Abstract
Sanghuangporus baumii (Pilát) (Zhou and Dai), a traditional Chinese medicinal fungus, has been confirmed to produce triterpenoids, which possess a good anti-cancer effect. However, the triterpenoids yield of S. baumii is low and its biosynthetic mechanism is not clearly understood. To investigate the triterpenoids biosynthetic mechanism, the whole cDNA sequence of the HMG-CoA reductase (HMGR) gene was obtained. The open reading frame of HMGR gene cDNA named SbHMGR, comprised 4170 bp, encoding a polypeptide of 1389 amino acids with a predicted protein molecular weight of 147.53 kDa. The HMGR gene includes four exons and three introns. Subsequently, the triterpenoids content was determined at different developmental stages of S. baumii. It showed a dynamic tendency—increased first, then decreased and peaked on 14th day in the mycelium stage. In addition, the changing trend of SbHMGR enzyme activity and transcript level of SbHMGR gene were measured. They were nearly consistent with the triterpenoids content, suggesting that SbHMGR gene may play a key regulatory role in triterpenoids biosynthetic pathway. Similarly, changes in the transcript level of downstream key genes (SbMVD, SbIDI, SbLSD) of SbHMGR gene were also positively correlated with the triterpenoids content, indicating those three genes were also involved in triterpenoids biosynthetic pathway. Moreover, SbHMGR gene had been observed to be expressed in prokaryote. The results will be valuable for elucidation of the biosynthetic pathway of triterpenoids in S. baumii, lay a foundation for genetic modifications to improve triterpenoids yield.
Introduction
Sanghuangporus baumii (synonym Phellinus baumii, Inonotus baumii) is the fruiting body of a fungus that grows on the stem bark of Syringa reticulata (Bl.) Hara. It is widely shared that S. baumii has a high-efficiency anti-cancer effect and is internationally acknowledged as the first medicinal fungus in the field of biological cancer treatment, attracting a lot of attention in the last decade [Citation1–4]. It also has been shown to possess other effective biomedical activities, such as immunomodulatory [Citation5], antioxidant [Citation6], anti-inflammatory [Citation7] and against hepatotoxins [Citation8]. So far, many effective bioactive components have been isolated and purified from S. baumii, including triterpenoids, polysaccharides, flavonoids, polyphenols and organic acids. It is worth mentioning that the triterpenoids have been proven to possess major medicinal activities and triterpenoids content is an important index to evaluate the medicinal value of S. baumii [Citation9–11]. However, the yield of triterpenoids is naturally relatively low (12.61 mg/g dry weight) in S. baumii [Citation12], it is difficult to meet the demand for medicinal use. Therefore, a method to increase the yield of triterpenoids is urgently needed.
Currently, one way to solve this problem would be reliable, which is the manipulation of the key enzyme genes in the biosynthetic pathway of triterpenoids to promote their expression and increase the amount of the target products using genetic engineering techniques. In eukaryotes, triterpenoids are synthesized via the classic mevalonate (MVA) pathway, and 3-hydroxy-3-methylglutaryl Coenzyme A reductase (HMGR) is the first rate-limiting enzyme that catalyzes the conversion of 3-hydroxy-3-methylglutaryl Coenzyme A into mevalonate [Citation13–15]. Next, the mevalonate is catalyzed by several downstream key enzymes, including mevalonate pyrophosphate decarboxylase (MVD), isopentenyl diphosphate isomerase (IDI), lanosterol 14-α-demethylase (LSD), which are an important connection between primary metabolism and triterpenoids () [Citation16–18]. But the biosynthetic pathway of triterpenoids in fungi is still poorly understood, so the ascertainment of this specific biosynthetic pathway will be urgently needed for attempts to increase triterpenoids products.
Figure 1. The biosynthetic pathway of triterpenoids. HMGR, 3-Hydroxy-3-methylglutayl Coenzyme A reductase; MVD, mevalonate pyrophosphate decarboxylase; IDI, isopentenyl diphosphate isomerase; LSD, lanosterol 14-alpha-demethylase.
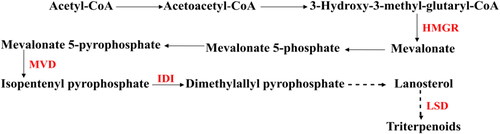
At present, many HMGR genes have been cloned, expressed and characterized, including Ganoderma lucidum [Citation19], Salvia miltiorrhiza [Citation20], Glycyrrhiza uralensis [Citation21]. Those results indicated that over-expression of HMGR gene can promote the biosynthesis of related downstream products. In Ganoderma lucidum, over-expression of the HMGR gene increases the triterpenoids yield, which suggested that HMGR enzyme reaction is a significant catalytic step in triterpenoids biosynthetic pathway [Citation22]. Although the HMGR gene function is significant, it has not been yet fully characterized. For example, it has not been confirmed whether the yield of triterpenoids is related to HMGR enzyme activity. Thus, it is pivotal to research the detailed enzyme activity and transcript level of SbHMGR gene at different developmental stages, which are associated with the triterpenoids yield in S. baumii.
In the present work, the structure of the SbHMGR gene cDNA and DNA were analyzed and described from S. baumii with bioinformatics analysis software for the first time. Subsequently, the relative relationship between the SbHMGR enzyme activity and triterpenoids yield were explored while the transcript level of SbHMGR gene, and downstream key genes SbMVD, SbIDI, SbLSD were also investigated at different developmental stages. Moreover, over-expression of SbHMGR gene was realised and recombinant protein was observed in Escherichia coli BL21 (DE3). Our study could provide a reference for better reconstructing the triterpenoids biosynthetic pathway and improving the yield of triterpenoids in S. baumii.
Materials and methods
Strain, plasmids and culture conditions
S. baumii strain DL101 was preserved in the protection laboratory of the Northeast Forestry University, which was identified by ITS method and submitted to the NCBI (NCBI GenBank No.KP974834). The E. coli strain Top10 and BL21(DE3) were purchased from biology company (Tiangen, Beijing, China). The vector pET-32a and pMD18-T were obtained from Takara Biotechnology (Takara, Dalian, China). The mycelia of S. baumii were inoculated into 500 mL Erlenmeyer flasks containing potato dextrose (PD) medium (200 g potato, 20 g glucose, 1 L H2O) and grown in a shaking incubator at 170 rpm and 25 °C for 6, 8, 10, 12 and 14 days [Citation23]. The primordia and fruiting body of S. baumii were maintained on bags filled with substrate (77% sawdust, 20% wheat bran, 2% corn flour, 0.5% gypsum and lime, based on the dry weight of ingredients).
The SbHMGR gene cDNA and DNA synthesis
The total RNA of S. baumii was extracted using an RNAprep pure Plant Kit (Tiangen). Reverse-transcribed using a PrimeScript TM 1st strand cDNA synthesis kit (Takara). The DNA of S. baumii was extracted using the CTAB method [Citation24].The SbHMGR gene cDNA was amplified using primers SbHMGR-F1 and SbHMGR-R1, the SbHMGR gene DNA was amplified using primers SbHMGR-F2 and SbHMGR-R2 (). The PCR reactions were carried out under the following conditions: 94 °C for 5 min, followed by 35 cycles of 94 °C for 30 s, 65 °C for 35 s and 72 °C for 1 min 30 s, and a final extension at 72 °C for 10 min. The amplified cDNA fragments were ligated into the vector pET-32a (linearized using EcoR I and Hind III restriction sites) to obtain the pET-32a-SbHMGR vector using an In-Fusion HD Kit (Takara). The pET-32a-SbHMGR vector was transformed into E. coli Top10 and confirmed. The amplified DNA fragments were integrated into the pMD18-T vector and confirmed (TsingKe). All the primers synthesis and products sequencing were done by TsingKe (Harbin, China).
Table 1. Primers used in this research.
Bioinformatic analysis of the SbHMGR protein
The whole SbHMGR gene DNA and cDNA were analyzed for exons and introns in detail (http://gsds.cbi.pku.edu.cn/). The molecular weight, theoretical pI, amino-acid composition, the instability index (II) and grand average of hydropathicity (GRAVY) of SbHMGR gene cDNA were estimated with ProtParam (http://www.expasy.ch/tools/ protparam. html). The signal peptide was achieved by SignalP software (http://www.cbs. dtu.dk/services/SignalP/). The transmembrane regions were predicted using TMHMM Server (http://www.cbs.dtu.dk/services/TMHMM/). The three-dimensional structure of the SbHMGR protein was predicted using Swiss model (http://www.swissmodel.expasy.org/) [Citation25]. The HMGR protein sequences of 22 species deposited in the NCBI GenBank database were selected for alignment with SbHMGR protein. Using MEGA 6.0 software for HMGR protein sequences alignment, a distance tree was constructed with the Neighbor-joining method. The larger branch support values, the more reliable it was.
Measurement of SbHMGR enzyme activity and triterpenoids content
The SbHMGR enzyme activity was detected (including mycelia at 6, 8, 10, 12 and 14 days, primordia and fruiting body) using fungus HMG-CoA reductase (HMGR) ELISA Kit following the manufacturer’s directions (mlbio, Shanghai, China). To extract the triterpenoids from S. baumii, the materials were dried at 45 °C to a constant weight. 0.1 g dried materials were accurately weighed, and then ultrasonic extraction was conducted for 30 min after adding 2 mL 60% ethanol. 0.1 mL supernatant was extracted after centrifugation and dried at 70 °C for 20 min. Subsequently, 200 μL 5% vanillin-acetic acid and glacial acetic acid, 800 μL perchloric acid, and 3.9 mL ethyl acetate were added. Finally, the absorbance of triterpenoids was measured at 551 nm, and the triterpenoids content was calculated by the standard curve [Citation26,Citation27]. All data were the mean values of three biological sample measurements, including the determination of SbHMGR enzyme activity, triterpenoids content and the transcript level of genes. Error bars in the figures expressed the standard deviation (±SD).
Measurement of the SbHMGR gene and downstream genes expression by quantitative real time PCR
The total RNA of S. baumii was extracted as described previously, then it was reverse-transcribed using a PrimeScriptRT reagent Kit (Takara). The SbMVD, SbIDI and SbLSD1sequences were preliminary cloned and deposited in NCBI GenBank under numbers MK977617, MK955885 and MN640689, respectively. The primers of SbHMGR, SbMVD, SbIDI, SbLSD1 and α-tubulin genes were designed () for quantitative real time PCR (qRT-PCR). PCR reactions were carried out using the SYBR Premix Ex TaqTM II (Takara) with the Mx3000P Sequence Detection System (Agilent Technologies, Santa Clara, CA, USA). After initial denaturation at 95 °C for 3 min, amplification occurred in three steps: 30 s of denaturation at 95 °C, 30 s of annealing at 58 °C and 1 min of extension at 72 °C, for a total of 40 cycles. A total of seven developmental stages of S. baumii were estimated for the gene transcript level, including 6, 8, 10, 12 and 14 days, primordia and fruiting body, and all samples had three biological replicates. The hyphae harvested at 6th day were defined as the reference sample and the gene expression was set as 1.0, against which the data for the others were compared. The transcript level calculations were performed using the 2–ΔΔCt method described by Livak and Schmittgen [Citation28].
Heterologous expression of SbHMGR gene in E. coli
The plasmid pET-32a-SbHMGR was extracted from E. coli Top10, and then transformed into E. coli BL21(DE3) to obtain a recombinant expression strain. The positive clones were screened by PCR using the primers pET32a-F and pET32a-R (). A positive individual bacterial colony was transferred into 50 mL of Luria–Bertani (LB) medium containing 100 μg/mL ampicillin and cultured in a rotary shaker incubator (180 rpm) for 12 h at 37 °C. Next, 100 μL culture was inoculated into 50 mL of fresh LB medium with 100 μg/mL ampicillin and incubated at 37 °C and 180 rpm until the optical density at 600 nm (OD600) reached 0.6–0.8. Then, 1 mmol/L isopropyl β-d-1-thiogalactopyranoside (IPTG) was added to the culture medium to induce recombinant SbHMGR gene expression at 28 °C and 180 rpm for 2, 4 and 6 h. The E. coli cultures for various times were centrifuged at 10,000 g for 10 min and the cells were resuspended in 100 μL of 4 × protein buffer (Solarbio, Beijing, China). The cells were then disrupted by incubation in a boiling water bath for 10 min, after which the mixture was subjected to 8% sodium dodecyl sulphate polyacrylamide gel electrophoresis (SDS-PAGE).
Results
Cloning of the SbHMGR gene DNA and cDNA from S. baumii
The SbHMGR gene was amplified from S. baumii, the sequencing results showed that 5094 bp DNA sequence and 4202 bp cDNA sequence were obtained. The comparison of the twos showed that SbHMGR gene DNA sequence contained four exons and three introns (). The SbHMGR gene cDNA sequence, encoding a putative 1389 amino-acid residue protein, was deposited in NCBI GenBank (Accession No. MN505212).
Analysis of the deduced protein sequence of SbHMGR protein
Searches at NCBI identified that SbHMGR protein was a member of the HMG-CoA reductase superfamily and had conserved domains. ProtParam predictive analysis showed that molecular weight of the protein was 147.53 kDa, with a theoretical pI of 8.79, a GRAVVY hydropathicity score of 0.001 and an instability index (II) of 49.09, indicating that SbHMGR protein was an unstable hydrophobic protein, which was consistent with the AcHMGR protein [Citation29]. The analysis of signal peptide and transmembrane region suggested that a possible signal peptide is predicted between pos. 36 and 37. While there were four transmembrane structure in the SbHMGR protein, suggesting that SbHMGR was a secretory protein. Similar results that HMGR protein was an integral membrane glycoprotein were also proven in eukaryotes [Citation30,Citation31]. The three-dimensional structure of SbHMGR protein was predicted using the Swiss model software, and HMG-CoA reductase crystal structure was selected as the template (SMTL ID: 3cd0.1.C) to simulate the SbHMGR three-dimensional structure [Citation32]. The sequence identity between SbHMGR protein and hmg-coa reductase inhibitors was 59.03% (). These results further confirmed that the SbHMGR protein was a member of the HMG-CoA reductase superfamily.
The result of the phylogenetic analysis () was consistent with the known biological evolution. The SbHMGR amino acid sequence had a distant genetic relationship with algae HMGR amino acid sequences, and was most closely related with fungal HMGR proteins, the explanation may be due to the differential expression of HMGR protein between eukaryotes and prokaryotes during biological evolution. In particular, SbHMGR protein showed the highest similarity with the HMGR protein sequences of G. lucidum and Lentinus tigrinus, which was nearly consistent with the evolutionary relationship of GlHMGR protein [Citation19]. It was speculated that the SbHMGR protein had similar function in triterpenoids biosynthesis as those of G. lucidum.
Relationship between SbHMGR enzyme activity and triterpenoids content
SbHMGR, which is the first rate-limiting step in MVA pathway, is also recognized to be a key regulatory enzyme in the biosynthesis of some important secondary metabolites. As secondary metabolites, triterpenoids content was largely dependent on the activity of SbHMGR enzyme, so the SbHMGR enzyme activity and triterpenoids content were detected in detail. The results showed that the triterpenoids content of S. baumii changed dynamically at different developmental stages (). The triterpenoids content of S. baumii increased gradually from 8–14th day in the mycelium stage, with the highest triterpenoids content being detected on the 14th day (3.22 mg/g dry weight). Then, the triterpenoids content gradually declined after the 14th day. The changing trend of triterpenoids content was nearly consistent with the SbHMGR enzyme activity, indicating that the SbHMGR enzyme may play a key catalytic role in biosynthesis of triterpenoids.
Transcript level of SbHMGR gene at different developmental stages
Triterpenoids content was determined by the transcript level of gene, to investigate the transcript level of SbHMGR gene at different developmental stages of S. baumii, qRT-PCR was performed. The results showed that the transcript level of SbHMGR gene also changed dynamically at different developmental stages (). In general, the transcript level of SbHMGR gene gradually increased from 8–12th day in the mycelium stage, before gradually decreasing from 12th day to the primordium stage, the changing trend of SbHMGR gene transcript level was positively correlated with the SbHMGR enzyme activity and triterpenoids content. The results suggested that SbHMGR gene affected the SbHMGR enzyme activity and regulated the production of triterpenoids content.
Figure 6. The transcript level of the SbHMGR, SbMVD, SbIDI and SbLSD at different developmental stages in S. baumii.
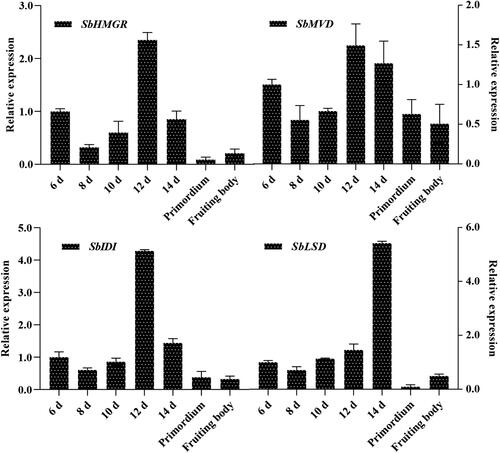
As the key genes in triterpenoids biosynthetic pathway, the transcript level of SbMVD, SbIDI [Citation23], SbLSD were also measured. Transcript level of the three genes were almost analogical, increased, then decreased and peaked on 12th or 14th day in the mycelium stage. Moreover, the changing trend of three genes transcript level were also nearly consistent with the triterpenoids content, indicating that the SbMVD, SbIDI, SbLSD were also involved in triterpenoids biosynthetic pathway and played the important role [Citation16–18].
Prokaryotic expression of SbHMGR gene in Escherichia coli BL21
To further confirm that the obtained SbHMGR gene cDNA can be expressed in prokaryotic, the whole SbHMGR gene ORF was linked into the expression vector pET-32a and then transformed into E. coli BL21(DE3). The results of SDS-PAGE showed that the recombinant vector produced clear protein bands with a molecular weight of approximately 168.53 kDa (including a 21.0 kDa fusion tag protein) (), which was consistent with the theoretical value (147.53 kDa). The result indicated that the SbHMGR gene could be expressed in prokaryotic.
Figure 7. Prokaryotic expression of SbHMGR in E. coli BL21(DE3) with IPTG induction. Lane M was the protein marker; lane 1 is the blank control, E. coli BL21(DE3) harbouring pET-32a vector without IPTG induction; lane 2-5 E. coli BL21(DE3) harboring vector pET-32a-SbHMGR induced by IPTG (1 mmol/L) at 0, 2, 4 and 6 h, respectively.
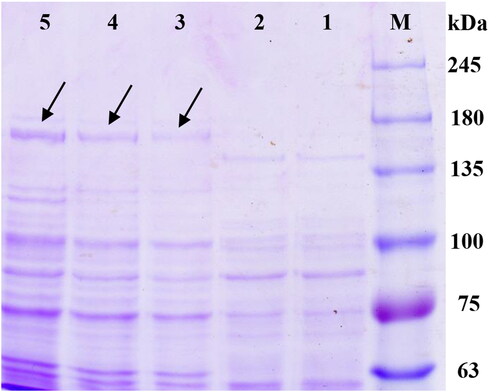
Discussion
It had been repeatedly reported that HMGR was a rate-limiting enzyme in the biosynthetic pathway of triterpenoids both in plants and fungi. High activity of HMGR can significantly increase the accumulation of triterpenoids. On the contrary, if its enzyme activity is inhibited, the triterpenoids yield dramatically declines [Citation13,Citation15,Citation19,Citation28]. In this study, we found that the SbHMGR enzyme activity and transcript level of SbHMGR gene were closely related to the variation of triterpenoids content, similar results were also found in G. lucidum [Citation33,Citation34]. The SbHMGR gene was most actively transcribed during the 12th day of the mycelium culture period, while the highest SbHMGR enzyme activity and triterpenoids content were measured on the 14th day. The reason could be explained by the induced expression of the biosynthetic gene occurring much earlier than the expression of protein and accumulation of secondary metabolites, which were also the case for Ganoderic acids and triterpenoids ginsenoside production [Citation35,Citation36]. The SbHMGR enzyme activity reached the highest at the fruiting body stage (4.25 U/g), which may be explained by its involvement in the synthesis of other secondary metabolites at the fruiting body stage, such as monoterpenoids, sesquiterpenoids, diterpenoids or pigments [Citation35].
Some key enzyme genes involved in triterpenoids biosynthetic step had not been identified yet in S. baumii, so more investigations of genes transcript level were carried out. The downstream genes (SbMVD, SbIDI) of SbHMGR gene showed the same tendency, reached the maximum on the 12th day in the mycelium stage, and were 1.49-fold and 4.29-fold, respectively, higher than the control (6th day). Both downstream genes were most actively transcribed during the 12th day of the mycelium stage, while the highest triterpenoids content appeared on the 14th day, it was consistent with the well-known conclusion that genes transcript occurred earlier than secondary metabolites. The results further confirmed that SbMVD and SbIDI were involved in the biosynthetic pathway of triterpenoids in S. baumii [Citation32,Citation37–39]. Uniquely, the transcript level of SbLSD was positively correlated with the triterpenoids content, but the highest value appeared on the 14th day (5.42-fold than control), the result contradict that gene transcript usually occurred earlier than secondary metabolites, the reason may be that SbLSD gene was involved in the demethylation of triterpenoids [Citation40]. Further study is needed to confirm the exact role of SbLSD in the biosynthetic pathway of triterpenoids of S. baumii.
In addition, we determined the effect of different induction times on the expression level of SbHMGR gene, the content of the target protein was markedly higher than that of other proteins, the results indicated that the SbHMGR protein was successfully synthesized in E. coli BL21(DE3). However, the target protein content is relatively low, the reason can be explained by its high molecular weight of protein result in lower yield per unit time or influenced by some other factors. In future, we will try to construct Agrobacterium-mediated transformation system, research the function of SbHMGR gene, and obtain the higher triterpenoids content strain of S. baumii [Citation25].
Conclusions
SbHMGR, a key enzyme gene was obtained from S. baumii, encoding a putative 1389 amino-acid, contained three introns and four exons. The enzyme activity and qRT-PCR measured showed that the SbHMGR activity and SbHMGR transcript level were closely related to the change in triterpenoids content, and three other genes had been linked to the biosynthesis of triterpenoids. The data will be valuable for elucidation of the biosynthesis of triterpenoids and lay a foundation for genetic modifications to improve triterpenoids yield.
Disclosure statement
The authors declare that they have no conflict of interest.
Data availability
All data that support the findings from this study are available from the corresponding author upon reasonable request.
Additional information
Funding
References
- Liu MM, Zeng P, Li XT, et al. Antitumor and immunomodulation activities of polysaccharide from Phellinus baumii. Int J Biol Macromol. 2016;91:1199–1205.
- Guo JJ, Zhu TB, Collins L, et al. Modulation of lung cancer growth arrest and apoptosis by Phellinus Linteus. Mol Carcinog. 2007;46(2):144–154.
- Zhu T, Guo J, Collins L, et al. Phellinus linteus activates different pathways to induce apoptosis in prostate cancer cells. Br J Cancer 2007;96(4):583–590.
- Zhu TB, Kim SH, Chen CY. A medicinal mushroom: Phellinus linteus. Curr Med Chem. 2008;15(13):1330–1335.
- Wu SJ, Liaw CC, Pan SZ, et al. Phellinus linteus polysaccharides and their immunomodulatory properties in human monocytic cells. J Funct Foods 2013;5(2):679–688.
- Park IH, Chung SK, Lee KB, et al. An antioxidant hispidin from the mycelial cultures of Phellinus linteus. Arch Pharm Res. 2004; 27(6):615–618.
- Kim BC, Choi JW, Hong HY, et al. Heme oxygenase-1 mediates the anti-inflammatory effect of mushroom Phellinus linteus in LPS-stimulated RAW264.7 macrophages. J Ethnopharmacol. 2006;106(3):364–371.
- Kim SH, Lee HS, Lee S, et al. Mycelial culture of Phellinus linteus protects primary cultured rat hepatocytes against hepatotoxins. J Ethnopharmacol. 2004;95(2-3):367–372.
- Szakiel A, Pączkowski C, Koivuniemi H, et al. Comparison of the triterpenoid content of berries and leaves of lingonberry Vaccinium vitisidaea from Finland and Poland. J Agric Food Chem. 2012;60(19):4994–5002.
- Shanmugam MK, Dai XY, Kumar AP, et al. Oleanolic acid and its synthetic derivatives for the prevention and therapy of cancer: preclinical and clinical evidence. Cancer Lett. 2014;346(2):206–216.
- Dai YC, Zhou LW, Cui BK, et al. Current advances in Phellinus sensu lato: medicinal species, functions, metabolites and mechanisms. Appl Microbiol Biotechnol. 2010;87(5):1587–1593.
- Sun TT, Zou L, Zhang LL, et al. Methyl jasmonate induces triterpenoid biosynthesis in Inonotus baumii. Biotechnol Equip. 2017;31(2):312–317.
- Wei H, Xu C, Movahedi A, et al. Characterization and function of 3-hydroxy-3-methylglutaryl-CoA reductase in Populus trichocarpa: Overexpression of PtHMGR enhances terpenoids in Transgenic Poplar. Front Plant Sci. 2019;10(1476).
- Zhang M, Liu H, Wang Q, et al. The 3-hydroxy-3-methylglutaryl-coenzyme A reductase 5 gene from Malus domestica enhances oxidative stress tolerance in Arabidopsis thaliana. Plant Physiol Biochem. 2020;146:269–277.
- Chappell J, Wolf F, Proulx J, et al. Is the reaction catalysed by 3-hydroxy-3-methylglutaryl coenzyme A reductase a rate-limiting step for isoprenoid biosynthesis in plants?Plant Physiol. 1995;109(4):1337–1343.
- Liao P, Hemmerlin A, Bach TJ, et al. The potential of the mevalonate pathway for enhanced isoprenoid production. Biotechnol Adv. 2016;34(5):697–713.
- Sun J, Zhang YY, Liu H, et al. A novel cytoplasmic isopentenyl diphosphate isomerase gene from tomato (Solanum lycopersicum): Cloning, expression, and color complementation. Plant Mol Biol Rep. 2010;28(3):473–480.
- Chen C, Kalb VF, Turi TG, et al. Primary structure of the cytochrome P450 lanosterol 14 alpha-demethylase gene from Candida tropicalis. DNA. 1988;7(9):617–626.
- Shang CH, Zhu F, Li N, et al. Cloning and characterization of a gene encoding HMG-CoA reductase from Ganoderma lucidum and its functional identification in yeast. Biosci Biotech Biochem. 2008;72(5):1333–1339.
- Dai ZB, Cui GH, Zhou SF, et al. Cloning and characterization of a novel 3-hydroxy-3-methylglutaryl coenzyme A reductase gene from Salvia miltiorrhiza involved in diterpenoid tanshinone accumulation. J Plant Physiol. 2011;168(2):148–157.
- Yang R, Yuan BC, Li WD, et al. Improving the accumulation of 18 α- and 18 β-glycyrrhizins by over-expressing GuHMGR, GuSQS1, and GuBAS genes in Glycyrrhiza uralensis. J Tradit Chin Med Sci. 2017;4(4):336–349.
- Zhang DH, Jiang LX, Li N, et al. Overexpression of the squalene epoxidase gene alone and in combination with the 3-hydroxy-3-methylglutaryl coenzyme A gene increases ganoderic acid production in Ganoderma lingzhi. J Agric Food Chem. 2017;65(23):4683–4690.
- Liu ZC, Sun TT, Wang SX, et al. Cloning, molecular properties and differential expression analysis of the isopentenyl diphosphate isomerase gene in Sanghuangporus baumii. Biotechnol Equip. 2020;34(1):623–630.
- Ali Q, Salisu IB, Raza A, et al. A modified protocol for rapid DNA isolation from cotton (Gossypium spp.). Methods 2019;6:259–264.
- Wang XT, Wang SX, Xu XR, et al. Molecular cloning, characterization, and heterologous expression of an acetyl-CoA acetyl transferase gene from Sanghuangporus baumii. Protein Expres Purif. 2020;170:105592.
- Herlinda C, Gerardo C, Edgar NC, et al. cDNA cloning, heterologous expression, protein folding and immunogenic properties of a phospholipase A2 from Bothrops ammodytoides venom. Protein Expres Purif. 2019;154:33–43.
- Sun TT. Analysis and systematic mining of genes involved in the biosynthetic pathway of triterpenoids in Sanghuangporus baumii [PhD dissertation]. Northeast Forestry University; 2017. (in Chinese).
- Livak KJ, Schmittgen TD. Analysis of relative gene expression data using real-time quantitative PCR and the 2 −ΔΔCT method. Methods 2001;25(4):402–408.
- Yuan XL, Xiao ZY, Chen LY, et al. Cloning and expression analysis of 3-hydroxy-3-methylglutaryl coenzyme a reductase gene in Antrodia Camphorata (AcHMGR). GAB. 2018;37(1):358–365. (in Chinese).
- Liscum L, Finer-Moore J, Stroud RM, et al. Domain structure of 3-hydroxy-3-methylglutaryl coenzyme A reductase, a glycoprotein of the endoplasmic reticulum. J Biol Chem. 1985;260(1):522–530.
- Basson ME, Thorsness M, Finer-Moore J, et al. Structural and functional conservation between yeast and human 3-hydroxy-3-methylglutaryl coenzyme A reductases, the rate-limiting enzyme of sterol biosynthesis. Mol Cell Biol. 1988;8(9):3797–3808.
- Waterhouse A, Bertoni M, Bienert S, et al. Swissmodel: Homology modelling of protein structures and complexes. Nucleic Acids Res. 2018;46(2):296–303.
- Zhang WX, Tang YJ, Zhong JJ. Impact of oxygen level in gaseous phase on gene transcription and ganoderic acid biosynthesis in liquid static cultures of Ganoderma lucidum. Bioprocess Biosyst Eng. 2010;33(6):683–690.
- Liang CX, Li YB, Xu JW, et al. Enhanced biosynthetic gene expressions and production of ganoderic acids in static liquid culture of Ganoderma lucidum under phenobarbital induction. Appl Microbiol Biotechnol. 2010;86(5):1367–1374.
- Xu YN, Xia XX, Zhong JJ. Induction of ganoderic acid biosynthesis by Mn2+ in static liquid cultivation of Ganoderma Lucidum. Biotechnol Bioeng. 2014;111(11):2358–2365.
- Huang C, Zhong JJ. Elicitation of ginsenoside biosynthesis in cell cultures of Panax ginseng by vanadate. Process Biochem. 2013;48(8):1227–1234.
- Shi L, Qin L, Xu YJ, et al. Molecular cloning, characterization, and function analysis of a mevalonate pyrophosphate decarboxylase gene from Ganoderma lucidum. Mol Biol Rep. 2012;39(5):6149–6159.
- Liao ZH, Chen M, Yang YJ, et al. A new isopentenyl diphosphate isomerase gene from sweet potato: Cloning, characterization and color complementation. Biologia 2008;63(2):221–226.
- Liu ZC, Sun TT, Wang SX, et al. The cloning and expression analysis of mevalonate pyrophosphate decarboxylase gene cDNA sequence from Sanghuangporus baumii. J Nanjing Forestry Univ. 2020;44(4):79–85. (in Chinese).
- Lee CH, Hsu KH, Wang SY, et al. Cloning and characterization of the lanosterol 14alpha-demethylase gene from Antrodia cinnamomea. J Agric Food Chem. 2010;58(8):4800–4807.