is the critical structural feature for any given bioactivities. Catechins are commonplace compounds in an array of herbs and foods, including apples, cacaos, tea, grapes, berries and persimmons [Citation57]. However, the Camellia sinensis (C. sinensis) and C. assumica are said to have the highest catechin content. Catechins possess many benefits that include skin damage prevention or reduction and intensive anti-oxidant and representative physiological activities [Citation39]. Catechins function indirectly as anti-oxidants through their effects on transcription factors and enzyme activities. As a matter of fact, the compounds strongly scavenge free radicals and retard extracellular matrix degradation induced by ultraviolet (UV) radiation and pollution. The swift and extensive metabolism of catechins by the human body further underscores the importance of their in vivo anti-oxidant activity [Citation58,Citation59]. The emulsification of catechins also improves their permeation through the skin, alongside better protective capability against UV rays and anti-aging effects [Citation60].
Ipso facto, catechins have since found applications in the cosmeceutical industry since the compounds can augment the penetration and absorption of bio cosmetics into the skin, thus improving their utility [Citation61]. Studies have also shown that catechins positively affect the skin by activating collagen synthesis and interfere with the matrix metalloproteinase enzyme production [Citation59]. The highly effective free-radical scavenging activity of catechin derivatives, the (-)-epigallocatechin and (-)-epicatechingallate than the standard anti-oxidants, viz. ascorbic acid, tocopherol and Trolox, has to do with the presence of the hydroxyl in the gallates [Citation61–63].
To date, catechins from the extract of Phyllanthus emblica L. branch extract have been formulated into a topical nanoemulsion comprising of isopropyl myristate (0.6% w/w), Brij 78 (0.35% w/w), P. emblica extract (0.15% w/w), and water (98.9% w/w). The catechin-rich nanoemulsion containing epicatechin gallate, epigallocatechin, epigallocatechin gallate and gallocatechin in P. emblica showed excellent anti-oxidant properties, alongside astringent, antimelanogenesis and anti-inflammatory MMP-I properties, thus implying its potential use as an anti-aging product. Likewise, the minute droplet sized (< 200 nm) of the O/W nanoemulsion indicated better penetration through the stratum corneum, as well as excellent storage stability for up to 90 days [Citation39].
Catechins are excellent bioactive ingredients in topical nanoemulsions due to their high solubility in W/O and O/W systems. Several studies have consistently shown that catechin-rich nanoemulsions could permeate across the stratum corneum and enter the deeper skin layers, including the viable epidermis and dermis [Citation61]. Another study experimented on catechins obtained from the leaves extract of Eugenia dysenterica DC as the bioactive ingredient for dermatological and cosmetics applications. The resultant topical nanoemulsion achieved the smallest droplet size of 27.0 ± 1.02 nm and 191.0 ± 7.20 nm for the W/O and O/W microemulsions, respectively, with long-term storage stability as long as three months. Further, in vivo studies revealed that catechins from the Eugenia dysenterica DC leave extract could reach the viable epidermis and dermis layers of the skin [Citation43].
Gallic acid
Gallic acid (GA) is a phenolic compound known as 3,4,5-trihydroxy benzoic acid with the molecular formula of C6H2(OH)3CO2H. The GA molecule has two types of functional groups, hydroxyl groups and a carboxylic acid group, which can interact to yield different salts and esters, including digallic acids [Citation64]. The unique coplanar and bent configuration of the ortho-bonded hydroxyl groups were shown to be responsible for the favorable antioxidative activities seen in GA [Citation65]. GA is widely distributed in the plant kingdom as a large family of secondary plant metabolites and natural anti-oxidants. The compound exists in both a free state and a component of tannins, such as the gallotannin, and GA derivatives. The compounds are found in almost every part of the plant, for instance, the bark, leaf, fruit, wood, seed and root. In particular, processed beverages such as red wine and green tea are exceptionally rich in GA. This naturally occurring phenolic compound forms white, yellowish-white, or pale fawn-colored organic acid crystals with good solubility in alcohol and ether but poorly soluble in water [Citation66,Citation67]. Aside from the potent anti-oxidant property, GA and its derivatives are also recognized for their antimicrobial, anti-inflammatory, and even antidepressant properties [Citation27,Citation68].
A dimeric derivative of GA, which is ellagic acid, found mostly in fruits and berries, i.e. raspberries, strawberries, blackberries and pomegranates, has been explored as an active ingredient in topical nanoformulations [Citation41,Citation69]. The pomegranate, specifically known scientifically as Punica grantum L., is a fruit-bearing deciduous shrub in the family Lythraceae. High anti-oxidant properties in the fruit peels have been reported because of the inherently high GA content. Extract of pomegranate peels strongly inhibited free radicals formed by harmful UV radiation and, was shown to protect skin from UV-induced erythema, burns, DNA fragmentation and depigmentation [Citation70]. The in-vitro skin permeation study of the P. grantum peel extract O/W nanoemulsion found that the UV radiation was well absorbed by the spherical-shaped GA droplets (a combination of allic acid, ellagic acid and punicalagin) before the harmful rays reached the viable skin layers. This confirmed the compounds’ ability to quench free radicals produced by UV radiation [Citation41]. Another study reported that polyphenols’ low surface-active ability, such as GAs could beneficially reduce the surface tension at the O/W interface. This indicated the plant-based active ingredient’s suitability to be formulated as either O/W or W/O nanoemulsions [Citation71]. It is reported that phenolic acids in the crude extract of tamarind (Tamarindus indica L.) fruit pulps possessed potential skin lightening, antityrosinase, anti-oxidant properties, and are promising anti-aging and skin whitening nanoemulsions [Citation40]. Both studies consistently proved that the GA-rich pomegranate peel extract nanoemulsion is a promising topical anti-oxidant delivery system for the skin.
Kaempferol
A member of flavonols, kaempferol or 3,40,5,7-tetrahydroxyflavone (C15H10O6), is a natural plentifully flavonoid found in apples, beans, broccoli, tea and strawberries. This compound’s pure form is a yellow colored powder, known for having a multitude of pharmacological properties, for instance, anticancer, anti-tumor growth, anti-oxidant, anti-inflammatory and anti-allergic activity, as reported in numerous research publications [Citation72]. Kaempferol can augment the body’s anti-oxidant defense against free radicals as it is a powerful anti-oxidant capable of averting oxidative damage to our cells, lipids and DNA. Conversely, the substance seemingly conserves normal cell viability, in some cases, exhibits a protective effect. The number of hydroxyl groups in this compound and their disposition increased the superoxide and free radical scavenging activity. Also, the substitution of the hydroxyl groups by methoxyl groups reduced their scavenging activity. Kaempferol and quercetin are thought to act synergistically in delaying cancer cell proliferation, where a combined therapy of quercetin and kaempferol is more potent than the effects of each flavonoid alone [Citation73,Citation74]. Studies have consistently shown that kaempferol reduces the resistance of human cancer cells lines to anticancer drugs [Citation73], induces apoptosis in human glioblastoma cells, and is better absorbed than quercetin in humans, even at low doses [Citation75]
Kaempferol is naturally lipophilic like other flavonoids and is absorbed by passive diffusion, facilitated diffusion, and active transport due to its lipophilicity. Consequently, the variety of nano-carrier systems can better improve kaempferol’s bioavailability for topical or cosmeceutical applications [Citation32,Citation76]. Novel topical delivery systems are continually being developed to enable the controlled-release of the kaempferol at a predetermined rate and prevent interaction with the gastric and intestinal fluids that can unwantedly degrade the substance [Citation51]. Given this, a wide range of nano delivery systems, i.e. kaempferol-containing nanoemulsions, has been prepared [Citation51]. Hernández-Jaimes et al. [Citation44] formulated a kaempferol-loaded nanoemulsion from Equisetum arvense extract, oil, water, using pseudo-ternary phase diagram, in which Tween 20 and ethanol were used as surfactant and co-surfactant, respectively. The droplets in the nanoemulsion were between 54.0 to 110 nm and fitted well with a first-order kinetics model [Citation44]. Kaempferol acts as a novel agent in treating UVB-induced tumorigenesis and photo-inflammation. Kaempferol was studied in skin cancer, where the COX 2 enzymes’ level was elevated. It inhibits the AP-1 (Activator protein) activity by suppressing COX-2 in JB6 P + mouse epidermal cells [Citation77]. In another study by Yun Chao et al., a pseudo ternary phase diagram helped prepare submicron emulsion (kaempferol 0.1%) using ethanol, and PEG 400 co-surfactant led to droplet sizes between 75.3 and 85.4 nm. The zero-order kinetics in vitro permeation study on rat skin showed the formulation increased kaempferol deposition with a reduced lag time [Citation78].
Curcumin
Curcumin is a polyphenolic compound from the Curcuma longa (turmeric) rhizomes. This root vegetable has been used widely to treat various skin diseases, for example, acne, alopecia, melanoma, psoriasis, and for wound healing. Curcumin has a wide range of biological and pharmacological activities, such as anticancer, anti-oxidant, antimicrobial, anti-inflammatory, and so forth [Citation45,Citation79,Citation80]. Interestingly, information on the structure–activity relationship of curcumin divulged its important role in various bioactivities that the compound displays. The o-diphenoxyl and o-dimethoxyphenoxyl groups in the curcumin structure exhibited higher DPPH scavenging due to the increased presence of electron-donating groups (methoxy and hydroxyl) in the o-position of 4-OH. Curcumin, however, is poorly soluble in an aqueous medium following its lipophilic nature. Recent studies attempted to package curcumin into nanoemulsion systems to resolve this significant limitation and improve bioactivity through increased bioavailability. This is done to enhance the absorption or permeability of curcumin into the stratum corneum for local and systemic delivery, as well as for topical bioavailability [Citation45,Citation81].
The encapsulation of curcumin has been described to enhance its delivery and increase the anti-oxidant activity, in particular, for nanoformulations that have been specifically designed to cease the aging process in the stratum corneum [Citation82]. In addition, curcumin was shown to significantly expedite the wound healing process in the stratum corneum, boost granulation in the tissues, and promote more effective cellular content and neo-vascularization and re-epithelialization of the wound [Citation46].
Resveratrol
Resveratrol, 3,5,4′-trihydroxytrans-stilbene (C14H12O3), is a member of stilbenoids, abundantly found in grapes, blueberries, peanuts, cranberries, soybeans and pistachios. Resveratrol analogs can be further divided into three different groups: catechol, resorcinol and pyragallol depending on the hydroxyls’ positions. However, resveratrol and its hydroxylated analogs at ortho position are shown to exhibit higher anti-oxidant activity. This compound also possesses several interesting pharmacological properties: anti-tyrosinase, anticancer, anti-oxidant and anti-inflammatory ones [Citation83].
In comparison to other anti-oxidants, resveratrol is an excellent skin protector against UV-B radiation. The compounds are capable of inhibiting the activities of certain kinases and tyrosinases and induce cancer cell apoptosis and senescence [Citation83]. Nevertheless, the downside to resveratrol’s clinical use is its poor water solubility (less than 1%), which reduces its bioavailability to target skin sites [Citation84]. Studies attempted to overcome this limitation by formulating a resveratrol-loaded nanoemulsion with 66–68 nm particle sizes, successfully boosting its bioavailability for protection against ultraviolet radiation [Citation36]. Recent in-vitro and in-vivo studies explored the potential of a nano-formulated resveratrol gel to prevent oxidative skin damage, which afforded a novel delivery system with enhanced permeability, free radical scavenging and retention effects [Citation85].
Lycopene
This compound is a form of natural pigment that hails from the carotenoids group and is exceptionally abundant in tomato seeds [Citation86]. Lycopene is widely used in skin care products because of its capacity to nullify harmful oxidants by scavenging and neutralizing free radicals’ negative effects. However, biolycopene suffers the same limitation as resveratrol as it is strongly lipophilic (log p∼15). This feature complicated lycopene absorption from the stratum corneum to other inner layers, mainly because of the compound’s affinity for the stratum corneum components and tended to be retained in this layer [Citation47]. The solution to this drawback is nano-encapsulation to enhance lycopene’s bioavailability through the skin [Citation87]. It is important to note that all trans-lycopenes have a linear chain and were 1.4 and 2.7 fold more efficient in quenching the reactive oxygen species (ROO•) than the cis isomers.
According to the literature, the prolonged diet of consuming tomatoes may prove useful in the repair of UV-induced sun-burned skin [Citation88]. In 2013, Ascenso et al. created a lycopene-rich nano-sized topical formulation that better protected the skin from UV-induced damage, as a result of the phytoconstituents’ improved bioavailability to the cutaneous level of the skin. Lycopene is also proven to have corrective roles in photo-damaged and preneoplastic keratinocytes, supporting the amenability of nano-formulated lycopene over conventional cosmetic products [Citation47,Citation89].
Cosmeceutically relevant crude plant extracts
The highly diverse plant kingdom is a reservoir for cosmeceutically exceptional crude extracts, packed with compounds that could be transformed into nanoformulations for delaying physiological aging. The wide range of phytoconstituents in plant crude extracts would render an array of synergically-significant bioactive effects compared to single bioactive compounds. Furthermore, naturally-occurring anti-oxidants from the crude extract of plants offer potential function in skin therapy against skin aging by benefiting from the complex composition of flavonoids, alkaloids, tannins, stilbenes, lignins, etc [Citation90].
For instance, the commonly found Prunus dulcis Mill from the Mediterranean and Middle East countries could be valuable as the active ingredient in nanocosmetics for skin repair [Citation90]. Its oleic- and linoleic acid-rich compositions were found comparable to almonds (80–90%) [Citation91]; hence, it is naturally endowed with exceptional anti-oxidant capacity. This is related to the myriad of polyphenols (flavonoids, phenolic acids) in the crude extracts from various parts of the plant [Citation92]. Another interesting plant extract from the Murraya koenigii belonging to Rutaceae family, commonly known as ‘curry leaves’ was high in free radicals-quenching compounds such as alkaloids, flavonoids, terpenoids and polyphenols. All the above-said compounds were found in leaves, roots, stem bark, fruits and seeds, indicating the plant is an excellent source of natural anti-oxidants for anti-aging formulations [Citation93].
Actions of plant-based bioactive compounds in cosmeceuticals
Advancements in nanoformulation technology attract many scientists who are increasingly in favor of nano-cosmeceuticals to prepare more effective moisturizers, anti-wrinkle, anti-acne, or even whitening creams to treat problematic skin with greater efficiency. Nanotechnology, particularly nanoemulsions loaded with naturally occurring bioactive compounds in the form of plant extracts, has been effective in boosting skin hydration or oiliness and reducing wrinkles’ formation. Efficiencies of such formulations have been reported to supersede commercial synthetic cosmetics products [Citation94]. For instance, moisturizers are applied to the skins’ surface to help with moisture retention in the stratum corneum surface and keep human skin hydrated over a longer duration. W/O moisturizers are formulated to go well with dry skin or skin-types that would benefit from the added oiliness on the stratum corneum and protect the human skin from extreme moisture loss. The applications or intended end-uses of anti-aging nanoemulsions are broad, and the following sub-sections further discuss their mechanism of action. The plant-based nano-sized formulations and their respective end-uses are presented in .
Table 2. Bioactive compounds, plant origin, delivery method, particles size, PDI and end use of nanoemulsions.
Moisturizer
The moisturizer is vital in cosmetics as it forms a thin protective film on the stratum corneum when applied to the skin surface and prevents dehydration of the stratum corneum. As a matter of fact, moisturizers are standard in cosmetic products to support skin beauty and enhance skin flexibility, as well-hydrated skin is a sign of healthy, young and uncompromised skin. Various skin problems such as dry skin disorders, psoriasis, atopic skin, ichthyosis, and contact dermatitis can benefit from a moisturizer’s consistent applications [Citation109]. The incorporation of moisturizers in nanoemulsions can boost skin hydration by two different pathways: a) by forming an occlusive thin film on the stratum corneum where the rate of water evaporation or dryness from the skin is controlled and, b) by transporting hygroscopic substances capable of binding and retaining water into the stratum corneum, for instance, glycerin [Citation62].
The effect of kaempherol, quercetin, galacturonic acid, glucose, rhamnose and arabinose in topical nanoemulsions of the Opuntia ficus-indica (L.) extract was investigated [Citation110]. They found that when galacturonic acid, glucose, rhamnose and arabinose are associated with a nanoemulsion, the compounds function as a humectant and proceed through a nonocclusion mechanism to hydrate the skin [Citation110]. Miller [Citation111] investigated as potential anti-aging and moisturizing agent on the stratum corneum [Citation111]. The hydrophilic O/W nanoemulsion of the 1% plant extract developed by [Citation8] successfully increased the moisture content of the stratum corneum on the forearm for 5 h after application, thereby proving its moisturizing efficacy. The formulation was also stable for at least 60 days [Citation8]. Vitamin E and fatty acids were found abundantly in Clinacanthus nutans (L.) leaf extract, which showed moisturizing efficacy. The O/W nanoemulsion reduced the water loss and improved the hydration level in the stratum corneum [Citation95,Citation96].
UV protection
With an increasing number of reported skin cancer cases, sunscreen products have become more critical than ever to protect the skin against ultraviolet (UV). Natural plant secondary metabolites, viz, phenolic acids, flavonoids, polyphenols, terpenoids and amino acid, alkaloids and tocopherols, are increasingly being used as UV blockers in sunscreen formulations due to their remarkable anti-oxidant properties [Citation112]. The compounds prevent harmful UV radiation from penetrating healthy skin and halt the inflammation process, oxidative stress and DNA damaging effects [Citation30]. Thus, topical nanoemulsion containing the aforementioned photo-protective compounds can better penetrate the skin’s stratum corneum compared to microemulsions [Citation113].
Drug delivery abilities and penetration enhancement by various lipid-based colloidal carriers to treat dermatological disorders such as photoaging and skin inflammation were investigated for retinoids, including vitamin A, retinyl palmitate and other derivatives. The studies found that fatty acid esters were intrinsically more stable and showed reduced irritant effects and immunogenic responses [Citation110,Citation114]. Nanoemulsions of retinyl palmitate gave the highest permeation and reached deeper skin layers [Citation115]. Nanoemulsions of the retinoids exhibited an increased tretinoin-induced anti-inflammatory response in ex vivo skin over other tested retinoids. The nano emulsified retinol microcapsules also induced other biological processes that shielded the skin against extracellular matrix lipid metabolism reactions, oxidative stress and cell proliferation when tested against a leading commercial product containing microencapsulated retinol or tretinoin [Citation110,Citation114,Citation116].
In 2016 [Citation117,] proved that the quercetin-rich ethanolic extract of Achyrocline satureioides showed adequate anti-oxidant capacity after it was formulated into a nanoemulsion. The minimal dose of the nano emulsified A. satureioides extract exhibited a higher anti-oxidant effect over thiobarbituric acid. This indicated the possible applicability of the nano formulated A. satureioides extract as a photo-protective product [Citation117]. Other bioactive compounds, namely, isoflavones in soybeans, aglyconeforms daidzein, glycitein, genistein and glucosidesdaidzin glycitin, genistin as well as malonylglucosides showed remarkable antioxidative properties, too. In 2018, a study by [Citation100] proved that isoflavone-rich soybean extract nanoemulsions were excellent photo-protective formulation to shield the human skin against oxidative damage caused by UVA/UVB [Citation100].
Mazzarino et al. [Citation118] reported that nanoemulsions containing the fruit peel extract of Plinia peruviana showed promising anti-oxidant capacity, namely as active ingredients in anti-aging cosmetic products. This is because the P. peruviana peel extract is rich in phenolic anti-oxidants, such as anthocyanin, gallic acid, ellagic acid, isoquercitrin, quercimeritrin, quercitrin, myricitrin and quercetin. Likewise, anti-oxidant assays of nanoemulsions using jaboticaba extract as the active ingredient revealed the strong 1,1-diphenyl-2-picrylhydrazyl (DPPH) and ferric reducing power thus feasible as a topical anti-aging cosmetic product [Citation118,Citation119].
Anti-wrinkle
The first noticeable signs of skin aging begin with the appearance of wrinkles on the surface of the stratum corneum. Wrinkles are formed as a result of environmental or nutritional instigated changes that are manifested as the degradation of the skin’s three primary structural constituents, namely, collagen, elastin and hyaluronic acid. The bond between the dermis and epidermis is weakened by wrinkles on the skin and degrades the fibril. The topical administration of nanoemulsions made from plant-based bioactive compounds could provide the moisturizing needed to attenuate the wrinkles [Citation120,Citation121]. A study by [Citation103] showed that the nanoemulsion contains Moringa oleifera seed oil to be an effective anti-wrinkle treatment when used topically on human skin. This has to do with the M. oleifera seed oil being rich in anti-oxidants. The treated skin appeared nourished, and fine lines and wrinkles disappeared upon topical application of this nanoemulsion. They believed that the W/O nanoemulsion of M. oleifera was well-dispersed and delivered through the stratum corneum due to its smallest droplet size of less than 100 nm. The anti-oxidative effect of the M. oleifera seed oil nanoemulsion stemmed from the colloidal system’s ability to prevent the loss of elastin and collagen. When topically applied at an extended duration, this eventually caused a profound reduction in skin wrinkles [Citation103].
The flavonoid-rich Marigold (Tagetes erecta Linn.) flower extract containing the hexahydroxyflavone quercetagetin was loaded into nanoemulsion and assessed for antiwrinkle efficacy on the stratum corneum [Citation122]. The active compound, quercetagetin, was successfully delivered into the skin and reduced skin wrinkles. Clinical studies using the T. erecta nanoemulsion revealed that the skin hydration on the stratum corneum was also improved. The wrinkle parameters, such as surface, volume, Ra and Rz, decreased significantly. The ability of the quercetagetin-rich nanoemulsion to reduce wrinkles may be explained by the compound’s ability to inhibit the UVB-induced phosphorylation of c-Jun and AK by binding to the signaling molecules c-Jun NH2-terminal kinase-1 and phosphatidylinositol 3-kinase [Citation122]. Quercetagetin also strongly suppresses thymus and activation-regulated chemokine and macrophage-derived chemokine production in HaCaT human keratinocytes. Therefore, this nanoemulsion has a promising use as an anti-wrinkle cosmetic product [Citation104].
Anti-acne
Acne currently affects approximately 9.4% of the global population, characterized by physical symptoms as soreness and itchiness. However, individuals inflicted with acne also suffer psychological issues such as self-esteem and degraded confidence. Acne-related inflammation is an immunomodulatory response towards the gram-positive anaerobe Propionibacterium acnes, which mainly inhabits the human pilosebaceous unit [Citation123]. Acne can be treated through the following pathways where i) P. acnes moieties stimulate the toll-like receptors (TLR-2,4,6) on keratinocytes, and ii) the activated TLRs trigger the release of inflammatory cytokines (TNF-á´¨, IL-6 and IL-8) by keratinocytes [Citation124]. The researchers found that the polyphenol-rich kernels of Mangifera indica L. formulated into a topical nanoemulsion that effectively reduced acne. The formulation exhibited notable antibacterial and cytokines anti-inflammatory properties. The ethanol fraction encapsulated nanoemulsion showed increased minimum inhibitory concentration (MIC) and minimum bactericidal concentration (MBC) when tested against P. acnes during the 90 days of storage. The M. indica L. anti-acne nanoemulsion showed long-term stability and better skin permeability [Citation105].
Likewise, nanoemulsion loaded with oregano essential oil of Origanum vulgare L. was developed by [Citation125] for acne skin. Their in vitro findings indicated that the nanoemulsion showed intense antimicrobial activity when tested against two acne-causing bacteria. The MIC and MBC of the Origanum vulgare L. was 0.34 mg/mL and 0.67 mg/mL for the Pseudomonas acne, and was 0.67 mg/mL and 1.34 mg/mL against the Staphylococcus epidermidis, signifying the potential of the nanoemulsion as a topical anti-acne agent [Citation125]. Another promising plant extract comes from cinnamon, widely known as a traditional medicine for various bacterial related diseases. A cinnamon extract anti-acne topical nanoemulsion was recently formulated and effectively inhibited Staphylococcus aureus that caused acne. The smallest particle size and PDI for the n-hexane extract of Cinnamomum burmanii loaded nanoemulsions were 25.8 nm and 0.48, respectively [Citation101].
Whitening agent
Skin hyperpigmentation is the most prevalent and worrisome phenomenon for both men and women. This has to do with the unsightly, excessive production of melanin or melanogenesis in both the dermis and epidermis layers of human skin. Nevertheless, melanogenesis or any related concerns, such as freckles, can be cured by avoiding UV exposure, the inhibition of tyrosinase and melanocyte metabolism, and preventing further melanin production by corneal ablation. The application of kojic acid may also help. This compound is a fungal metabolite from Aspergillus oryzae, well-known for its tyrosinase inhibitory property [Citation126]. Tyrosinase is instrumental in the metabolic action that causes hyperpigmentation of the human skin. This copper-containing membrane-bound enzyme’s role is to catalyze the biosynthesis of melanin in the human body [Citation127].
A relatively recent study experimented on a kojic monooleate (KMO)-enriched nanoemulsion for in-vitro cytotoxicity using 3-(4,5-dimethylthiazol-2-yl)-2,5-diphenyltetrazolium bromide (MTT) on the 3T3 cell of mouse embryonic fibroblast cells. The IC50 value of below 30 μg/mL indicated that the nanoemulsion was safe for topical application [Citation106]. In 2019, Ting et al. nano emulsified the adlay bran oil (ABO) and produced minute particles in the range of ∼150 nm, and the formulation exhibited potent topical whitening property. Tyrosinase activity and melanin production was reduced in both in-vitro and in-vivo assays, using the B16F10 cells and zebrafish embryos [Citation128]. Likewise, nano-encapsulated ellagic acid from pomegranate peels showed the most potent inhibition effect against tyrosinase enzyme with an IC50 value of 38.3 ± 0.09 µg/mL, making it the ideal whitening agent [Citation107].
Plant-based bioactive compounds and their mechanisms of action for skin improvement
Synthetic anti-oxidants, including butylated hydroxytoluene (BHT), butylated hydroxyanisole (BHA), propyl gallate (PG) and tert-butylhydroquinone (TBHQ), are not safe to be used in cosmetics. The substances may pose unwanted side effects such as skin allergy or perhaps cancer, with extended use [Citation129]. Natural plant-based bioactive constituents are the more attractive and safer substitutes over synthetic anti-oxidants as active ingredients in topical nanoemulsions to improve skin quality. Anti-aging properties in compounds, phenolic acids, terpenoids, polyunsaturated fatty acids, lycopene, resveratrol, lignan, tannins, indoles, flavonoids, coenzyme Q, polysaccharides and anthocyanins have been investigated and studied extensively [Citation130]. The anti-oxidants mentioned above can be extracted from other parts of the plant, including fruits, grains, seeds, roots, leaves, stems and flowers. Then, they are formulated into nanoemulsions for topical applications [Citation18]. summarises the plant-based bioactive compounds and their inhibition mechanisms with corresponding IC50 values of their in-vitro assays.
Table 3. Bioactive compounds, plant origins, inhibition mechanisms, particles size, PDI and inhibition values at IC50 for nanoemulsions.
Melanogenesis
Melanin is a color pigment whose pertinent role is to protect human skin against damaging UV radiation. The pigment is synthesized by melanocyte cells through the melanogenesis biosynthesis process. However, prolonged exposure to sun and skin conditions, such as hyperpigmentation, namely, melasma, freckles or ephelides, can cause melanin to be over secreted and cause undesirable skin darkening [Citation137]. Reactive oxygen species, i.e. hydrogen peroxide, resulting from overexposure to the sun, are produced in keratinocytes. The reaction subsequently triggers UV-induced melanogenesis or skin pigmentation [Citation138]. There are two categories of melanin pigments, the first being the black-brown eumelanin (darker melanin), and the second is the yellow to reddish pheomelanin (lighter melanin). Melanogenesis involves two steps, which are mediated by the tyrosinase enzyme. The first step is dihydoxyphenylalanine or DOPA synthesis, while the second step oxidizes DOPA to dopaquinone [Citation139]. Carefully selected bioactive compounds may prove useful in preventing skin darkening by inhibiting hyperpigmentation via two main mechanisms: the inhibition of tyrosinase activity and the suppression of melanosome transfer. In the first mechanism, the bioactive compounds can suppress melanogenesis by inhibiting the activity of 5,6-dihydroxyindole-2-carboxylic acid (DHICA) polymerase, which polymerizes the 5,6-DHICA, thereby reducing the eumelanin production without interfering with the mRNA gene expression. The compounds can also interfere with tyrosinase activity in the B16 murine melanoma cells without interfering with DNA expression. In the second mechanism, translocation of melanosomes from melanocytes to adjacent keratinocytes is suppressed by phytochemicals. This step essentially limits the accumulation of melanosomes-containing melanin in keratinocytes, thus keeping hyperpigmentation under control [Citation140,Citation141].
Since flavonoids have anti-hyperpigmentation property, the compounds are capable of altering the melanin synthetic pathway through the following detailed mechanisms: i) tyrosinase structure alteration, ii) chelation of copper, the tyrosinase co-factor, iii) tyrosinase competitive or non-competitive, and suicide inhibition, finally through iv) tyrosinase expression reduction [Citation142]. Park et al. [Citation70] reported that polyphenols from the P. granatum extract effectively inhibited UVB-induced damage in the skin. The plant extract protected UVB damage in human fibroblasts by upregulating collagen and downregulating the expression of MMP-1 [Citation70]. The photoprotective effect of a nanoemulsion containing sucupira oil from Pterodon emarginatus helped minimized the UV-induced stress on human stratum corneum. IL-6 and IL-8 were effectively downregulated, implying the nanoemulsion’s potential for immunomodulatory activity and minimizing inflamed skin due to UVA radiation [Citation131].
Collagenesis
Collagen is the protein found throughout the human body, made of repetitive monomers of tropocollagens in tissues. Collagenesis is a necessary naturally-occurring metabolic process that happens in the human skin to replace the self-degraded fibrils. At a young age, the collagens are tightly packed and orderly arranged but are lost in photo-aged skin. Keeping the collagens intact is vital for maintaining tissues and organs’ structural integrity in terms of cell or tissue repair [Citation143]. There are two steps for collagen biosynthesis, which are intracellular and extracellular. The biosynthesis of collagen in human skin involves translating the collagen mRNA in polysomes and the hydroxylation of the peptidyl proline and lysine using specific enzymes. Disulfide bonds form the triple helix, and, lastly, is the enzyme-aided glycosylation [Citation144]. Prolonged exposure to UV radiation intensifies collagen degradation by producing metalloproteinase (MMP) 1 (collagenase), MMP-3 (stromelysin 1), and MMP-9 (92-kD gelatinase) in human dermal fibroblasts (dermis). UV exposure profoundly increases the cleavage of collagen by MMP 1 in the fibroblasts of human skin. It leads to excessive molecular denaturation that lowers collagen’s stability and reduces its elasticity in human skin. Likewise, Kontomaris et al., showed that UV irradiation caused a significant decline in Young’s modulus values in collagen fibrils and fibril diameters, length and leads [Citation145]. The above results thus validated the destroyed structural integrity seen in photo-aged human skin, such as dryness, wrinkle formation [Citation146]. Studies have shown that UVA rays exhibit a more substantial effect than UVB rays in destroying collagen, where wavelengths of 300-340 nm caused hardening and reduced elasticity of in-vitro tested collagen gels, with a pronounced effect at 330-nm radiation [Citation147].
In the ongoing investigation for skin-improving nanoemulsions Dhawan and Nanda [Citation132] developed a topically applied nanoemulsion using pomegranate seed oil. The formulation exhibited relatively potent anti-elastase, anti-collagenase and anti-hyaluronidase properties with an IC50 value of 309 mg/mL, 4 mg/mL and 95 mg/mL, respectively [Citation132]. Nanoemulsions can boosts the action of wound healing bioactive ingredients, as demonstrated by Alam et al. in 2016 using the wound excision rat model. The orally administered rat models showed a large amount of granulation tissue, restoration of the adnexa, and extensive fibrosis, allowing the wound to recover faster [Citation133].
Free radical scavenging activity
Anti-oxidants protect against UV-induced photodamage and inhibit inflammation on human skin by scavenging the harmful free radicals. Topical formulations loaded with anti-oxidants have been proven to offer anti-aging protection by shielding the human skin by absorbing UV radiation [Citation148]. Anti-oxidant mechanisms of polyphenols can be divided into three, with the first two mechanisms involving hydrogen atom transfer (HAT) (mechanism 1) and single electron transfer (SET) (mechanism 2) of the free radicals inactivated by the polyphenols. The polyphenols (ArOH) react with a free radical, R● by donating a hydrogen atom through a homolytic cleavage of the O-H bond. On the other hand, the second mechanism delineates an electron’s transfer to the free radical, R● to form a polyphenolic cation, ArOH+, and anion, R−. The third mechanism is transition metal chelation (TMC). The polyphenols chelate the transition metals or ligands into forming stable complexes so that the metal ions are incapable of creating free radicals for the oxidative process [Citation149].
Encapsulated plant-based anti-oxidants or bioactive compounds in hydrophilic/lipophilic nanoemulsions can undergo oxidation or phase separation (flocculation, coalescence and sedimentation) [Citation150,Citation151]. Literature has shown that harmful free radicals are the primary cause of unwanted oxidation in W/O or O/W nanoemulsions. The change comes from surface interaction between lipid hydroperoxides, transition metals, or pro-oxidants in the aqueous phase and vice versa [Citation152]. Moreover, free radical scavengers are likely effective surface-active anti-oxidants mainly found in zones where the free radicals are created. Therefore, the higher surface area of the smallest particle size in nanoemulsions impedes the lipid oxidation reaction, which mainly occurs at the oil-water interface [Citation153,Citation154].
Commercial applications and patented nanocosmetics
Commercial/practical applications of nanocosmetics are patented yearly by established cosmetic companies. These patents encompass nanoemulsions alongside a wide range of delivery systems such as nanoparticles, nanosomes, nanospheres, niosomes, nanocapsules and nanoemulsions. As a matter of fact, nearly all fields of application have found nanoemulsions to be highly useful. and enlist the commercially available nanocosmetics with their corresponding applications, alongside known patented nanocosmetics, respectively. Several commercial cosmetics products are sold as anti-wrinkle, anti-acne, moisturizer, UV protection and whitening agents [Citation155,Citation156].
Table 4. List of commercially available nanocosmetics for different applications.
Table 5. List of patented nanocosmetics.
Patents numbers US 20130011348A1 and US 20090220556A1 by Tomiko Takakura and International Technology Center, respectively described nanoformulations for specific use as sunscreens or UV protection. Meanwhile, the method for stabilizing retinol (vitamin A) for wrinkle and fine lines-reducing nanoemulsion was patented by Act Co Ltd (patent number US 20130095157A1 dated April 18, 2013) and (patent number EP 2583665A2 dated April 24, 2013). The retinol polymer nanocapsule was fashioned by nano emulsifying retinol in porous polymer beads of 50–200 nm in sizes [Citation16,Citation157].
Conclusions and future outlook
The past decade has seen that plant-based bioactive compounds loaded nanoemulsions have found useful applications in the cosmeceutical industry, producing a wide-ranging product with photoprotection, moisturizer and anti-aging and anti-wrinkle capabilities. Nonetheless, there are many challenges ahead and also plenty of room for improvement by the formulation scientist as plant-based-bioactive compounds tend to possess low permeability into the stratum corneum. The plant bioactive ingredients’ tendency to form relatively large size droplets in the emulsion denote the impending low stability and their poorer permeation through the skin. Nanotechnology has mostly overcome this shortcoming, whereby cosmetics employing nano-sized plant-based bioactive compounds have increased their bioavailability into the skin. The phytoconstituents are retained on the surface of the stratum corneum for a more extended period, thereby improving skin hydration, skin elasticity and UV protection effects, and thus, delay skin aging [158].
Plant-based nanoemulsions in cosmeceuticals are a promising nano-delivery technique for the future. Thus, the next decade foresees a higher market trend for nanocosmeceutical products containing primarily naturally extract compounds, replacing synthetic chemicals. This is because of consumers’ growing awareness for safer products to be used on the skin. Aside from the more refined texture and ease of applying nanoemulsions, choosing the right natural plant ingredients hinges on the cosmetic product’s requirements. Further research is needed to improve plant-based nano-cosmetics nanotechnology, thereby ensuring that nano-scaled plant extract-loaded formulation remains the best and safest choice in the coming years. Specifically, nanoemulsions are prepared for the well-being and safety of consumers. With increasing reports of evidence-based herbal biotechnology on phytohormones and their physiologic effects, future market trends will see a higher demand for nano-formulated phytohormones for repairing and/or rejuvenating human skin. By combining the cutting-edge nano-dermatology research with Ayurvedic and ancient herbal medicine based on plant stem cells, a higher preference for vegan cosmetics is expected, if not already trending, among consumers across diverse regions.
Disclsoure statement
The authors have no conflicts of interest to declare that are relevant to the content of this article.
References
- Bale S, Cameron J, Meaume S, et al. Skin care. In: Romanelli M, Clark M, Cherry GW, Colin D, Defloor T, editors. Science and practice of pressure ulcer management. London: Springer; 2018. p. 111–124.
- Kong M, Chen XG, Kweon DK, et al. Investigations on skin permeation of hyaluronic acid based nanoemulsion as transdermal carrier. Carbohydr Polym. 2011;86(2):837–843.
- Otlatıcı G, Yeğen G, Güngör S, et al. Overview on nanotechnology based cosmeceuticals to prevent skin aging. Istanb J Pharm. 2018;48(2):55–62.
- Menon GK, Cleary GW, Lane ME. The structure and function of the stratum corneum. Int J Pharm. 2012;435(1):3–9.
- El-Leithy ES, Makky AM, Khattab AM, et al. Optimization of nutraceutical coenzyme Q10 nanoemulsion with improved skin permeability and anti-wrinkle efficiency. Drug Dev Ind Pharm. 2018;44(2):316–328.
- Van Tran V, Nguyen TL, Moon J-Y, et al. Core-shell materials, lipid particles and nanoemulsions, for delivery of active anti-oxidants in cosmetics applications: challenges and development strategies. Chem Eng J. 2019;368:88–114.
- Mukul S, Surabhi K, Atul N. Cosmeceuticals for the skin: An overview. Asian J Pharm Clin Res. 2011;4(2):1–6.
- Ribeiro AS, Estanqueiro M, Oliveira MB, et al. Main benefits and applicability of plant extracts in skin care products. Cosmetics. 2015;2(2):48–65.
- Bocheva G, Slominski RM, Slominski AT. Neuroendocrine aspects of skin aging. Int J Mol Sci. 2019;20(11):2798.
- Khogta S, Patel J, Barve K, et al. Herbal nano-formulations for topical delivery. J Herb Med. 2020;20:100300.
- Kashif M, Akhtar N, Mustafa R. An overview of dermatological and cosmeceutical benefits of Diospyros kaki and its phytoconstituents. Rev Bras Farmacogn. 2017;27(5):650–662.
- Faria-Silva C, Ascenso A, Costa AM, et al. Feeding the skin: A new trend in food and cosmetics convergence. Trends Food Sci Technol. 2020;95:21–32.
- Mu L, Sprando RL. Application of nanotechnology in cosmetics. Pharm Res. 2010;27(8):1746–1749.
- Dasgupta N, Ranjan S, Gandhi M. Nanoemulsion ingredients and components. Environ Chem Lett. 2019;17(2):917–928.
- Walia N, Dasgupta N, Ranjan S, et al. Fish oil based vitamin D nanoencapsulation by ultrasonication and bioaccessibility analysis in simulated gastro-intestinal tract. Ultrason Sonochem. 2017;39:623–635.
- Che Marzuki NH, Wahab RA, Abdul Hamid M. An overview of nanoemulsion: concepts of development and cosmeceutical applications. Biotechnol Biotechnol Equip. 2019;33(1):779–797.
- McClements DJ. Nanoemulsions versus microemulsions: terminology, differences, and similarities. Soft Matter. 2012;8(6):1719–1729.
- Costa R, Santos L. Delivery systems for cosmetics-From manufacturing to the skin of natural antioxidants. Powder Technol. 2017;322:402–416.
- Giacone DV, Dartora VFMC, de Matos JKR, et al. Effect of nanoemulsion modification with chitosan and sodium alginate on the topical delivery and efficacy of the cytotoxic agent piplartine in 2D and 3D skin cancer models. Int J Biol Macromol. 2020;165:1055–1065.
- Mustafa IF, Hussein MZ. Synthesis and technology of nanoemulsion-based pesticide formulation. Nanomaterials. 2020;10(8):1608.
- Singh Y, Meher JG, Raval K, et al. Nanoemulsion: Concepts, development and applications in drug delivery. J Control Release. 2017;252:28–49.
- Kelmann RG, Colombo M, Nunes RJ, et al. Nanoemulsion-loaded hydrogels for topical administration of pentyl gallate. AAPS PharmSciTech. 2018;19(6):2672–2678.
- Nishitani Yukuyama M, Tomiko Myiake Kato E, Lobenberg R, et al. Challenges and future prospects of nanoemulsion as a drug delivery system. Curr Pharm Des. 2017;23(3):495–508.
- Sonneville-Aubrun O, Yukuyama MN, Pizzino A. Application of nanoemulsions in cosmetics. In: Jafari S, McClements DJ, editors. Nanoemulsions. Amsterdam: Elsevier; 2018. p. 435–475.
- Azmi NAN, Elgharbawy AAM, Motlagh SR, et al. Nanoemulsions: factory for food, pharmaceutical and cosmetics. Processes. 2019;7(9):617.
- Costa M, Freiría-Gándara J, Losada-Barreiro S, et al. Effects of droplet size on the interfacial concentrations of antioxidants in fish and olive oil-in-water emulsions and nanoemulsions and on their oxidative stability. J Colloid Interface Sci. 2020;562:352–362.
- Lee C-H, Huang G-C, Chen C-Y. Bioactive compounds from natural product extracts in Taiwan cosmeceuticals-Mini review. Biomed Res. 2017;28(15):6561.
- Antonopoulou I, Varriale S, Topakas E, et al. Enzymatic synthesis of bioactive compounds with high potential for cosmeceutical application. Appl Microbiol Biotechnol. 2016;100(15):6519–6543.
- Saewan N, Jimtaisong A. Natural products as photoprotection. J Cosmet Dermatol. 2015;14(1):47–63.
- Ahsan H. The biomolecules of beauty: biochemical pharmacology and immunotoxicology of cosmeceuticals. J Immunoassay Immunochem. 2019;40(1):91–108.
- Santos AC, Morais F, Simões A, et al. Nanotechnology for the development of new cosmetic formulations. Expert Opin Drug Deliv. 2019;16(4):313–330.
- Lagoa R, Silva J, Rodrigues JR, et al. Advances in phytochemical delivery systems for improved anticancer activity. Biotechnol Adv. 2020;38:107382.
- Sanjeewa KKA, Kim E-A, Son K-T, et al. Bioactive properties and potentials cosmeceutical applications of phlorotannins isolated from brown seaweeds: a review. J Photochem Photobiol B. 2016;162:100–105.
- Farahin AW, Yusoff FM, Basri M, et al. Use of microalgae: Tetraselmis tetrathele extract in formulation of nanoemulsions for cosmeceutical application. J Appl Phycol. 2019;31(3):1743–1752.
- Ganesan P, Choi D-K. Current application of phytocompound-based nanocosmeceuticals for beauty and skin therapy. Int J Nanomed. 2016;11:1987.
- Puglia C, Santonocito D. Cosmeceuticals: nanotechnology-based strategies for the delivery of phytocompounds. Curr Pharm Des. 2019;25(21):2314–2322.
- Bidone J, Argenta DF, Kratz J, et al. Antiherpes activity and skin/mucosa distribution of flavonoids from achyrocline satureioides extract incorporated into topical nanoemulsions. BioMed Res Int. 2015;2015:1–7.
- Chaiittianan R, Sripanidkulchai B. Development of a nanoemulsion of Phyllanthus emblica L. branch extract. Drug Dev Ind Pharm. 2014;40(12):1597–1606.
- Isnaini N. Preparation and investigation of antioxidant, antibacterial and antityrosinase potentials of o/w nanoemulsions containing extract of Tamarindus indica L. Fruit Pulp. Hat Yai: Prince of Songkla University; 2019.
- Baccarin T, Lemos-Senna E. Potential application of nanoemulsions for skin delivery of pomegranate peel polyphenols. AAPS PharmSciTech. 2017;18(8):3307–3314.
- Fasolo D, Bassani VL, Teixeira HF. Development of topical nanoemulsions containing quercetin and 3-O-methylquercetin. Pharmazie. 2009;64(11):726–730.
- Ferreira-Nunes R, da Silva SMM, de Souza PEN, et al. Incorporation of Eugenia dysenterica extract in microemulsions preserves stability, antioxidant effect and provides enhanced cutaneous permeation. J Mol Liq. 2018;265:408–415.
- Hernández-Jaimes C, Fouconnier B, Pérez-Alonso C, et al. Antioxidant activity degradation, formulation optimization, characterization, and stability of Equisetum arvense extract nanoemulsion. J Dispersion Sci Technol. 2013;34(1):64–71.
- Algahtani MS, Ahmad MZ, Ahmad J. Nanoemulsion loaded polymeric hydrogel for topical delivery of curcumin in psoriasis. J Drug Delivery Sci Technol. 2020;59:101847.
- Thomas L, Zakir F, Mirza MA, et al. Development of curcumin loaded chitosan polymer based nanoemulsion gel: In vitro, ex vivo evaluation and in vivo wound healing studies. Int J Biol Macromol. 2017;101:569–579.
- Ascenso A, Pinho S, Eleutério C, et al. Lycopene from tomatoes: vesicular nanocarrier formulations for dermal delivery. J Agric Food Chem. 2013;61(30):7284–7293.
- Yang S, Liu L, Han J, et al. Encapsulating plant ingredients for dermocosmetic application: an updated review of delivery systems and characterization techniques. Int J Cosmet Sci. 2019;42:16–28.
- Pereira AB, da Silva AM, Barroca MJ, et al. Physicochemical properties, antioxidant action and practical application in fresh cheese of the solid inclusion compound γ-cyclodextrin quercetin, in comparison with β-cyclodextrin· quercetin. Arab J Chem. 2020;13(1):205–215.
- D’Andrea G. Quercetin: a flavonol with multifaceted therapeutic applications?Fitoterapia. 2015;106:256–271.
- Nagula RL, Wairkar S. Recent advances in topical delivery of flavonoids: A review. J Control Release. 2019;296:190–201.
- Bose S, Michniak-Kohn B. Preparation and characterization of lipid based nanosystems for topical delivery of quercetin. Eur J Pharm Sci. 2013;48(3):442–452.
- Lesjak M, Beara I, Simin N, et al. Antioxidant and anti-inflammatory activities of quercetin and its derivatives. J Funct Foods. 2018;40(October 2017):68–75.
- Park EJ, Kim J-Y, Jeong MS, et al. Effect of topical application of quercetin-3-O-(2 ″-gallate)-α-l-rhamnopyranoside on atopic dermatitis in NC/Nga mice. J Dermatol Sci. 2015;77(3):166–172.
- Karadag A, Yang X, Ozcelik B, et al. Optimization of preparation conditions for quercetin nanoemulsions using response surface methodology. J Agric Food Chem. 2013;61(9):2130–2139.
- Ebrahimi P, Salmanpour S. Topical Quercetin Nanoemulsions: Optimization of preparation using chemometric approaches. Pharm Chem J. 2014;48(6):402–407.
- Isemura M. Catechin in human health and disease. Basel: Multidisciplinary Digital Publishing Institute; 2019.
- Higdon JV, Frei B. Tea catechins and polyphenols: health effects, metabolism, and antioxidant functions. Crit Rev Food Sci Nutr. 2003;43(1):89–143.
- Shi M, Nie Y, Zheng X-Q, et al. Ultraviolet B (UVB) photosensitivities of tea catechins and the relevant chemical conversions. Molecules. 2016;21(10):1345.
- Yoshino S, Mitoma T, Tsuruta K, et al. Effect of emulsification on the skin permeation and UV protection of catechin. Pharm Dev Technol. 2014;19(4):395–400.
- Bae J, Kim N, Shin Y, et al. Activity of catechins and their applications. Biomed Dermatol. 2020;4(1):1–10.
- Kim E, Hwang K, Lee J, et al. Skin protective effect of epigallocatechin gallate. Int J Mol Sci. 2018;19(1):173.
- Kim H, Kim JT, Barua S, et al. Seeking better topical delivery technologies of moisturizing agents for enhanced skin moisturization. Expert Opin Drug Deliv. 2018;15(1):17–31.
- Schaechter M. Encyclopedia of microbiology. Cambridge MA: Academic Press; 2009.
- Erukainure OL, Sanni O, Islam MS. Clerodendrum volubile: phenolics and applications to health. In: Watson R, Preedy V, Zibadi S, editors. Polyphenols: Mechanisms of action in human health and disease. Amsterdam: Elsevier; 2018. p. 53–68.
- Chackalamannil S, Rotella D, Ward S. Comprehensive medicinal chemistry III. Amsterdam: Elsevier; 2017.
- Rosas EC, Correa LB, das Graças Henriques M. Antiinflammatory properties of Schinus terebinthifolius and its use in arthritic conditions. In Watson R, Preedy V, editors. Bioactive food as dietary interventions for arthritis and related inflammatory diseases. Amsterdam: Elsevier; 2019. p. 489–505.
- Mitsou E, Pletsa V, Sotiroudis GT, et al. Development of a microemulsion for encapsulation and delivery of gallic acid. The role of chitosan. Colloids Surf B. 2020;190:110974.
- Baccarin T, Lemos-Senna E. Pomegranate seed oil nanoemulsions encapsulating pomegranate peel polyphenol-rich ethyl acetate fraction: development and antioxidant assessment. J Nanopharm Drug Deliv. 2014;2(4):333–343.
- Park HM, Moon E, Kim A, et al. Extract of Punica granatum inhibits skin photoaging induced by UVB irradiation. Inte J Dermatol. 2010;49(3):276–282.
- Zillich OV, Schweiggert - Weisz U, Eisner P, et al. Polyphenols as active ingredients for cosmetic products. Int J Cosmet Sci. 2015;37(5):455–464.
- Chen X, Ba Y, Ma L, et al. Characterization of microRNAs in serum: a novel class of biomarkers for diagnosis of cancer and other diseases. Cell Res. 2008;18(10):997–1006.
- Ackland ML, Van De Waarsenburg S, Jones R. Synergistic antiproliferative action of the flavonols quercetin and kaempferol in cultured human cancer cell lines. In Vivo. 2005;19(1):69–76.
- Sekiguchi A, Motegi S, Fujiwara C, et al. Inhibitory effect of kaempferol on skin fibrosis in systemic sclerosis by the suppression of oxidative stress. J Dermatol Sci. 2019;96(1):8–17.
- Masibo M, He Q. Major mango polyphenols and their potential significance to human health. Compr Rev Food Sci Food Saf. 2008;7(4):309–319.
- Alam W, Khan H, Shah MA, et al. Kaempferol as a dietary anti-inflammatory agent: Current therapeutic standing. Molecules. 2020;25(18):4073.
- Jeon AJ, Lim T-G, Jung SK, et al. Black soybean (Glycine max cv. Heugmi) seed coat extract suppresses tpa or uvb-induced cox-2 expression by blocking mitogen activated protein kinases pathway in mouse skin epithelial cells. Food Sci Biotechnol. 2011;20(6):1735–1741.
- Chen AY, Chen YC. A review of the dietary flavonoid, kaempferol on human health and cancer chemoprevention. Food Chem. 2013;138(4):2099–2107.
- Jiang T, Liao W, Charcosset C. Recent advances in encapsulation of curcumin in nanoemulsions: A review of encapsulation technologies, bioaccessibility and applications. Food Res Int. 2020;132:109035.
- Rafiee Z, Nejatian M, Daeihamed M, et al. Application of curcumin-loaded nanocarriers for food, drug and cosmetic purposes. Trends Food Sci Technol. 2019;88:445–458.
- Iqbal R, Mehmood Z, Baig A, et al. Formulation and characterization of food grade O/W nanoemulsions encapsulating quercetin and curcumin: Insights on enhancing solubility characteristics. Food Bioprod Process. 2020;123:304–311.
- Murthy KNC, Monika P, Jayaprakasha GK, et al. Nanoencapsulation: An advanced nanotechnological approach to enhance the biological efficacy of curcumin. In: Jayaprakasha GK, Patil BS, Gattuso G, editors. Advances in plant phenolics: from chemistry to human health. Washington: ACS Publications; 2018. p. 383–405.
- Palliyage GH, Singh S, Ashby CR, et al. Pharmaceutical topical delivery of poorly soluble polyphenols: potential role in prevention and treatment of melanoma. AAPS PharmSciTech. 2019;20(6):1–24.
- Arora D, Jaglan S. Therapeutic applications of resveratrol nanoformulations. Environ Chem Lett. 2018;16(1):35–41.
- Sharma B, Iqbal B, Kumar S, et al. Resveratrol-loaded nanoemulsion gel system to ameliorate UV-induced oxidative skin damage: from in vitro to in vivo investigation of antioxidant activity enhancement. Arch Dermatol Res. 2019;311(10):773–793.
- Saini A, Panesar PS, Bera MB. Valorization of fruits and vegetables waste through green extraction of bioactive compounds and their nanoemulsions-based delivery system. Bioresour Bioprocess. 2019;6(1):1–12.
- Butnariu MV, Giuchici CV. The use of some nanoemulsions based on aqueous propolis and lycopene extract in the skin’s protective mechanisms against UVA radiation. J Nanobiotechnol. 2011;9(1):3.
- Cooperstone JL, Tober KL, Riedl KM, et al. Tomatoes protect against development of UV-induced keratinocyte carcinoma via metabolomic alterations. Sci Rep. 2017;7(1):1–9.
- Ascenso A, Pedrosa T, Pinho S, et al. The effect of lycopene preexposure on UV-B-irradiated human keratinocytes. Oxid Med Cell Longev. 2016.
- Yahya NA, Attan N, Wahab RA. An overview of cosmeceutically relevant plant extracts and strategies for extraction of plant-based bioactive compounds. Food Bioprod Process. 2018;112:69–85.
- Beltrán Sanahuja A, Maestre Pérez SE, Grané Teruel N, et al. Variability of chemical profile in almonds (Prunus dulcis) of different cultivars and origins. Foods. 2021;10(1):153.
- Kahlaoui M, Borotto Dalla Vecchia S, Giovine F, et al. Characterization of polyphenolic compounds extracted from different varieties of Almond Hulls (Prunus dulcis L.). Antioxidants. 2019;8(12):647.
- Balakrishnan R, Vijayraja D, Jo S-H, et al. Medicinal profile, phytochemistry, and pharmacological activities of Murraya koenigii and its primary bioactive compounds. Antioxidants. 2020;9(2):101.
- Santos AC, Rodrigues D, Sequeira JAD, et al. Nanotechnological breakthroughs in the development of topical phytocompounds-based formulations. Int J Pharm. 2019;572:118787.
- Sulaiman ISBC. Design and optimization of nanoemulsion formulation containing Clinacanthus nutans Lindau leaf extract for cosmeceutical application. Seri Kembangan: Universiti Putra Malaysia; 2017.
- Sulaiman ISC, Basri M, Masoumi HRF, et al. Design and development of a nanoemulsion system containing extract of Clinacanthus nutans (L.) leaves for transdermal delivery system by D-optimal mixture design and evaluation of its physicochemical properties. RSC Adv. 2016;6(71):67378–67388.
- Ribeiro RCA, Barreto SMAG, Ostrosky EA, et al. Production and characterization of cosmetic nanoemulsions containing Opuntia ficus-indica (L.) Mill extract as moisturizing agent. Molecules. 2015;20(2):2492–2509.
- Back PI, Furtado LR, Nemitz MC, et al. Skin permeation and oxidative protection effect of soybean isoflavones from topical nanoemulsions—a comparative study of extracts and pure compounds. AAPS PharmSciTech. 2018;19(7):3029–3039.
- Barreto SMAG, Maia MS, Benicá AM, et al. Evaluation of in vitro and in vivo safety of the by-product of Agave sisalana as a new cosmetic raw material: Development and clinical evaluation of a nanoemulsion to improve skin moisturizing. Ind. Crops Prod. 2017;108:470–479. doi:10.1016/j.indcrop.2017.06.064.
- Balestrin LA, Bidone J, Bortolin RC, et al. Protective effect of a hydrogel containing Achyrocline satureioides extract-loaded nanoemulsion against UV-induced skin damage. J Photochem Photobiol B Biol. 2016;163:269–276. doi:10.1016/j.jphotobiol.2016.08.039.
- Setyawati A, Mardyaningrum LW, Damayanti T. Formulation, physical stability test and anti-bacterial test of nanoemulsion from water and n-hexane extract of Cinnamomum burmanii. AIP Conf Proc. 2020;2229(1):30031.
- Duraivel S, Asma SS, Basha SK. Formulation and evaluation of anti-wrinkle activity of cream and nanoemulsion of Moringa oleifera seed oil. IOSR J Pharm Biol Sci. 2014;9(4):58–73.
- Mansur MCPPR, Campos C, Vermelho AB, et al. Photoprotective nanoemulsions containing microbial carotenoids and buriti oil: Efficacy and safety study. Arabian J Chem. 2020;13(8):6741–6752. doi:10.1016/j.arabjc.2020.06.028.
- Leelapornpisid P, Kiattisin K, Jantrawut P, et al. Nanoemulsion loaded with marigold flower extract (Tagetes erecta Linn) in gel preparation as anti-wrinkles cosmeceutical. Int J Pharm Pharm Sci. 2014;6(2):231–236.
- Poomanee W, Khunkitti W, Chaiyana W, et al. Optimization of Mangifera indica L. kernel extract-loaded nanoemulsions via response surface methodology, characterization, stability, and skin permeation for anti-acne cosmeceutical application. Pharmaceutics. 2020;12(5):454.
- Azhar SNAS, Ashari SE, Salim N. Development of a kojic monooleate-enriched oil-in-water nanoemulsion as a potential carrier for hyperpigmentation treatment. Int J Nanomed. 2018;13:6465.
- Zhang H, Zhao Y, Ying X, et al. Ellagic acid nanoemulsion in cosmetics: The preparation and evaluation of a new nanoemulsion method as a whitening and antiaging agent. IEEE Nanotechnol Mag. 2018;12(1):14–20.
- CamargoJrFB, Gaspar LR, Maia Campos PMBG. Skin moisturizing effects of panthenol-based formulations. J Cosmet Sci. 2011;62(4):361.
- Buranajaree s, Donsing P. Depigmenting action of a nanoemulsion containing heartwood extract of Artocarpus incisus on UVB-induced hyperpigmentation in C57BL/6 mice. J Cosm Sci. 2011;62(1):1.
- Afornali A, Lorencini M. Nanoemulsions to prevent photoaging. In: Hamblin MR, Avci P, Prow TW, editors. Nanoscience in dermatology. Cambridge, MA: Elsevier; 2016. p. 237–246.
- Miller T. Clinical testing to uphold an anti-aging claim. In: Dayan N, editor. Skin aging handbook. Amsterdam: Elsevier; 2009. p. 363–389.
- Korać RR, Khambholja KM. Potential of herbs in skin protection from ultraviolet radiation. Pharmacogn Rev. 2011;5(10):164.
- Saraf S, Kaur CD. Phytoconstituents as photoprotective novel cosmetic formulations. Pharmacogn Rev. 2010;4(7):1.
- Shapiro SS, Seiberg M, Cole CA. Vitamin A and its derivatives in experimental photocarcinogenesis: preventive effects and relevance to humans. J Drugs Dermatol. 2013;12(4):458–463.
- Clares B, Calpena AC, Parra A, et al. Nanoemulsions (NEs), liposomes (LPs) and solid lipid nanoparticles (SLNs) for retinyl palmitate: Effect on skin permeation. Int J Pharm. 2014;473(1–2):591–598.
- Wang SQ, Dusza SW, Lim HW. Safety of retinyl palmitate in sunscreens: a critical analysis. J Am Acad Dermatol. 2010;63(5):903–906.
- Zorzi GK, Caregnato F, Moreira JCF, et al. Antioxidant effect of nanoemulsions containing extract of Achyrocline satureioides (Lam) DC—Asteraceae. AAPS PharmSciTech. 2016;17(4):844–850.
- Mazzarino L, da Silva Pitz H, Lorenzen Voytena AP, et al. Jaboticaba (Plinia peruviana) extract nanoemulsions: development, stability, and in vitro antioxidant activity. Drug Dev Ind Pharm. 2018;44(4):643–651.
- Rahman HS, Othman HH, Hammadi NI, et al. Novel drug delivery systems for loading of natural plant extracts and their biomedical applications. Int J Nanomed. 2020;15:2439.
- Baumann L. Skin ageing and its treatment. J Pathol. 2007;211(2):241–251.
- Souto EB, Fernandes AR, Martins-Gomes C, et al. Nanomaterials for skin delivery of cosmeceuticals and pharmaceuticals. Appl Sci. 2020;10(5):1594.
- Baek S, Kang NJ, Popowicz GM, et al. Structural and functional analysis of the natural JNK1 inhibitor quercetagetin. J Mol Biol. 2013;425(2):411–423.
- Poomanee W, Chaiyana W, Mueller M, et al. In-vitro investigation of anti-acne properties of Mangifera indica L. kernel extract and its mechanism of action against Propionibacterium acnes. Anaerobe. 2018;52:64–74.
- Sinha P, Srivastava S, Mishra N, et al. New perspectives on antiacne plant drugs: contribution to modern therapeutics. BioMed Res Int. 2014;2014:1–19.
- Taleb MH, Abdeltawab NF, Shamma RN, et al. Origanum vulgare L. essential oil as a potential anti-acne topical nanoemulsion—in vitro and in vivo study. Molecules. 2018;23(9):2164.
- Rigopoulos D, Gregoriou S, Katsambas A. Hyperpigmentation and melasma. J Cosmet Dermatol. 2007;6(3):195–202.
- Kim Y-J, Uyama H. Tyrosinase inhibitors from natural and synthetic sources: structure, inhibition mechanism and perspective for the future. Cell Mol Life Sci. 2005;62(15):1707–1723.
- Ting Y, Hu Y, Hu J, et al. Nanoemulsified adlay bran oil reduces tyrosinase activity and melanin synthesis in B16F10 cells and zebrafish. Food Sci Nutr. 2019;7(10):3216–3223.
- Romes NB, Wahab RA, Hamid MA. Proximate analysis and bioactivity study on acoustically isolated Elaeis guineensis leaves extract. AIP Conf Proc. 2019;2155(1):20001.
- Ingle AP, Shende S, Gupta I, et al. Recent trends in the development of nano-bioactive compounds and delivery systems. In: Verma M, Chandel A, editors. Biotechnological Production of Bioactive Compounds. Amsterdam: Elsevier; 2020. p. 409–431.
- Pacheco MT, Silva ACG, Nascimento TL, et al. Protective effect of sucupira oil nanoemulsion against oxidative stress in UVA-irradiated HaCaT cells. J Pharm Pharmacol. 2019;71(10):1532–1543.
- Dhawan S, Nanda S. In vitro estimation of photo-protective potential of pomegranate seed oil and development of a nanoformulation. Curr Nutr Food Sci. 2019;15(1):87–102.
- Alam P, Ansari MJ, Anwer MK, et al. Wound healing effects of nanoemulsion containing clove essential oil. Artif Cells Nanomed Biotechnol. 2017;45(3):591–597.
- Vaibhav S, Lakshaman K. Tyrosinase enzyme inhibitory activity of selected Indian herbs. Int J Res Pharm Biomed Sci. 2012;3:977–982.
- Eid AM, Jaradat NA, Al-Masri M, et al. Development and antimicrobial evaluation of Eruca sativa oil nanoemulgel with determination of the oil antioxidant, sun protection factor and elastase inhibition. Curr Pharm Biotechnol. 2020;21(3):244–255.
- Mahdi ES, Noor AM, Sakeena MH. Formulation and in vitro release evaluation of newly synthesized palm kernel oil esters-based nanoemulsion delivery system for 30% ethanolic dried extract derived from local Phyllanthus urinaria for skin antiaging. Int J Nanomed. 2011;6:2499.
- Dal Mas J, Zermiani T, Thiesen, LC, et al. Nanoemulsion as a carrier to improve the topical anti-inflammatory activity of stem bark extract of Rapanea ferruginea. Int J Nanomed. 2016;11:4495. doi:10.2147/IJN.S110486.
- Masaki H. Role of antioxidants in the skin: anti-aging effects. J Dermatol Sci. 2010;58(2):85–90.
- Ortonne J. Photoprotective properties of skin melanin. Br J Dermatol. 2002;146:7–10.
- Hanif N, Al-Shami AMA, Khalid KA, et al. Plant-based skin lightening agents: a review. J Phytopharmacol 2020;9(1):54–60.
- Katiyar S, Saify K, Singh SK, et al. Botanical study of skin lightening agents. Int J Pharmacogn. 2014;1(4):243–249.
- Ephrem E, Elaissari H, Greige-Gerges H. Improvement of skin whitening agents efficiency through encapsulation: Current state of knowledge. Int J Pharm. 2017;526(1–2):50–68.
- Gelse K, Pöschl E, Aigner T. Collagens—structure, function, and biosynthesis. Adv Drug Delivery Rev. 2003;55(12):1531–1546.
- Perez-Tamayo R. Pathology of collagen degradation. A review. Am J Pathol. 1978;92(2):508.
- Kontomaris SV, Yova D, Stylianou A, et al. The effects of UV irradiation on collagen D-band revealed by atomic force microscopy. Scanning. 2015;37(2):101–111.
- Fisher GJ, Kang S, Varani J, et al. Mechanisms of photoaging and chronological skin aging. Arch Dermatol. 2002;138(11):1462–1470.
- Maeda K. Analysis of ultraviolet radiation wavelengths causing hardening and reduced elasticity of collagen gels in vitro. Cosmetics. 2018;5(1):14.
- Ramos-e-Silva M, Celem LR, Ramos e, Silva, et al. Anti-aging cosmetics: Facts and controversies. Clin Dermatol. 2013;31(6):750–758.
- Leopoldini M, Russo N, Toscano M. The molecular basis of working mechanism of natural polyphenolic antioxidants. Food Chem. 2011;125(2):288–306.
- Jafari SM, Paximada P, Mandala I, et al. Encapsulation by nanoemulsions. In: Jafari M, editor. Nanoencapsulation technologies for the food and nutraceutical industries. Amsterdam: Elsevier; 2017. p. 36–73.
- Kumar DHL, Sarkar P. Encapsulation of bioactive compounds using nanoemulsions. Environ Chem Lett. 2018;16(1):59–70.
- Öztürk B. Nanoemulsions for food fortification with lipophilic vitamins: Production challenges, stability, and bioavailability. Eur J Lipid Sci Technol. 2017;119(7):1500539.
- Berton-Carabin CC, Ropers M, Genot C. Lipid oxidation in oil-in-water emulsions: involvement of the interfacial layer. Compr Rev Food Sci Food Saf. 2014;13(5):945–977.
- McClements DJ, Decker E. Interfacial antioxidants: a review of natural and synthetic emulsifiers and coemulsifiers that can inhibit lipid oxidation. J Agric Food Chem. 2018;66(1):20–35.
- Patel RB, Thakore SD, Patel MR. Recent survey on patents of nanoemulsions. Curr Drug Deliv. 2016;13(6):857–881.
- Lohani A, Verma A, Joshi H, et al. Nanotechnology-based cosmeceuticals. Int Sch Res Not. 2014;2014:1–14.
- Kaul S, Gulati N, Verma D, et al. Role of nanotechnology in cosmeceuticals: a review of recent advances. J Pharm. 2018;2018:1–19.
- Aziz ZAA, Mohd-Nasir H, Mohd Setapar SH, et al. Role of nanotechnology for design and development of cosmeceutical: application in makeup and skin care. Front Chem. 2019;7:739.