Abstract
Peel colour is an important economic trait in blueberries (Vaccinium spp.) and is determined by the composition and concentration of anthocyanins. Anthocyanin accumulation within the blueberry peel is significantly affected by light. In the present study, the response mechanism of blueberry anthocyanin synthesis to various light intensities was explored in an effort to identify candidate genes involved in anthocyanin content changes using transcriptomic analysis. The analysis results indicated that a total of 671 differential genes were found, including 53 transcription factors. Among the 16 gene families of the anthocyanin synthesis pathway, 428 genes have certain expression. A total of 6,004 transcription factors in blueberry were identified following the analysis, and divided into 58 transcription factor families of which the largest was the MYB family (510). Co-expression analysis of transcription factors and anthocyanin synthesis pathway genes revealed that 36 genes (16 families) in the anthocyanin synthesis pathway are co-expressed with 66 transcription factors. The quantitative real-time polymerase chain reaction analysis results were consistent with the transcriptomic results. Six of these genes likely play an important role in the anthocyanin synthesis pathway, and further evaluation of these candidate genes is necessary. This study provides a foundation for further research on the mechanism of light-induced anthocyanin synthesis in blueberry.
Supplemental data for this article is available online at https://doi.org/10.1080/13102818.2021.1924078 .
Introduction
Anthocyanins, one of the most important groups of phenolic compounds synthesized through the shikimate pathway, phenyl-propane pathway and flavonoid-anthocyanin pathway [Citation1]. They act as secondary metabolites for flower and fruit colouration, dispersal of seeds and resistance against abiotic and biotic factors [Citation2–4]. The flavonoid biosynthesis pathway is one of the critical branches for the synthesis of anthocyanin regulated by several structural and regulatory genes in many plant species [Citation5]. Recently, anthocyanin biosynthesis and regulation have been reported in many crop plants [Citation6, Citation7]. In the anthocyanin biosynthetic pathway, chalcone synthase (CHS) is the first determinant which collaborates with others, i.e. flavanone-3-hydroxylase (F3H), dihydro-flavonol reductase (DFR), chalcone isomerase (CHI), UDP-flavonoid glucosyltransferase (UFGT) and anthocyanidin synthase (ANS), to synthesize anthocyanin [Citation8–10]. The corresponding regulatory genes have been divided into early biosynthetic genes (EBGs) and late biosynthetic genes (LBGs). Early biosynthetic genes (EBGs) are involved in the production of flavonols, whereas the late biosynthetic genes (LBGs) are responsible for the production of anthocyanins in vegetative parts of pants [Citation11]. The transcription factors also involved in the regulation of anthocyanin synthesis are divided mainly into MYB, bHLH and WD40 families [Citation12]. In addition, several studies have shown that the transcription of anthocyanin related genes is also associated with genes such as NAC and ERF [Citation13, Citation14].
The biosynthesis of anthocyanin is affected by various environmental stresses, in which light is the crucial one[Citation15–17]. The plant photoreceptors perceive different light wavelengths through light-regulating anthocyanin gene via intra-cellular second messenger systems. The light dependent biosynthesis of anthocyanin involves three types of major photoreceptors such as blue or ultraviolet, red light sensing phytochromes and UV-B specific photo-receptor [Citation18–22]. Previously, the effect of light intensity in the development of anthocyanin has been reported in many plants such as strawberries (Fragaria ananassa) [Citation23], grapes (Vitis vinifera L.) [Citation24], lychee (Litchi chinensis Sonn.) [Citation25] and apples (Malus domestica L.) [Citation26, Citation27]. Shao et al. revealed that strawberries exposed to 25% and 75% light intensity showed less accumulation of anthocyanins, as compared to those exposed to 100% of light intensity [Citation23]. In addition, several candidate genes were identified such as HY5, UVR8, PHY, CRY and COL, which play a critical role in the response and transmission of light signals in grapes [Citation28]. Furthermore, multiple transcriptional genes, that is, 1MYB, 3bHLH, 2NAC and 1ERF, are involved in regulating the light-induced anthocyanin synthesis in grapes [Citation28]. However, the effect of light intensity in the biosynthesis of blueberry anthocyanin has not been elucidated.
Blueberry, Cyanococcus (Vaccinium; Ericaceae) is an economically important crop throughout the world, including China, with a planting area covering 31,210 hm2 [Citation29]. According to the United Nations Food and Agriculture Organization, it is called the ‘king of berries’ [Citation30]. Blueberry is rich in vitamin and nutritional value due to abundance of anthocyanins. It is also beneficial for health, being associated with improvements in eyesight, physical strength and immunity, and the berries also have anti-inflammatory, anti-ultraviolet, anti-infection, anti-aging and anti-cancer properties [Citation31–33]. The functional genes and transcription factors related to anthocyanin synthesis in blueberry fruits have been gradually isolated. Studies have shown that the expression of CHI (ORTHOMCL 7083) and DFR (ORTHOMCL 11867), as well as other genes involved in the process of fruit colour formation, can differ significantly, which indicates that different fruit colours are the result of several different pigments present in the fruits [Citation33]. Researchers have also shown that irradiating blueberry fruits with different light qualities can induce the expression of the VcMYBPA1 gene, thereby promoting the accumulation of anthocyanins [Citation34, Citation35], and Chau et al. showed that ultraviolet light irradiation significantly increased the accumulation of blueberry ‘Duke’ anthocyanins, especially UV-C [Citation36]. While the functionality of light exposure has been shown in blueberries, the mechanism by which light intensity affects anthocyanin synthesis in blueberry fruits has yet to be fully elucidated. In order to address this knowledge gap, candidate genes associated with light-induced anthocyanin synthesis in blueberry fruits were identified in this study using the Rabbiteye blueberry variety ‘Pink Blue’ (Vaccinium ashei Reade).
Gaining a better understanding of anthocyanin biosynthesis-related gene expression is expected to inform cultivation methods in order to better regulate the synthesis of anthocyanins and improve blueberry fruit quality. Furthermore, it can provide novel insights into the synthesis and accumulation of fruit polyphenols. Therefore, understanding anthocyanin synthesis and identifying the candidate genes involved in the pathway are necessary for improving the quality and quantity of anthocyanins. The used genomics to accomplish these goals is accelerating the efforts to increase the commercial and medicinal values of blueberries.
Materials and methods
Plant materials
Three-year-old rabbiteye blueberry (Vaccinium ashei) were grown at Kaili, Guizhou, China, with planting distance of 30 × 30 cm. It was located at 107°61 East longitude and 26°52 North latitude. The light treatment started in May 2019 and the fruits were harvested in July 2019. For this purpose, multiple shading nets and illuminance metre (Shenzhen Jumaoyuan Technology Co., Ltd.) were used to measure different levels of shading and intensity of light, respectively. The fruit samples were collected from thirty plants under different light treatments including: natural light (intensity 100%: T), moderate shading (intensity 50%: F) and severe shading (intensity 20%; Q). All harvested fruit samples were frozen immediately in liquid nitrogen and kept at −80 °C for subsequent molecular experiments. A portion of harvested fruits were used for determination of anthocyanin contents and soluble sugar contents.
Determination of anthocyanin and soluble sugar content
Anthocyanin content was determined using a pH differential method [Citation37], and soluble sugar content was determined using an anthrone assay [Citation38].
RNA extraction and library construction
RNA was isolated from three blueberry fruits in each treatment from three biological replicates by using Plant RNAout (TIANDZ, Beijing, China) in accordance with the manufacturer’s protocol. The purity was assayed using Nano Photometer spectrophotometer (IMPLEN, CA, USA). The RNA concentration was measured using the Qubit RNA analysis kit (Life Technologies, California, USA) with a Qubit 2.0 fluorometer. The RNA integrity was assessed using a Bioanalyzer 2100 system (Agilent Technologies, CA, USA). A total of 3 μg amount of RNA was used for each sample to generate a library using NEBNext (New England Biolabs, Ipswich, MA, USA). Nine libraries were produced and sequenced using an Illumina HiSeq platform (Illumina, CA, USA) to generate 125–150 bp paired-end reads. The sequence data were extracted using a custom Perl script with a base pair quality of Q ≥ 20.
Transcriptome sequencing and enrichment analysis
Alignments were made with highbush blueberry (V. corymbosum) reference genome (http://gigadb.org/dataset/100537) [Citation39], using HISAT2 software to map the filtered reads for reference genome [Citation40]. Fragments Per Kilobase of transcript per Million fragments mapped reads (FPKM) was used as an indicator of transcription or gene expression levels. DESeq2 was used to identify any differentially expressed genes (DEGs) [Citation41]. The filter conditions were |log2 (fold change)| ≥ 1, and the false discovery rate (FDR) was < 0.05. Gene Ontology (GO) and Kyoto Encyclopedia of Genes and Genomes (KEGG) enrichment analyses of the DEGs were implemented using the Clusterprofiler R software packages (using a corrected P-value < 0.05) [Citation42]. The online database PlantTFDB was used to identify transcription factors [Citation43]. The co-expression of the anthocyanin synthesis pathway and transcription factor genes was visualized using Cytoscape [Citation44]. According to the analysis of plant anthocyanin pathway by Ye et al., the blueberry anthocyanin synthesis pathway was identified by blast, and the E-value was e−5 [Citation45].
Real-time quantitative polymerase chain reaction (RT-qPCR) analysis
The cDNA was synthesized and RT-qPCR analysis was carried out to validate the transcriptomic analysis results [Citation46]. A bilberry GAPDH (AY123769) and ACT7 genes were selected as internal controls. RT-qPCR primers were designed using Primer Premier 5 software [(PREMIER Biosoft International) (Supplemental Table S1)] [Citation47, Citation48]. Three biological replicates were used for all samples. The relative expression level was calculated using the 2−ΔΔCT method [Citation49].
Table 1. Transcriptome sequencing data statistics for each of the biological replicates under the three different light transmittance treatments (T, F and Q).
Data analysis
MS Excel 2010 (Microsoft, Redmond, WA, USA) and SPSS v25 (IBM, Armonk, NY, USA) software were used for statistical data analysis. The significant differences were assessed by Duncan’s new double range at p ≤ 0.05.
Results
Effect of light intensity on contents of anthocyanin, soluble sugar and titratable acid of blueberry fruit
As light transmittance increased, the anthocyanins content also gradually increased, and the blueberry fruit became darker in colour. At 100% light transmittance (T), the anthocyanin content of the blueberry fruits increased by 3.55% and 288.89% compared with a light transmittance of 50% (F) and 20% (Q), respectively (). The soluble sugar content at T was higher compared to 47.04% F and 48.78% Q (). The percent titratable acids of T, F and Q were 0.40%, 0.30% and 1.00%, respectively. Our results showed that at a higher light intensity, pigment contents and soluble sugars were increased, whereas the titratable acid content was significantly decreased ().
Transcriptome sequencing
Nine blueberry samples were sequenced for cDNA libraries construction. The low-quality data were removed to obtain clean reads (). There were 45 M + sample raw data reads, and filtering out any invalid data did not significantly reduce the number of clean reads, indicating that the reads were of high quality. The GC content of the libraries was about 46%, the Q20 ratio was at least 96%, and the Q30 ratio was 91%.
Differential gene enrichment analysis
The effects of the three light treatments (T, F and Q) on the transcriptome of the blueberry fruits were compared. shows that a total of 1,176 genes were up-regulated in the T treatment compared to the F treatment (TF), and a total of 7,715 genes were up-regulated in the T treatment compared to the Q treatment (TQ). A total of 671 common genes were identified between the TF and TQ treatments. While both Q and F constituted lower light intensity treatments compared to T, the number of differentially up-regulated genes was much higher in TQ compared to TF, likely because of the significant differences in light intensity (Supplemental Table S2; ).
Figure 2. Enrichment analysis results of (A) differentially transcribed genes displayed as a Venn diagram representing the intersection of DEGs between the T and F treatments (T_VS_F) and the T and Q treatments (T_VS_Q); and (B) statistical analysis of differential gene expression using KEGG enrichment.
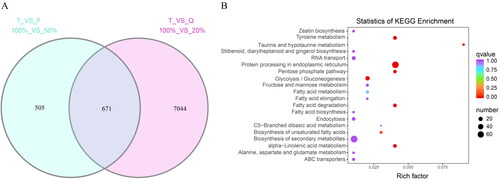
Following KEGG enrichment (Supplemental Table S3), 265 of the 671 DEGs were found to be enriched in 78 pathways, including metabolic pathways (ko01100, 105), biosynthetic pathways of secondary metabolites (ko01110, 72), endoplasmic reticulum protein processing (ko04141, 67), plant pathogen interaction (ko04626, 22) and glycolysis/gluconeogenesis (ko00010, 21).
Concurrently, GO enrichment analysis was performed on the 671 genes (Supplemental Table S4), and 433 were found to be enriched in 1062 processes. Of these, 409 genes were enriched in 730 biological processes, including response to high light intensity (GO: 0009644, 23), response to light intensity (GO: 0009642, 23), response to chitin (GO: 0010200, 22), inorganic ion transmembrane transport (GO: 0098660, 17), response to hydrogen peroxide (GO: 0042542, 15), drug transmembrane transport (GO: 0006855, 15), inorganic anion transport (GO: 0015698, 15), drug transport (GO: 0015893, 15), nicotinamide nucleotide metabolism process (GO: 0046496, 15), pyridine nucleotide metabolism process (GO: 0019362, 15), pyridine-containing compounds metabolic process (GO: 0072524, 15) and redox coenzyme metabolic process (GO: 0006733, 15), indicating that blueberry anthocyanin synthesis is enriched in the light response pathway.
With regards to cell composition, there were 433 enriched genes included in 112 cell composition annotations, including extracellular space (GO: 0005615, 14), extracellular region part (GO: 0044421, 14), chaperone complex (GO: 001031, 7), chloroplast-like nucleus (GO: 0042644, 7), plastid-like nucleus (GO: 0042646, 7), nucleus-like (GO: 0009295, 7) and membrane side (GO: 0098552, 7), validating that under different light intensities, blueberry gene activity varies.
Among the molecular functions, there were 389 genes enriched in 220 molecular functions, including sulfate transmembrane transporter activity (GO: 0015116, 13), alcohol dehydrogenase (NAD) activity (GO: 0004022, 13), sulfur compound transmembrane transporter activity (GO: 1901682, 13), inorganic anion transmembrane transporter activity (GO: 0015103, 13), thiosulfate transmembrane transporter activity (GO: 0015117, 11), succinic acid membrane transporter activity (GO: 0015141, 11), oxidative phosphorylation uncoupling agent activity (GO: 0017077, 11), oxaloacetate transmembrane transporter activity (GO: 0015131,11), malate transmembrane transporter activity (GO: 0015140, 11), C4-dicarboxylic acid transmembrane transporter activity (GO: 0015556, 11) and dicarboxylic acid transmembrane transporter activity (GO: 0005310, 11) (), which illustrates that different light treatments alter protein expression activity in blueberry. During the GO enrichment process, the blueberry anthocyanin synthesis DEGs were most enriched within the high-light intensity and light intensity responses.
Blueberry anthocyanin synthesis pathway-related genes
Transcriptome analysis was performed to identify genes related to the anthocyanin synthesis pathway in blueberries under the three different light conditions (Supplemental Table S5; ). There are 36 genes (16 gene families) in the anthocyanin synthesis pathway that are highly expressed under full light.
Figure 4. Genes involved in the anthocyanin accumulation pathway and the gene expression pattern of light-regulated anthocyanin synthesis are shown. Heatmaps show gene expression levels from left to right for light transmittance values of 20%, 50% and 100%, respectively. Colour changes from blue to red indicate a gradual increase in gene expression. (A) Anthocyanin accumulation pathways and expression patterns of various structural genes. (B) Pattern diagram of light regulation of anthocyanin synthesis. A solid line indicates that the mechanism of action is known, and a dashed line indicates that the mechanism of action is unknown. (C) qRT-PCR analysis of candidate genes related to light-induced anthocyanin biosynthesis, where orange represents FPKM and green qPCR.
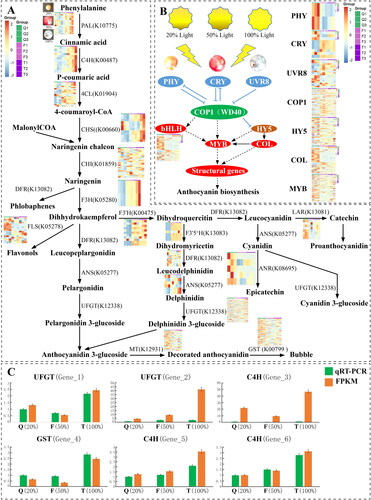
At the beginning of the anthocyanin synthesis pathway, the PAL gene plays an important role, and based on the PAL heatmap, it was up-regulated at 100% (T) transmittance, but not in the F or Q treatments. Other genes that were up-regulated at 100% transmittance (T) but hardly expressed in the F and Q treatments included C4H, F3H and F3′5′H, suggesting that these genes are associated with the anthocyanin synthesis pathway.
Based on the research results of Sun et al. [Citation28] and the findings reported herein (), blueberries may form a complete light-responsive system under light induction. The proposed mechanism would function with the light response factor being activated first, then the triplet complex would be further regulated, and finally the internal anthocyanin formation would be regulated (Supplemental Table S6; ). In the first light response stage, PHY, CRY and UVR8 would function as plant light sensing devices, which were all expressed to different degrees in the different samples. At the same time, MYB, bHLH and WD40 could form a complex triplet, which is capable of regulating the cis-element of the target gene, thereby controlling its expression and affecting anthocyanin content.
At the proposed step where COP1 becomes involved in the regulation process, gene expression gradually increased in this experiment. This suggests that the light-induced response suppressed the expression of downstream genes. The expression of COP1 in the T treatment was less than that in Q or F, indicating that the gene’s inhibitory effect on downstream genes is likely gradually reduced, which results in higher anthocyanin content in T than Q or F. After being regulated by COP1, multiple genes downstream of MYB, HY5, COL, bHLH and WD40 were up-regulated. The increased expression was positively correlated with light intensity, and the expression of HY5, COL, MYB and bHLH during this period indicates that, in terms of co-regulated genes subsequent to the COP1 gene, gene expression in the regulatory pathway tends to be consistent over the same period. These findings are consistent with what is known about the regulation of key genes involved in anthocyanin production. The light-induced blueberry anthocyanin synthesis pathway, through the joint regulation of light response factors, inhibitory factors and the triple complex, is regulated in response to different light conditions that either promote or inhibit anthocyanin production.
Identification and co-expression of blueberry transcription factors
A total 58 transcription factor families containing 6,004 transcription factors were identified within the blueberry genome (Supplemental Table S7). Using related genes and transcription factors in the anthocyanin synthesis pathway for co-expression (R > 0.9), a total of 36 genes co-expressed with 66 transcription factors were identified in the anthocyanin synthesis pathway (Supplemental Table S8; ). These same 36 genes were also highly expressed under the T (100%) treatment (). Based on this current research, FLS, F3′5′H and GST likely play a key role in the anthocyanin synthesis pathway.
RT-qPCR validation
Transcriptome DEGs were selected for quantitative fluorescence spectroscopy analysis to validate the transcriptomic analysis results. The blueberry GAPDH and ACT7 were selected as an internal reference, and six candidate genes were selected for analysis. Based on the qRT-PCR results (), the amplification curves of the six genes were smooth and showed a single peak. Expression gradually increased as light intensity increased, which was consistent with the transcription results (R > 0.9). Among them, Gene_2, Gene_5 and Gene_6 showed a continuous upward trend with increasing light intensity, while Gene_1 was up-regulated during the T treatment. These findings validate the results of the transcriptomic analysis.
Discussion
Studies have shown that light intensity can increase the anthocyanin content in fruit, and the effect of shading on anthocyanin content in fruit is negative [Citation24, Citation26, Citation27]. It has also been shown that strong light (100% transmittance) inhibits the accumulation of anthocyanins, such as the biosynthesis of anthocyanins in petunia (Petunia × atkinsiana), which is hardly affected by intense light [Citation50]. Other studies suggest that the response to light intensity is highly variety-specific with regards to anthocyanin accumulation and structural gene expression. For instance, anthocyanin and flavanol accumulation in the grape variety ‘Cabernet Sauvignon’ is significantly induced by light [Citation51], whereas the induction of anthocyanin accumulation in the grape variety ‘Shiraz’ by light is not significant [Citation52]. By integrating our knowledge of genetics with plant breeding using hybridization or biotechnological methods, high-quality varieties that combine multiple desirable traits can be formed.
Varietal and geographical differences also account for some of the variation in anthocyanin content. Environmental differences in light, temperature and humidity occur between the north and the south in China, and these are major factors influencing the production of high-value crops such as grapes, apples and blueberries [Citation53]. This study used natural sunlight in conjunction with shade cloth to expose blueberries to different light intensities, which resulted in obvious differences in blueberry fruit phenotypes and fruit quality. At ripening, the blueberry peels of the shaded treatments showed obviously reduced pigment accumulation, clearly illustrating that different light intensities affect peel colouring. The experiment also found that at 100% full light, the anthocyanin content and soluble sugars in the blueberries were evidently higher compared to the other two treatments, further indicating that full-light exposure improves the yield and quality of blueberries.
Studies have shown that the synthesis of anthocyanins is carried out through the flavonoid pathway [Citation3], among them, the enzymes that catalyse the reaction of the anthocyanin synthesis pathway and the genes encoding these enzymes have been identified [Citation54]. But the expression patterns of different genes encoding enzymes are different (). Gene expression analysis showed that the expression of key structural genes involved in anthocyanin biosynthesis was significantly reduced after shading (Supplemental Table S5 and ). This study also discovered that the expression of two F3′5′H genes (maker-VaccDscaff1-augustus-gene-56.30 and maker-VaccDscaff8-augustus-gene-360.23) significantly increased (Supplemental Table S5 and ). The increased expression may account for the higher anthocyanin content in the fruits under full light. However, whether this candidate gene is the functional gene that affects anthocyanin synthesis under differing light environments needs to be determined.
Previous studies have shown that GST encodes a protein that binds directly to anthocyanins and acts as an anthocyanin transporter [Citation55]. This aforementioned study found that, under different light conditions, the expression level of GST increased with increased light transmittance, indicating that continuous anthocyanin accumulation may be promoted by the increased expression of GST. Furthermore, studies have found that under light induction, cis-acting elements may interact with trans-acting factors to activate gene expression. Cominelli et al. [Citation56] found that under white light conditions, PAP1 (MYB-type transcription factors) and TT8 (bHLH-type transcription factor) expression levels are enhanced with the extension of the light exposure period [Citation56]. In addition, the gene expression levels of key enzymes involved in anthocyanin synthesis, such as CHS, F3H and DFR, correspondingly increased the functional elements, which can regulate gene expression levels. In this study, ACE cis-acting elements were also found in candidate genes (). Moreover, both CHS and F3H were up-regulated under 100% light transmittance, whereas no significant up-regulation was detected in the other treatments. Given these findings, these two genes are proposed as candidate genes in light-induced anthocyanin accumulation in blueberry.
The three major transcription factors in the anthocyanin synthesis pathway, WD40, bHLH and MYB, form a complex to regulate anthocyanin production. Among them, bHLH and MYB combine with DNA to regulate the expression of target genes, and studies of WD40 have shown that when it is knocked out it can lead to the inhibition of anthocyanin synthesis [Citation57]. Similarly, MBW (MYB, bHLH and WD40) forms a trimeric complex with cis-elements in the same gene, which can be transcriptionally regulated to promote or inhibit gene expression. In this study, MYB, bHLH and WD40 were analysed in order to predict any regulatory pathways involved in anthocyanin synthesis, and any cis-functions of the candidate genes, UFGT, C4H and GST, associated with the anthocyanin synthesis pathway after the formation of a trimeric complex were deduced. The combination of these elements promotes the formation of blueberry anthocyanins in response to light. This study also found a series of light response and transmission factors, including COL, COP1, PHY, CRY, UVR8 and HY5, that affect light-induced anthocyanin accumulation as well as elements, such as ERF, HD-ZIP, MYB and NAC, that affect the synthesis of anthocyanins. The specific impact of these candidate genes has yet to be thoroughly explored.
Conclusions
This study found that blueberry anthocyanin content increased with the increase of light intensity. Transcriptome data showed that a total of 671 differentially expressed genes were found, including 53 transcription factors. There are 428 genes (16 gene families) involved in blueberry anthocyanin synthesis pathway. It was found that 36 genes (16 gene families) in the anthocyanin synthesis pathway were co-expressed with 66 transcription factors. Following extensive analyses, six candidate genes associated with the mechanism for response to light intensity in anthocyanin production in blueberry were proposed. Although further evaluation of these candidate genes is necessary, including functional studies, these findings are foundational for further research on the mechanism of light-induced anthocyanin synthesis in blueberry.
Disclosure statement
The authors declare no conflict of interest.
Data availability statement
The authors confirm that the data supporting the findings of this study are available within NCBI (PRJNA705534).
References
- Akagi T, Ikegami A, Tsujimoto T, et al. DkMyb4 is a Myb transcription factor involved in proanthocyanidin biosynthesis in persimmon fruit. Plant Physiol. 2009;151(4):2028–2045.
- Xie XB, Li S, Zhang RF, et al. The bHLH transcription factor MdbHLH3 promotes anthocyanin accumulation and fruit colouration in response to low temperature in apples. Plant Cell Environ. 2012;35:1884–1897.
- Kovinich N, Kayanja G, Chanoca A, et al. Abiotic stresses induce different localizations of anthocyanins in Arabidopsis. Plant Signal Behav. 2015;10:e1027850.
- Hu DG, Sun CH, Ma QJ, et al. MdMYB1 regulates anthocyanin and malate accumulation by directly facilitating their transport into vacuoles in apples. Plant Physiol. 2016;170:1315–1330.
- Holton TA, Cornish EC. Genetics and biochemistry of anthocyanin biosynthesis. Plant Cell. 1995;7:1071–1083.
- Jaakola L. New insights into the regulation of anthocyanin biosynthesis in fruits. Trends Plant Sci. 2013;18(9):477–483.
- Wang Y, Wang Y, Song Z, et al. Repression of MYBL2 by both microRNA858a and HY5 leads to the activation of anthocyanin biosynthetic pathway in Arabidopsis. Mol Plant. 2016;9(10):1395–1405.
- Zhou B, Wang Y, Zhan Y, et al. Chalcone synthase family genes have redundant roles in anthocyanin biosynthesis and in response to blue/UV-A light in turnip (Brassica rapa; Brassicaceae). Am J Bot. 2013;100:2458–2467.
- Petroni K, Tonelli C. Recent advances on the regulation of anthocyanin synthesis in reproductive organs. Plant Sci. 2011;181:219–229.
- Xu W, Grain D, Bobet S, et al. Complexity and robustness of the flavonoid transcriptional regulatory network revealed by comprehensive analyses of MYB-bHLH-WDR complexes and their targets in Arabidopsis seed. New Phytol. 2014;202(1):132–144.
- Stracke R, Jahns O, Keck M, et al. Analysis of production of flavonol glycosides-dependent flavonol glycoside accumulation in Arabidopsis thaliana plants reveals MYB11-, MYB12- and MYB111-independent flavonol glycoside accumulation. New Phytol. 2010;188:985–1000.
- Gonzalez A, Zhao M, Leavitt JM, et al. Regulation of the anthocyanin biosynthetic pathway by the TTG1/bHLH/MYB transcriptional complex in Arabidopsis seedlings. Plant J. 2008;53:814–827.
- Zhou H, Lin-Wang K, Wang H, et al. Molecular genetics of blood-fleshed peach reveals activation of anthocyanin biosynthesis by NAC transcription factors. Plant J. 2015;82(1):105–121.
- Koyama T, Sato F. The function of ETHYLENE RESPONSE FACTOR genes in the light-induced anthocyanin production of Arabidopsis thaliana leaves. Plant Biotechnol (Tokyo). 2018;35(1):87–91.
- Zhang HN, Li WC, Wang HC, et al. Transcriptome profiling of light-regulated anthocyanin biosynthesis in the pericarp of litchi. Front Plant Sci. 2016;7:963.
- Bai S, Sun Y, Qian M, et al. Transcriptome analysis of bagging-treated red Chinese sand pear peels reveals light-responsive pathway functions in anthocyanin accumulation. Sci Rep. 2017;7:63.
- Jiang M, Ren L, Lian H, et al. Novel insight into the mechanism underlying light-controlled anthocyanin accumulation in eggplant (Solanum melongena L.). Plant Sci. 2016;249:46–58.
- Ahmad M, Cashmore AR. The blue-light receptor cryptochrome 1 shows functional dependence on phytochrome A or phytochrome B in Arabidopsis thaliana. Plant J. 1997;11:421–427.
- Meng LS, Liu A. Light signaling induces anthocyanin biosynthesis via AN3 mediated COP1 expression. Plant Signal Behav. 2015;10:e1001223.
- Wade HK, Bibikova TN, Valentine WJ, et al. Interactions within a network of phytochrome, cryptochrome and UV-B phototransduction pathways regulate chalcone synthase gene expression in Arabidopsis leaf tissue. Plant J. 2001;25:675–685.
- Chatterjee M, Sharma P, Khurana JP. Cryptochrome 1 from Brassica napus is up-regulated by blue light and controls hypocotyl/stem growth and anthocyanin accumulation. Plant Physiol. 2006;141:61–74.
- Su N, Lu Y, Wu Q, et al. UV-B-induced anthocyanin accumulation in hypocotyls of radish sprouts continues in the dark after irradiation. J Sci Food Agric. 2016;96:886–892.
- Shao WL, Li YL, Gao S, et al. Effects of light intensity on the fruit coloration and anthocyanian biosynthesis in Fragaria × ananassa Duch. ‘Benihoppe’ and the possible molecular mechanism. Bull Bot Res. 2018;38(5):661–668.
- Azuma A, Yakushiji H, Koshita Y, et al. Flavonoid biosynthesis-related genes in grape skin are differentially regulated by temperature and light conditions. Planta. 2012;236(4):1067–1080.
- Zhang HN, Li WC, Wang HC, et al. Transcriptome profiling of light-regulated anthocyanin biosynthesis in the pericarp of litchi. Front Plant Sci. 2016;7:963.
- Takos AM, Jaffé FW, Jacob SR, et al. Light-induced expression of a MYB gene regulates anthocyanin biosynthesis in red apples. Plant Physiol. 2006;142(3):1216–1232.
- Feng F, Li M, Ma F, et al. The effects of bagging and debagging on external fruit quality, metabolites, and the expression of anthocyanin biosynthetic genes in ‘Jonagold’ apple (Malus domestica Borkh.). Sci Hortic (Amsterdam). 2014;165:123–131.
- Sun L, Li S, Tang X, et al. Transcriptome analysis reveal the putative genes involved in light-induced anthocyanin accumulation in grape ‘Red Globe’ (V. vinifera L.). Gene. 2020;728:144284.
- Li Y, Pei J, Sun H. Status and prospect of global blueberry industry. J Jilin Agric Univ. 2018;40(4):421–432.
- Kaspar KL, Park JS, R. Brown C, et al. Sensory evaluation of pigmented flesh potatoes (Solanum tuberosum L.). FNS. 2013;04(01):77–81.
- Cassidy A, Mukamal KJ, Liu L, et al. High anthocyanin intake is associated with a reduced risk of myocardial infarction in young and middle-aged women. Circulation. 2013;127(2):188–196.
- Ancillotti C, Ciofi L, Pucci D, et al. Polyphenolic profiles and antioxidant and antiradical activity of Italian berries from Vaccinium myrtillus L. and Vaccinium uliginosum L. subsp. gaultherioides (Bigelow) S.B. Young. Food Chem. 2016;204:176–184.
- Lin Y, Wang Y, Li B, et al. Comparative transcriptome analysis of genes involved in anthocyanin synthesis in blueberry. Plant Physiol Biochem. 2018;127:561–572.
- González-Villagra J, Marjorie RD, Alberdi M, et al. Solar UV irradiation effects on photosynthetic performance, biochemical markers, and gene expression in highbush blueberry (Vaccinium corymbosum L.) cultivars. Sci Hortic (Amsterdam). 2020;259:108816.
- Inostroza-Blancheteau C, Reyes-Díaz M, Arellano A, et al. Effects of UV-B radiation on anatomical characteristics, phenolic compounds and gene expression of the phenylpropanoid pathway in highbush blueberry leaves. Plant Physiol Biochem. 2014;85:85–95.
- Chau TN, Kim J, Yoo KS, et al. Effect of prestorage UV-A, -B, and -C radiation on fruit quality and anthocyanin of ‘Duke’ blueberries during cold storage. J Agr Food Chem. 2014;62(50):12144–12151.
- Sun J, Liu Y, Zhao Y, et al. Optimization of analytical condition of determining anthocyanins content in blueberry wine by pH-differential method. China Brew. 2011;30(11):171–174.
- Wei J, Wu CY, Jiang Y, et al. Sample preparation optimization for 493 determination of soluble sugar in red jujube fruits by anthrone method. Food Sci. 2014;35(24):136–140.
- Colle M, Leisner CP, Wai CM, et al. Haplotype-phased genome and evolution of phytonutrient pathways of tetraploid blueberry. GigaScience. 2019;8(3):giz012.
- Kim D, Paggi JM, Park C, et al. Graph-based genome alignment and genotyping with HISAT2 and HISAT-genotype. Nat Biotechnol. 2019;37(8):907–915.
- Love M, Ahlmann-Eltze C, Anders S, et al. DESeq2: Differential gene expression analysis based on the negative binomial distribution [Internet]. 2014 [cited 2020 Sep 15]. Available from: https://rdrr.io/bioc/DESeq2/.
- Yu G, Wang LG, Han Y, et al. clusterProfiler: an R package for comparing biological themes among gene clusters. Omics: J Integrative Biol. 2012;16(5):284–287.
- Jin J, Tian F, Yang DC, et al. PlantTFDB 4.0: toward a central hub for transcription factors and regulatory interactions in plants. Nucleic Acids Res. 2017;45(D1):D1040–D1045.
- Otasek D, Morris JH, Bouças J, et al. Cytoscape automation: empowering workflow-based network analysis. Genome Biol. 2019;20:185.
- Ye JB, Wang GY, Tan JP, et al. Identification of candidate genes involved in anthocyanin accumulation using Illmuina-based RNA-seq in peach skin. Scientia Horti. 2019;250:184–198.
- Yao WJ, Li CZ, Lin SY, et al. Morphological characteristics and transcriptome comparisons of the Shoot Buds from flowering and non-flowering Pleioblastus pygmaeus. Forests. 2020;11(11):1229.
- Wang Y, Yang R, Zheng J, et al. Exogenous foliar application of fulvic acid alleviate cadmium toxicity in lettuce (Lactuca sativa L.). Ecotoxicol Environ Saf. 2019;167:10–19.
- Vashisth T, Johnson LK, Malladi A. An efficient RNA isolation procedure and identification of reference genes for normalization of gene expression in blueberry. Plant Cell Rep. 2011;30:2167–2176.
- Livak KJ, Schmittgen TD. Analysis of relative gene expression data using real-time quantitative PCR and the 2 − ΔΔCT method. Methods. 2001;25(4):402–408.
- Weiss D, Halevy AH. The role of light reactions in the regulation of anthocyanin synthesis in Petunia corollas. Physiol Plant. 1991;81(1):127–133.
- Matus JT, Loyola R, Vega A, et al. Post-veraison sunlight exposure induces MYB-mediated transcriptional regulation of anthocyanin and flavonol synthesis in berry skins of Vitis vinifera. J Exp Bot. 2009;60(3):853–867.
- Downey MO, Harvey JS, Robinson SP. The effect of bunch shading on berry development and flavonoid accumulation in Shiraz grapes. Aust J Grape Wine Res. 2008;10(1):55–73.
- Wang H, Li MF, Yang Y, et al. Recent advances on the molecular mechanisms of anthocyanin synthesis in fruits. Plant Physiol J. 2015;51(1):29–43.
- Tanaka Y, Ohmiya A. Seeing is believing: engineering anthocyanin and carotenoid biosynthetic pathways. Curr Opin Biotechnol. 2008;19(2):190–197.
- Mueller LA, Goodman CD, Silady RA, et al. AN9, a petunia glutathione S-transferase required for anthocyanin sequestration, is a flavonoid-binding protein. Plant Physiol. 2000;123(4):1561–1570.
- Cominelli E, Gusmaroli G, Allegra D, et al. Expression analysis of anthocyanin regulatory genes in response to different light qualities in Arabidopsis thaliana. J Plant Physiol. 2008;165(8):886–894.
- Carey CC, Strahle JT, Selinger DA, et al. Mutations in the pale aleurone color1 regulatory gene of the Zea mays anthocyanin pathway have distinct phenotypes relative to the functionally similar TRANSPARENT TESTA GLABRA1 gene in Arabidopsis thaliana. Plant Cell. 2004;16(2):450–464.