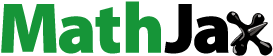
Abstract
This study explored the nematicidal activity of silver nanoparticles (AgNPs), ascorbic (AA) and salicylic acid (SA) compared with Mycorrihizin (Myco) against Meloidogyne javanica infecting eggplants. The characterization and size confirmation of the AgNPs was done by ultraviolet–visual (UV–vis) spectrophotometry, surface zeta-potential and transmission electron microscopy. The results illustrated that 150 μg/mL AgNPs had nematicidal activity against the second-stage juvenile and egg hatching of M. javanica in vitro, and caused 100% mortality in juveniles after 48 h of exposure. In open-field conditions, the treatment with 100 µg and 150 µg AgNPs 24 h before transplanting killed almost all of the nematode eggs and galls per root system compared with the control. All treatments improved the eggplant growth in terms of plant height, root weight, number and weight of fruits, shoot and root length and fresh weight of shoot and root with various degrees as compared to control. The activities of oxidative enzymes, peroxidase (POX), polyphenol oxidase (PPO) and phenylalanine ammonia lyase (PAL), during the flowering stage, increased with all treatments comparing to healthy and infected plants. To assess the defense response of infected eggpant, the gene expression levels (PAL, POX and PPO) were monitored using quantitative reverse transcription polymerase chain reaction (qRT-PCR). The PPO enzyme showed the highest rate on day 60 after infection when 50 µg AgNPs were used during transplanting. The POX enzyme activity showed the highest increase in all treatments during all stages of growth, on day 30, 45 and 60.
Introduction
Eggplant (Solanum melongena) is one of the most important vegetable crops from Solanaceae family, where it occupies third place after potatoes and tomatoes for fresh consumption and processing. Eggplant is a vital cheap summer vegetable as it contains 92.7% water, 1.4% protein, 103% fiber, 0.3% fat, 0.3% minerals, and the remaining 4% consists of various carbohydrates and vitamins (A and C) [Citation1]. Eggplant is susceptible to numerous diseases and parasites, particularly Fusarium and Verticillium wilts, insects and nematodes [Citation2]. Nematodes cause crop production problems which occur as a result of root dysfunction, reduced rooting volume and utilization of water and nutrients. Several different genera and species of nematodes can be important to crop production. In general root–knot nematodes are the most economically important group of plant parasitic nematodes worldwide. These biotrophic parasites attack more than 2000 plant species, making them a major threat [Citation3–5], especially on eggplant. This crop is highly vulnerable to plant parasitic nematodes, root–knot nematodes, so they cause heavy losses in the yield. The infection causes root galls and impedes the normal uptake of water and nutrients, which is responsible for yield losses up to 16.8–85.0% [Citation3]. Though the use of nematicides is very effective in nematode control, their limited utilization is attributed to higher cost, non-availability, environment pollution, residual problems, etc. Therefore, a control strategy of nematodes should be developed which may be safe, environmentally friendly and cost effective [Citation6]. Biological control agents have disadvantages of possessing a narrow range of treatment effects and a lack of reliability under varying environmental conditions. As an example, a Pasteuria sp., is a bacterial parasite of sting nematodes Belonolaimus longicaudatus, and was developed as a commercial biological nematicide [Citation7]. The commercial formulation of Pasteuria sp. was ineffective for sting nematodes management on golf course turf [Citation8], and does not work for the other species of plant–parasitic nematodes such as root–knot nematodes.
It was therefore imperative to find alternative solutions to this challenge. A new strategy for controlling diseases is based on activation of the plant defense system. Abiotic agents have been used for induction of resistance against root–knot nematode, therefore inducers attracted attention during the last decades. Osman et al. [Citation9] used ascorbic acid by foliar spray and root dipping technique on eggplant under greenhouse conditions and showed that induced systemic resistance led to synthesis of plant defense enzymes including peroxidase (POX), polyphenol oxidase (PPO) and phenylalanine ammonia lyase (PAL). Other studies [Citation10–11] used SA to control the root–knot nematode Meloidogyne incognita and found that SA significantly reduced the root galling and final nematode population on eggplant roots, and the treatments with SA increased the activity of POX enzyme compared with control. Sharma and Sharma [Citation12] studied the effect of AMF (arbuscular mycorrhizal fungi) on root–knot nematode in tomato plants and they observed significant effect on plant growth parameters and demonstrated that reduction of root–knot nematode parameters is due to AMF. Cromwell et al. [Citation13] studied the effect of silver nanoparticles (AgNPs) on root–knot nematode in vitro. They found that AgNPs decreased the activity of M. incognita. As the exposure time and concentration of AgNPs increased, the percentage of healthy second-stage juveniles (J2S) decreased. Under field conditions they found that AgNPs reduced gall formation in turfgrass roots caused by M. graminis compared with untreated plants. Abdellatif et al. [Citation14] studied the effect of green silver nanoparticles (GSN) extracted from two algal species (Ulva lactuca and Turbinaria turbinata). They found that (GSN) with concentration of 127.5 μg/mL were effective in controlling root–knot nematode.
The objective of this study was to investigate the effect of salicylic acid (SA), ascorbic acid (AA), Mycorrihizin and AgNPs on the enzyme activity of POX, PPO and PAL and the expression of those three genes in eggplant infected with Meloidogyne javanica and their effect on nematode parameters under laboratory and field conditions.
Materials and methods
Antioxidants
Two antioxidants, namely ascorbic acid (AA) and salicylic acid (SA), were purchased from El-Gamhoria Chemical Co. (Egypt). The antioxidants were dissolved separately in water to obtain the desired concentrations, 2.5, 5.0, and 10.0 mmol/L, for each antioxidant in order to test their effects on the Meloidogyne infected eggplant.
Mycorrihizin
Mycorrihizin is a nematicide commercial product. It was obtained from the Soil, Water and Environmental Research Institute, Egypt. It was used in the recommended dose of 5 g/pot during transplanting.
Chemicals
Silver nitrate (AgNO3; 99.99%) was purchased from Votre Partenaire Chimie (SdS), Peypin, France.
Nematodes extraction
Rhizosphere soil and associated roots, about 500 cm3 each, were cautiously collected using a small agricultural hoe and were placed in labeled bags. The samples were kept in a cold sampling box till sending to the Nematology Laboratory at the Plant Protection and Biomolecular Diagnosis Department, Arid Lands Cultivation Research Institute (ALCRI), City of Scientific Research and Technological Applications (SARTA, City), Alexandria, Egypt, for begin nematode extraction and identification. Plant–parasitic nematodes were obtained from 250 cm3 of soil sample according to [Citation15] with slight modification, within 24 h after sample collection. Each soil sample was thoroughly mixed, and a volume of 250 cm3 soil was washed for nematode extraction by means of Cobb’s wet-sieving and centrifugal sugar flotation techniques. The root samples were gently washed with tap water to remove all adhering soil particles and were cut into smaller pieces and examined for root galling and root–knot nematode infection. The egg masses, galled roots were soaked in solution of Phloxine B stain 0.15 g/L for 15–20 min and then rinsed in running water to remove residual stain. The number of egg masses was recorded for perineal pattern nematode identification and assay experiments.
Root–knot nematodes (RKN) identification
Ten stationary adult females of the RKN were isolated from the infected galled roots using a needle, and their posterior terminations were directly cut and immerged in lactic acid solution (45%), mounted in a glycerin drop on a microslide, cover slipped using nail polish and examined under a stereomicroscope [Citation16]. Identification of the perineal patterns was done according to [Citation17] at the Plant Protection and Biomolecular Diagnosis Department, Arid Lands Cultivation Research Institute (ALCRI), City of Scientific Research and Technological Applications (SARTA, City), Alexandria, Egypt
Pathogenicity test
The pathogenicity test was conducted under greenhouse conditions at an average temperature of 35 ± 2 °C. Seedlings (n = 3) of each eggplant variety, Anan, 9999, Black Beauty and Classic, were maintained in pots (30 cm in diameter) filled with sterilized sand and clay in a ratio of 2:1. Each plant was inoculated with a suspension of approximately 5000 eggs and newly hatching second-stage juveniles (J2S) obtained from the pure single egg mass culture of the original M. javanica population. Fifteen pots were used for each eggplant variety. Pots of each variety were divided in two groups, the first was infected with approximately 5000 eggs and newly hatching J2S of M. javanica, the second one was untreated and served as a control. Seventy days after inoculation, plants were carefully uprooted, and roots were galled washed with a gentle stream of running tap water. Nematode eggs were extracted from eggplants roots by cutting the entire root into 2.5-cm pieces, placing the pieces in a round flask, and agitating for 3 min in a 1% NaOCl, according to [Citation18] with slight modification. The number of eggs, number of galls on the root system per plant and number of nematodes per 250 cm3 soil were determined.
Preparation of the AgNPs
AgNPs were chemically synthesized according to [Citation19, Citation20] with slight modifications, as follows. A 100-mL solution of 1.0 × 10−3 mol/L silver nitrate (AgNO3) was mixed with a 300-mL aqueous solution of 2.0 × 10−3 mol/L sodium borohydride (NaBH4). Millipore water was utilized for these solutions, and both solutions were cooled at 0 °C before blending. The mixture solution was stirred vigorously at 1200 rpm until a change to dark color appeared for an hour until it became stabilized and constant. It was concentrated ten times using a rotary vacuum evaporator. Then, by diluting this solution, each sample of different concentrations was used to determine the most efficient concentration of AgNPs against root–knot nematodes.
Characterization of the resulting AgNPs
To portray the characteristics of the synthesized AgNPs, there are some properties that should be measured in the Nanotechnology Center, Faculty of Science, Kafr Elsheikh University. Particles size and surface charge were determined as described by [Citation21, Citation22] as follows:
UV–Vis spectroscopy (UV/Vis)
The free-radical generation from Ag nanoparticles was recorded on a UV–Vis spectrometer Shimadzu UV-2450 equipped with a double-beam system in the range of 190–1100 nm. The spectrometer outputs were recorded using the OrginPro6.1 software.
Transmission electron microscopic imaging (TEM)
The morphology (shape and size) and distribution of the obtained Ag nanoparticles were characterized by transmission electron microscopy (TEM). Several drops of AgNPs aqueous colloidal solution were dropped onto a TEM copper grid, and the solvent was removed by drying. The resulting TEM image was obtained by high-resuspension TEM (JEOL, JEM-2100) at an accelerating voltage of 200 kV. The produced particle size and distribution were derived from a histogram considering more than 100 particles determined using multiple TEM micrographs with the help of ImageJ 1.49v software [Citation23].
Surface zeta-potential
The operative zeta potential in aqueous colloidal solutions of different AgNPs was measured by a Brookhaven zeta potential/particle size analyzer, and the values are shown as a mean value of three independent experiments.
Nematicidal activity (in vitro)
Egg hatching
Eggs hatchability activity was assessed using eggs of Meloidogyne javanica. Stock solutions of AgNPs were prepared in distilled water as follows: 0, 12.5, 25, 50, 100 and 150 µg/L (10 mL). One milliliter of nematode concentrate (400 eggs) was placed in glass vials with the AgNPs solution, distilled water was used as a control. Their activity was studied against M. javanica. Hussey and Barker’s method was used to isolate the eggs of M. javanica from a pure culture maintained on eggplant (Solanum melongena). Roots were placed on sterilized distilled water and incubated for 24 h at room temperature for hatching. Each treatment had five replications. Hatched eggs were examined under a microscope and were counted after 14 days. According to [Citation24], egg hatching (%)= [number of juveniles/(number of juveniles + number of eggs)] × 100 Reduction (%)= [(egg hatching in the control – egg hatching in the treatment)/Egg hatching in the control] × 100.
Juveniles mortality
Approximately 140 freshly hatched second stage juveniles of M. javanica in 1 mL distilled water were poured into the wells of 6-well tissue culture plates, where stock solutions of AgNPs were prepared in distilled water as follows 0, 12.5, 25, 50, 100 and 150 µg/L (10 mL aliquots). The plates were then covered with the lid and kept in an incubator at 25 °C. A 2 mL volume of distilled water containing nematode larvae served as control. Each treatment was replicated four times. Dead nematodes were counted and recorded after 24, 48 and 72 h. Mobility was confirmed by touching the nematode with a fine needle. Nematodes that showed no realistic movement were considered dead. Percentages of nematode mortality were then calculated and recorded for each concentration according to [Citation25]: percent juveniles mortality (%)=(number of juveniles killed/total number of juveniles)×100.
Open field experiment
The open field experiment was carried out in May 2019 at Meniat genag village, Desouq, Kafr–Elshiekh Governorate, Egypt, in clay loam soil irrigated by drip irrigation in a field naturally infested with M. javanica. Forty-five-day-old eggplant (Solanum melongena L.) cv. Classic seedlings were transplanted in the infested field. The experimental area was divided into plots each containing rows (4 m long and 60 cm apart), in which the distance between the plants was 1 m. The experiment was set up in a completely randomized block design with 26 treatments and 5 plants per treatment (with a total of 130 plants) as follows:
The experiment was set up in a completely randomized block design with 26 treatments:
1–2. Salicylic acid (SA): 50 mL of SA at a concentration 5000 ppm [Citation4] was used as a spray and as a drench (soaking solution) 24 h before transplanting.
3–4. Ascorbic acid (AA): 50 mL of AA at a concentration 1000 ppm [Citation9] was used as a spray and as a drench 24 h before transplanting.
5. Mycorrihizin: was used in the recommended dose of 5g/pot during transplanting.
6–7. SA and Mycorrihizin: 50 mL of SA at a concentration of 5000 ppm used as a spray and as a drench on the plant 24 h before transplanting, then 5 g/plant of mycorrihizin were added during transplanting.
8–9. AA and Mycorrihizin: 50 mL of AA at a concentration of 1000 ppm was used as a spray and as a drench on the plant 24 h before transplanting, then 5 g/plant of Mycorrihizin were added during transplanting.
10–12. AgNPs: 10 mL of AgNPs at concentrations of 50, 100 and 150 µg/L used as a drench 24 h before transplanting.
13. AgNps + SA: 50 mL of SA at a concentration of 5000 ppm were used as a spray on the plant 24 h before transplanting, then 10 mL of AgNPs were added at a concentration of 150 µg/L used as a drench during transplanting.
14–16. AgNPs + SA: 50 mL of SA at a concentration of 5000 ppm were used as a drench on the plant 24 h before transplanting, then 10 mL of AgNPs were added at concentrations of 50, 100 and 150 µg/L used as a drench during transplanting.
17. AgNPs + AA: 50 mL of SA at a concentration of 1000 ppm was used as a spray on the plant 24 h before transplanting, then 10 mL AgNPs were added at a concentration of 150 µg/L used as a drench during transplanting.
18–20. AgNPs + AA: 50 mL of SA at a concentration of 1000 ppm was used as a drench on the plant 24 h before transplanting, then 10 mL of AgNPs were added at concentrations of 50, 100 and 150 µg/L used as a drench during transplanting.
21–23. AgNps + Mycorrihizin: 5 g/pot of mycorrihizin was given as a recommended dose during transplanting, then 10 mL of AgNPs were added at concentrations of 50,100 and 150 µg/L.
24–26. AgNPs: 10 mL of AgNPs at concentrations of 50, 100 and 150 µg/L were used as a drench during transplanting.
At day 30, 45 and 70 after transplanting, eggplant leaves were harvested for determination of oxidative enzymes, peroxidase (POX), polyphenol oxidase (PPO) and phenylalanine ammonia lyase (PAL), during the flowering and blooming stage of plants. Final nematode soil populations were extracted [Citation26], the number of J2S/250 cm3 soil, galls/root system, egg masses/root system and eggs/root system were counted and reduction % of J2S was calculated as follows:
The increase of plant growth parameters was evaluated by determining the length of plant, length of root system, fresh and dry weight of shoot system, weight of root system, number of flowers and fruit per plant and weight of fruits per plant. The increasing % was calculated as follows:
Assay of defense-related enzyme
Enzymes extracts were prepared according to [Citation27]. One gram of fresh weight of eggplant leaf samples were ground with liquid nitrogen and homogenized in 4 mL of 0.1 mol/L sodium phosphate buffer (pH 6.8) in an ice bath. The homogenates were filtered through 4 layers of cheese cloth and the filtrates were centrifuged at 6000g for 15 min and 4 °C and then the clear supernatants were used to evaluate the enzyme activities of peroxidase (POD), polyphenol oxidase (PPO), phenylalanine ammonia lyase (PAL).
PPO (polyphenol oxidase) enzyme activity was measured using the oxidation of pyrogallol method according to [Citation28, Citation29] with slight modification. Briefly, 0.5 mL of 0.1 mol/L sodium phosphate buffer at pH 6.8, 0.3 mL enzyme extract, 0.3 mL of 0.05 pyrogallol (m.w. C6H3COH3=126.11), 0.1 mL of 10% H2O2 and distilled water were pipetted into a cuvette to a volume of 3.0 mL. the mixture was incubated in a water bath at 35 °C for 5 min, and then the optical density at 425 nm was measured. One unit of PPO activity was presented as the change in absorbance of 0.01 at 425 nm per 1 g fresh weight per minute.
POD enzyme activity was measured using the guaiacol assay. The reaction mixtures contained 50 μL of enzyme extract supernatant and 4.8 mL of 18 mmol/L guaiacol (mixed with 0.1 mol/L sodium phosphate buffer, pH 5.8); the mixture was incubated in a bath at 35 °C for 1 min, and 150 μL of H2O2 (2.5%) was added to start the reaction. The absorbance was measured at 495 nm using a spectrophotometer (model T60-PG instruments) and recorded at 0, 1, 2, 3, 4 min. The POD activity was expressed as change in absorbance (optical density/min/0.5 g).
PAL enzyme activity was assayed in sodium borate buffer, pH 8.8. The reaction mixture contained 0.5 mL enzyme preparation, 1.5 mL borate buffer 0.2 mol/L, pH 8.8, 1 mL of 1% phenylalanine and 2.5 mL deionized water. One milliliter of deionized water was added instead of phenylalanine as a blank. The mixture was incubated at 40 °C for 1 h. The reaction was stopped by adding 0.5 mL of 5 N HCl to each tube. The enzyme activity was expressed as optical density at 290 nm.
Statistical analysis
The data were processed by Microsoft Excel version 2007. Statistically, the obtained data were subjected to analysis of variance (ANOVA) as a factorial in complete block design [Citation30] followed by Duncan’s multiple range test to compare the means [Citation31].
Quantitative reverse transcription-polymerase chain reaction (qRT-PCR)
We performed qRT-PCR to analyze the expression of the peroxidase, polyphenol oxidase and phenylalanine ammonia lyase genes. Total RNA was isolated from 26 eggplant roots sampled (infected with M. javanica in comparison to 26 treatments with application of AgNPs alone and/or in combination with various inducers (Mycorrihizin, SA, AA, SA (spray)+Myco., SA (drench)+Myco., AA (spray)+Myco., AA (drench)+Myco) at different concentrations) using TRIzol reagent (Invitrogen, USB Cooperation, Cleveland, Ohio, USA), according to the manufacturer’s protocol. Root tissues were ground well to a fine powder with sterilized glass beads. About 500 mg root tissue from each treatment was used for RNA extraction, and the final RNA product was dissolved in 30 µL RNAase-free water. RNA integrity was checked on ethidium bromide stained 1.5% w/v agarose gel and the purified total RNA concentration was determined by measuring the absorbance at 260 and 280 nm using Spectrostar Nano (BMG LABTECH GmbH/ Germany). Complementary DNA (cDNA) was synthetized from total RNA with oligo (dT) primer, dNTPs and reverse transcriptase (RT) enzyme (M-MLV, RT, Fermentas, USA) according to the standard protocol and buffer (5X): 3 µL of RNA was added to (10 µL (5x) RT – Buffer), 2.5 µL (25 mmol/L) dNTPs, 5 μL from oligo (dT) primer (20 pL/μL), 0.25 µL (20 µ/µL) RT enzyme and 4.3 µL H2O. RT-PCR amplification was performed in a thermal cycler (Eppendorf, Germany): the mixture was incubated at 40 °C for 1 h, then at 70 °C for 10 min, followed by storage at −20 °C until used. Q-PCR was performed on the resulting cDNA using a Light Cycler Rotor-Gene 6000 system instrument (Qiagen, United States) with a set of gene-specific primers, Phenylalanine ammonia-lyase (PAL), F-GAGGTGGACAAGGTGTTCGT, R-TTGCCACAACCCACAACTAA [Citation32], Polyphenol oxidase (PPO) F-CATGCTCTTATGAGGCGTA, R-CCATCTATGGAACGGGAAGA according to [Citation33], Peroxidase (POX) F-GCTTTGTCAGGGGTTGTGAT, R-TGCATCTCTAGCAACCAACG according to [Citation34], and the eggplant ITS rDNA gene was used as a house-keeping gene. The final volume of each reaction (25 μL) contained 12.5 μL of 2× Quantitech SYBR® Green RT Mix, forward and reverse primers at 1.5 μL of 10 pmol/μL, 2 μL of template cDNA (50 ng) and 7.5 μL of RNase-free water. The reactions were performed in duplicate using the following thermal cycling conditions: an initial denaturation step at 95 °C for 10 min and a real-time PCR program including predenaturation at 95 °C for 20 min subsequently 45 cycles of 95 °C for 15 s, 60 °C for 30 s, and then a final extension at 72 °C for 30 s. The relative gene expression at day 70 relative to the control condition (day 0) was calculated based on the following equation (ΔCq=Cq – reference gene, ΔΔCq=Cq – control and ΔΔCq expression = 2 (–ΔΔCq). Three biological replicates were analyzed.
Results
Pathogenicity test
The susceptibility of four eggplant cultivars to the root–knot nematode Meloidogyne spp. was examined. The eggplant cv. Classic, Black Beauty, Anan and 9999 were highly susceptible to M. javanica (; ), with a large number of galls 371.25, 259, 209.25 and 140 per root system and nematode egg masses % reduction with respect to control 350.25, 229.75, 178.75 and 119 per root system recorded on roots. In addition, the number of J2S per 250 cm3 of soil was also large: 832.5, 196.75, 132, and 131, respectively.
Figure 1. Effect of the treatments on the root galls of eggplant plants 1) SA spray, 2) SA drench, 3) AA spray, 4) AA, drench, 5) Myco., 6) SA (spray)+Myco, 7) SA (drench)+Myco., 8) AA (spray)+Myco., 9) AA (drench)+Myco., 10) AgNPs before transplanting (50 µg), 11) AgNPs before transplanting (100 µg), 12) AgNPs before transplanting (150 µg), 13) AgNPs (150 µg)+SA (spray), 14) AgNPs (50 µg)+SA (drench), 15) AgNPs (100 µg)+SA (drench), 16) AgNPs (150 µg)+SA (drench), 17) AgNPs (150 µg)+AA (spray), 18) AgNPs (50 µg)+AA (drench), 19) AgNPs (100 µg)+AA (drench), 20) AgNPs (150 µg)+AA (drench), 21) AgNPs (50 µg)+Myco., 22) AgNPs (100 µg)+Myco., 23) AgNPs (150 µg)+Myco., 24) AgNPs (50 µg) during transplanting, 25) AgNPs (100 µg) during transplanting, 26) AgNPs (150 µg) during transplanting, 27) Control.
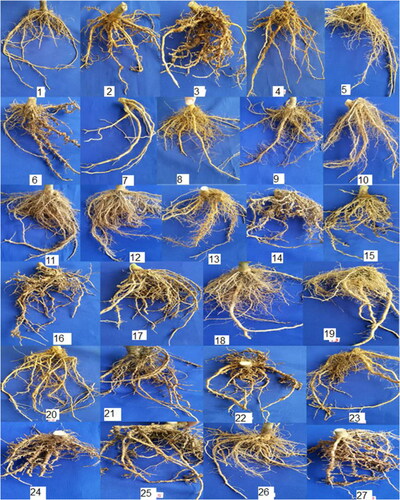
Table 1. Greenhouse evaluation of four eggplant cultivars for resistance to Meloidogyne javanica.
Characterization of silver nanoparticles
In this study, AgNPs were obtained by chemical synthesis. Visual observation of the reaction of sodium borohydride with silver nitrate at room temperature showed no color change could be demonstrated in AgNO3. AgNPs exhibit a unique absorption band displayed as a sharp extinction peak at 403 nm, which refers to the absorption band of spherical AgNPs due to their surface plasmon (). The resultant colloidal solution was stable for more than three months. For more confirmation, other UV–visible absorption spectra were carried out, and the same peak was obtained.
Transmission electron microscopic imaging (TEM) analysis
The particles morphology of the first colloidal solution was observed using TEM (). The size distribution was calculated from the obtained electron micrograph, using ImageJ software. Most of the particles were spherical and monomodally dispersed. As a model, one hundred particles were employed to determine the particle diameter, which was 9.17 nm on average, ranging mostly between 8 and 11 nm with a standard error of 0.577 nm.
Figure 3. Analysis of AgNPs. (A) A TEM image of AgNPs colloidal solution (1) dispersed on a TEM copper grid (a scale bar: 200 nm). (B) A histogram illustrating size distribution of Ag nanoparticles (0–100 nm), and (C) A pie chart representing particles distribution within the resulted histogram (B).
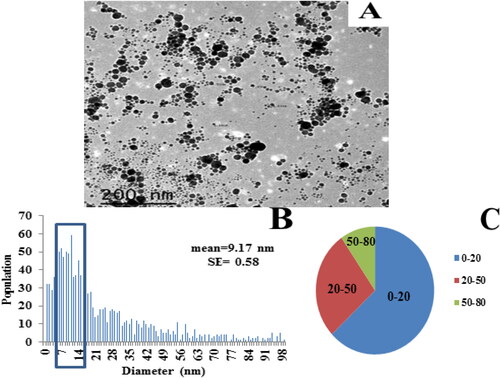
Surface zeta-potential (Z-potential)
The effective zeta potential in the aqueous colloidal solution of the AgNPs was measured. The surface zeta potential of AgNPs in colloidal solution was slightly negative. In the colloid solution, there exist nitrate and borate ions adsorbed on the surface of Ag nanoparticles with the result that the surface charge of the Ag nanoparticles was slightly negative. Also, the presence of trisodium citrate as a capping agent is involved in the negative charge of the particles. illustrates the surface charges of the prepared colloidal solution.
Assessment of the effect of AgNPs on eggs hatchability in vitro
AgNPs significantly decreased M. javanica egg hatching (%) and this effect increased in a concentration-dependent manner from 12.5, 25, 50, 100 to 150 µg/L AgNPs in vitro (). The AgNPs decreased generally the mean egg hatching to 88.8, 60.9, 49.3, 33 and 16.3%, respectively, compared with the control (untreated).
Table 2. Effect of different concentrations of AgNPs on eggs hatching of Meloidogyne javanica under laboratory conditions.
Assessment of the effect of AgNPs on juveniles mortality invitro
All the tested concentrations of AgNPs (12.5, 25, 50, 100 and 150 µg/L) showed reduction of juveniles compared to the control (). At the highest concentration (150 μg/mL), the AgNP treatment caused 100% mortality in juveniles after 48 h from exposure, followed by the concentration of 100 μg/mL, which caused 91 and 100% reduction in juveniles after 48 and 72 h from exposure, respectively. Meanwhile, the three concentrations 50, 25 and 12.5 µg/L killed the nematode juveniles to a moderate extent, with 89, 82 and 73.5% after 72 h from exposure, respectively.
Table 3. Effect of different concentrations of AgNPs on J2S of M. javanica under laboratory conditions.
Evaluation of the effect of biotic and abiotic inducers on eggplants in an open field experiment
The data presented in indicate that all treatments with inducers significantly protected eggplant plants against M. javanica as compared to the untreated control.
Table 4. Effect of SA, AA (antioxidant agent) and Mycorrihizin on root–knot nematode in field conditions.
Table 5. Effect of AgNPs and Mycorrihizin on root–knot nematode in field conditions.
Table 6. Effect of different concentration of AgNPs with SA and AA compared with Mycorrihizin on root–knot nematode in field conditions.
Table 7. Effect of AgNPs with different concentration and combination with Mycorrihizin on plant growth parameters in field conditions.
Table 8. Effect of AgNPs, SA, AA and Mycorrihizin on plant growth parameters in field conditions.
We studied the effect of SA (spray), SA (drench), AA (spray), AA (drench), Mycorrihizin, SA (spray)+Mycorrihizin, SA (drench)+Mycorrihizin, AA (spray)+Mycorrihizin and AA (drench)+Mycorrihizin on the number of galls/root system, number of eggs/root system and number of J2S/250 cm3 soil on eggplant cv. Classic grown in soil infected with M. javanica, 70 days after the corresponding inoculation and treatments (). The mean number of galls/root and mean number of egg masses significantly decreased with the tested agents. SA (drench)+Mycorrihizin, AA (spray)+Mycorrihizin and AA (drench)+Mycorrihizin were the most effective treatments, and reduced both parameters (number of galls and number of eggs per root) to zero compared to 973.75 and 691.25, respectively, for the untreated infected group (control). These treatments reduced the number of J2S/250 cm3 soil to 246.25, 243.75 and 245.75, respectively, compared to 858.75 for the untreated controls infected with the nematode ().
The data in represent the effect of chemically synthesized AgNPs and combinations with Mycorrihizin with various concentrations on the number of galls and eggs/root system and the number of J2S/250 cm3 soil on eggplant cv. Classic grown in soil infected with M. javanica, 70 days after the corresponding inoculation and treatments. Application of 50, 100 and 150 µg AgNPs before transplanting was highly toxic to the nematodes, killing almost 100% of the number of galls and number of eggs per root system compared with those in the untreated control, 973.75 and 691.250, respectively. On the other hand, the number of J2S/250 cm3 soil after treatment with 50 µg AgNPs before transplanting was 368.75, compared with 858.75 in the untreated control. The other treatments gave moderate mortalities ().
The data in indicate that all treatments (AgNPs (50 μg)+AA (drench), AgNPs (100 μg)+AA (drench) and AgNPs (150 μg)+AA (drench) could kill the nematodes. For eggplant plants, the number of galls and eggs per root system, and the final number of second-stage juveniles per 250 cm3 of soil decreased to zero following all treatments ().
The growth of eggplant plants infected with M. javanica was also studied in comparison to 26 treatments with AgNPs alone and/or in combinations with various inducers (Mycorrihizin, SA, AA, SA (spray)+Myco., SA (drench)+Myco., AA (spray)+Myco., AA (drench)+Myco) with different concentrations (). The results revealed that all treatments caused a remarkable improvement in eggplant growth parameters of plant height, weight of root, the number and the weight of fruits per plant shoot and root length and fresh weight of shoot and root at various degrees as compared to the control. Among all tested treatments, SA (drenching) gave the highest increase in most plant growth parameters compared with the control and Mycorrihizin (). The treatment with SA (drenching) was the most effective in terms of plant height (a 47.08% increase) and fresh weight (a 79.15% increase) and length of root (a 63.95% increase). On the other hand, the least effective treatments in terms of plant height were SA (spraying)+Mycorrihizin and AA (spraying)+Mycorrihizin, with an increase of 27.8% and 31.03%, respectively. All treatments significantly affected the length of the root compared with the control, where SA (drench) showed the greatest increase% (63.94%). The weight of root also significantly differed compared with the control. SA (spray) affected the root weight with a decrease by 15.78% and the lowest weight was measured after SA (drench)+Myco treatment with a reduction of 72.10%. Noticeably, the number of fruits/plant significantly increased in the treatments compared with the control. The treatment with AA (spray)+Myco gave the largest number of fruit/plant (increasing by 82.76%), but there was no significant difference between treatments SA (drench)+Myco., SA (drench), SA (spray)+Myco. and SA (spray), with 73.68, 70.9, 68.75 and 61.54% respectively. The weight of fruits was positively affected at 84.86% with AA (drench)+Myco application.
Table 9. Effect of SA, AA and mycorrihizin on plant growth parameters under field conditions.
The results in showed that all applied treatments caused a significant increase in the growth parameters compared with the control. Treatment with 150 µg AgNPs after transplanting gave the highest increase (54.44%), but the lowest increase at 39.76 and 36.10% was observed with AgNPs (100 µgm)+Myco and Mycorrihizin, respectively. In the fresh weight of the shoot system, all treatments significantly differed from the control. The treatments AgNPs (150 µg) and AgNPs (100 µg) before transplanting resulted in the highest increase with 78.22 and 77.69%, respectively. All treatments before nematode inoculation caused a significant increase in root and shoot dry weight in comparison with that of the control treatment. In the length of root there was also observed a significant difference between the treatments and the control. The highest increase was observed by AgNPs (150 µg) treatment before transplanting (67.44%). The applied treatments caused insignificant differences in the weights of root and number of fruits in comparison with those of the control treatment. The weight of fruit significantly differed between treatments and the control, in which the best treatment was AgNPs (150 µg) before transplanting, accounting for an 88.82% increase.
As shown in , all treatments increased the plant height, the fresh weight and dry weight of the shoot system compared with the control. The most potent treatment was AgNPs 150µg + AA (drench), resulting in the greatest increasing by 55.06 80.00, and 75.89%, respectively. All treatment variants significantly differed from the control, and the AgNPs150µg + SA (drench) treatment increased the root length with 66.65%, whereas the lowest increase (25.66% and 19.23%) was recorded following Mycorrihizin treatment and AgNPs (150 µg)+SA (spray) treatment, respectively.
Determination of enzyme activity
Regarding the antioxidant enzymes activity, the activity of phenylalanine ammonia-lyase (PAL), polyphenol oxidase (PPO) and peroxidase (POX) in healthy plants were (0.0008, 0.0004, and 0.00007), (0.000144, 0.000063 and 0.000042) and (0.301, 0.534 and 0.262) unit/mg protein/min in shoots and roots on day 30, 45 and 60, respectively (–C).
Figure 5. Activities of (A) poly phenol oxidase (PPO), (B) peroxidase (POX) enzymes and (C) phenylalanine ammonia-lyase (PAL) in the roots of eggplants.
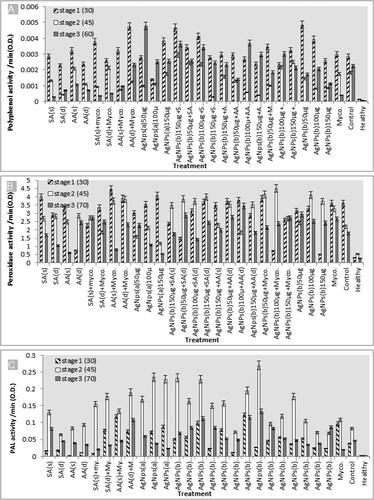
Nematode infection significantly increased such enzymes activities PAL, PPO, and POX with (0.037, 0.082 and 0.046), (0.002844,0.001809 and 0.00225) and (3.624, 2.19 and 1.785 unit/mg protein/min in shoots and roots) after infection stage at 30, 45 and 60 day in that order.
The antioxidant enzyme activities were increased in all treatments on day 30 and 60 of infection (). The lowest rate was observed following the mycorrihizin treatment with PPO enzyme; while the highest rate was observed following treatment by 50 µg AgNPs (during transplanting), 150 µg AgNPs(during transplanting)+SA (spray), and AA (drench)+Myco on day 30 and 50 µg AgNps (before transplanting) on day 60 after infection. Noticeably, the POX enzyme activity showed the highest increase in all treatments during all stages of growth on day 30, 45 and 60 after infection, with the highest activity recorded following treatment with 100 µg AgNPs (during transplanting)+Myco on day 45 and AA (spray)+Myco on day 30 after infection (). The activity of PAL enzyme showed the highest increase on day 45 after infection after 150 µg AgNps (during transplanting)+AA (drench) treatment ().
Real-time PCR (Q-PCR)
Real-time PCR was performed on the treated plants to examine the plant response based on the results obtained by the 26 antinematode treatments: SA (spray), SA (drench), AA (spray), AA (drench), Mycorrihizin SA (spray)+Myco, SA (drench)+Myco, AA (drench)+Myco, AA (spray)+Myco, AgNP (during transplanting)50 µg, AgNP (during transplanting)100 µg, AgNp (during transplanting)150 µg, AgNP (150 µg) (drench)+SA (spray), AgNP (drench)+SA (spray)50 µg, AgNP (drench)+SA (drench)100 µg, AgNP (drench)+SA (drench)150 µg, AgNP (drench)+AA (spray)150 µg, AgNp (drench)+AA (drench)50 µg, AgNp (drench)+AA (drench)100 µg, AgNp (drench)+AA (drench)150 µg, AgNP + Myc AgNP + Myc, AgNP + Myc, AgNP 50 µg, AgNP 100 µg and AgNP 150 µg. The plant response toward either the pathogen or treatments could be examined through the study of the plant defense system and the relevant gene expression levels in the tested plants compared with the control treatment. Here in this study, the expression levels of three genes encoding defense enzymes, Phenylalanine ammonia-lyase (PAL), Polyphenol oxidase (PPO) and Peroxidase (POX), were used as endpoints to demonstrate the plant pathogen–treatment response. Showed high expression level of the gene encoding PPO in SA (drench)+Myco, AA (drench), AgNP150µg and AgNP + Myc treatment respectively (). The PAL gene () showed high expression levels in all treatments except AA (drench), SA (drench)+Myco, AA (drench)+Myco, AA (spray)+Myco treatments. The POX gene expression level was high in the plants infected with M. javanica and treated with SA (spray)+Myco, AgNPs (during transplanting) 100 µg, AgNP (drench)+SA (drench)100 µg ().
Figure 6. Quantitative Real-Time Polymerase Chain Reaction (qRT-PCR) validation of (A) PPO, (B) PAL, and (C) POX relative gene expression levels in roots of eggplant inoculated with M. javanica and treated with SA spray, SA drench, AA spray, AA drench, Myco., SA (spray)+Myco, SA (drench)+Myco., AA (spray)+Myco., AA (drench)+Myco., AgNPs before transplanting (50 µg), AgNPs before transplanting (100 µg), AgNPs before transplanting (150 µg), AgNPs (150 µg)+SA (spray), AgNPs (50 µg)+SA (drench), AgNPs (100 µg)+SA (drench), AgNPs (150 µg)+SA (drench), AgNPs (150 µg)+AA (spray), AgNPs (50 µg)+AA (drench), AgNPs (100 µg)+AA (drench), AgNPs (150 µg)+AA (drench), AgNPs (50 µg)+Myco., AgNPs (100 µg)+Myco., AgNPs (150 µg)+Myco., AgNPs (50 µg) during transplanting, AgNPs (100 µg) during transplanting, AgNPs (150 µg) during transplanting, compare with control. The expression level of the target genes was normalized relative to the actin rRNA gene and relative expression of untreated control plants at each time. Each value represents mean ± SE (n = 3).
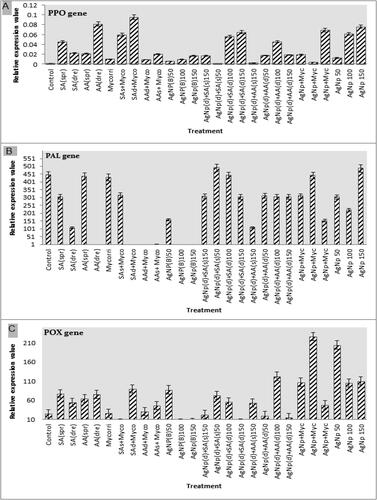
Discussion
Plant pathogen nematodes including root–knot nematodes are involved in considerable losses of the most important vegetable crops including eggplant. The results of the test of pathogenicity showed that the eggplant cv. Classic infested with Meloidogyne javanica was highly susceptible to M. javanica. The plants progressively developed symptoms at all the stages of plant growth with an increase in the galls. These observations were evidence that M. javanica is a potential pathogen for this variety. This result is in agreement with Karajeh and Mohawesh [Citation34], who used the eggplant cv. ‘Classic’, a cultivar susceptible to M. javanica, for cropping in field experiments.
The AgNPs were characterized using UV–Viz Spectroscopy at wavelength of 403 nm. Several researchers have observed absorption maxima of colloidal silver solution at wavelength between 397 and 440 nm, which is assigned to surface plasmon of various metal nanoparticles. The fluorescence spectrum showed a broad emission peak of AgNPs at 403 nm. In agreement with aforementioned reports, the absorption peak at 403 nm is possibly due to the excitation of longitudinal plasmon vibrations and formation of quasi-linear superstructures of nanoparticles [Citation35]. With respect to the tested AgNPs, it was observed that the reduction in juveniles and eggs hatching were clearer with all the various concentrations of AgNPs compared to the control ones in the bioassay performed in vitro.
In the present study, the application of chemically synthesized AgNPs alone or in combination with SA, AA and Mycorrihizin to M. javanica infected eggplant seedlings c.v. Classic in open-field conditions significantly suppressed the nematode population and, in return, enhanced the plant growth parameters. Application of 50, 100 or 150 µg AgNPs (before transplanting) was highly toxic to the nematodes, killing almost 100% of the number of eggs and number of galls/root system compared with the untreated control
In our study, SA (drench)+Mycorrihizin was the most effective treatment and reduced both the number of galls and the number of eggs/root system to zero compared with the untreated infected group (control). These results are in agreement with a previous report in tomato plants [Citation36] in which SA applied as a 50 μmol/L seed treatment caused 95%, and as a soil drench 78%, reduction in the number of egg masses (numbers of galls were affected to a lesser extent). In addition, Mostafa Nezhad et al. [Citation37] also found that soil drench and spraying tomato plants with SA significantly reduced the diameter of M. javanica galls and the numbers of galls and egg masses; the treatments also increased the activity of enzymes and the phenolic compounds content. Mukherjee et al. [Citation38] reported that root dip in SA solutions or SA sprayed onto leaves of tomato plants could induce some resistance to M. incognita and improve plant growth, in addition to other studies [Citation39–41].
This synchronized with enhancement in plant growth parameters and fruit quality of the treated plants and an increase in plant height, length, roots/shoot dry and fresh eggplant weights. These findings are in agreement with previous studies [Citation42, Citation43], which found that solutions of some organic and/or amino acids suppressed the numbers of second stage juveniles, females, egg masses, root galls of M. incognita and M. javanica root–knot nematodes on other hosts.
Our results showed enhancement of PAL, POX and PPO enzyme activity (qualitative determination) and gene expression (quantitative) analysis in eggplant plants with AgNP, SA, AA, Mycorrihizin treatment, or combinations between them. The PPO enzyme activity was higher on day 30 and day 60 after infection, than on day 45 following applications with AgNPs (during transplanting)50µg, AgNPs (50 µg)150µg SA (spray), and AA (drench) Myco and AgNPs (before transplanting)50µg treatments. The POX enzyme activity showed the highest increase in all treatments during all stages of growth (on day 30, 45 and 60 after infection). The activity of PAL enzyme increased noticeably only in treatments at 45 day stage after infection, with the highest increase achieved by AgNPs (during transplanting)150µg + AA (drench) treatment. Researchers have shown increasing interest in inducing resistance in plants via chemical inducers and organic materials. Application of SA has been shown to activate SAR-associated genes in tobacco [Citation44], tomato[Citation45] and parsley [Citation46]. Also, our result is in agreement with the understanding that salicylic acid plays a critical signaling role in the activation of plant defense responses after pathogen attack [Citation47]. The mode of action of salicylic acid is thought to involve enhancement of defense mechanisms in the plant tissue [Citation48].
Conclusions
In this study, all tested nanoparticles treatments or organic inducers applied singly or in combination impaired gall formation, reduced the eggmasses on these galls and the juveniles recovered from the soil, as compared with the untreated control with few exceptions. An important finding from this study revealed that all tested inducers had positive effects on plant growth, yield and fruit quality of eggplant plants grown under field conditions through enhancement of the immune defense system.
Disclosure statement
The authors do not have any relevant financial or non-financial competing interests.
Data availability
All data that support the findings reported in this study are available from the corresponding author upon reasonable request.
References
- Tindall HD. Commercial vegetables growing. London: Oxford University Press; 1978, p. 129.
- Usman A, Siddiqui MA. Effect of some fungal strains for the management of root–knot nematode (Meloidogyne incognita) on eggplant (Solanum melongena). J Agric Technol. 2012;8(1):213–218.
- Sasser JN. Root–knot nematodes a global menace to crop production. Plant Dis. 1980;64(1):36–41.
- Osman HA, Youssef MMA, El-Ginidi AY, et al. Effect of salicylic acid and Pseudomonas flourescens against Meloidogyne incognita in eggplant using split–root technique. Pak J Nematol. 2012;30(2):101–113.
- Hassan MEM, Zawam HSS, El-Nahas EM, et al. Comparative study between silver nanoparticles and two nematicides against Meloidogyne incognita on tomato seedlings. Plant Pathol J. 2016;15(4):144–151.
- Abid M, Zaki MJ, Khan MK, et al. Use of marine algae for the management of root–knot nematode (Meloidogyne javanica) in okra and tomato plant. Int J Phycol Phycochem. 2005;1:187–192.
- Luc EJ, Wenjing P, Robin M, et al. Effects of formulation and host nematode density on the ability of in vitro-produced Pasteuria endospores to control its host Belonolaimus longicaudatus. J Nematol. 2010;42(2):87–90.
- Crow WT, Luc JE, Giblin-Davis RM. Evaluation of EconemTM, a formulated Pasteuria sp. Bionematicide, for management of Belonolaimus longicaudatus on gulf course turf. J Nematol. 2011;43:101–109.
- Osman HA, Youssef MMA, El-Ginidi AY, et al. Effect of abiotic resistance inducers, ɣ-amino-n-butric acid (GABA), ascorbic acid and chitosan on certain enzyme activities of eggplant inoculated with root–knot nematode Meloidogyne incognita. Arch Phytopathol Plant Protect. 2013;46(15):1857–1863.
- Hagag ESF, Taha NAA, Hafez YM. Control of root–knot nematode (Meloidogyne incognita) on eggplant plants using biotic and abiotic inducers of resistance. Egypt J Biol Pest Control. 2016;26(2):269–275.
- Ghareeb YR, Adss AI, Bayoumi SR, et al. The nematicidal potentiality of some algalextracts and their role in enhancement the tomato defense genes against rootknot–nematodes. Egypt J Biol Pest Control. 2019;29(1):53.
- Sharma IP, Sharma AK. Application of arbuscular mycorrihiza for managing root–knot disease in tomato (Lycopersicon esculantum) under glass house conditions in Pantnagar. Afr J Microbiol Res. 2015;9(7):463–468.
- Cromwell WA, Yang J, Starr JL, et al. Nematicidal effects of silver nanoparticles on root–knot nematode in Bermuda grass. J Nematol. 2014;46(3):261–266.
- Abdellatif KF, Hamouda RA, El-Ansary MSM. Green nanoparticles engineering on root–knot nematode infecting eggplants and their effect on plant DNA modification. Iran J Biotechnol. 2016;14(4):250–259.
- Ayoub SM. Plant nematology an agriculture training aid. Scrmento, California, USA: Nema. Aid. Publications; 1980. p. 195.
- Taylor AL, Netscher C. An improved technique for preparing perineal patterns of Meloidogyne spp. Nematology. 1974;20(2):268–269.
- Eisenback JD, Hirschmann H, Sasser JN, et al. A guide to the four most common species of root–knot nematodes (Meloidogyne spp.) with a pictorial key. A cooperative publication of the department of plant pathology and genetics. North Carolina: North Carolina State University and the United States Agency for International Development Raleigh; 1981.
- Hussey RS, Barker KR. Comparison of methods for collecting inocula of Meloidogyne spp. including a new technique. Plant Dis Rep. 1973;57:1025–1028.
- Lopez IA, Gomez I. Photoinduced shape transformation from nanospheres to silver triangular nanoprisms and nanodisks: citrate ion concentration and stirring effects. Rev Mex Fis. 2012;58:289–292.
- Suh JS, DiLella DP, Moskovits M. Surface-enhanced Raman spectroscopy of colloidal metal systems: a two dimensional phase equilibrium in p-aminobenzoic acid adsorbed on silver. J Phys Chem. 1983;87(9):1540–1544.
- Kim SC, Adesogan AT, Arthington JD. Optimizing nitrogen utilization in growing steers fed forage diets supplemented with dried citrus pulp. J Anim Sci. 2007;85(10):2548–2555.
- Sharma R, Bisen DP, Shukla U, et al. X-ray diffraction: a powerful method of characterizing nanomaterials. Recent Res Sci Technol. 2012;4(8):77–79.
- Abramoff MD, Magalhães PJ, Ram SJ. Image processing with image. J Biophoton Int. 2004;11(7):36–41.
- Seham M, Hamed A, Mostafa MA, et al. Biosynthesis of silver and silver chloride nanoparticles by Parachlorella kessleri SAG 211-11 and evaluation of its nematicidal potential against the root–knot nematode Meloidogyne incognita. Aust J Basic Appl Sci. 2016;10(18):354–364.
- Safdar H, Javed N, Aleem KS, et al. Control of Meloidogyne incognita (Kofoid and White) chitwood by cadusafos (Rugby)® on tomato. Pak J Zod. 2012;44(66):1703–1710.
- Barker KR. Nematode extraction and bioassay. In: Barker KR, Carter CC, Sasser JN, editors. An advanced treatise on meloidogyne, vol 11: methodology. Raleigh, North Carolina, USA: North Carolina State University Graphics; 1985. p. 19–35.
- Maxwell DP, Beteman DF. Changes in the activities of some oxidase in extracts of Rhizoctonia infected bean hypocotyls in relation to lesion maturation. Phytopathology. 1967;57:132–136.
- Allam AI, Hollis JP. Sulphide inhibition of oxidase in rice roots. Phytopathology. 1972;62(6):634–639.
- Srivastava SK. Peroxidase and poly phenol oxidase in Brassica juncea plants infected with Macrophomina phaseolina (Tassai) Goid. and their implication in disease resistance. 1987;120(3):249–254.
- Gomez KA, Gomez A. Statistical procedures for agricultural research. 2nd ed.New York: Wiley; 1984.
- Duncan D. Multiple ranges and multiple F test. Biometrics. 1955;11(1):1–42.
- Starr RJ, Yang W, Yan Y, et al. Expression of phenylalanine ammonia lyase genes in maize lines differing in susceptibility to Meloidogyne incognita. J Nematol. 2014;46(4):360–364.
- Jogaiah SM, Abdelrahman LP, Shin-ichi I. Characterization of rhizosphere fungi that mediate resistance in tomato against bacterial wilt disease. J Exp Bot. 2013;64(12):3829–3842.
- Karajeh M, Mohawesh O. Root–knot nematode (Meloidogyne javanica)-deficit irrigation interactions on eggplant cropped under open field condition. J Horticult Res. 2016;24(1):73–78.
- Raza MA, Kanwal Z, Rauf A, et al. Size- and shape-dependent antibacterial studies of silver nanoparticles synthesized by wet chemical routes. Nanomaterials (Basel). 2016;6(4):74.
- Moslemi F, Fatemy S, Bernard F. Inhibitory effects of salicylic acid on Meloidogyne javanica reproduction in tomato plants. Span J Agric Res. 2016;14(1):e1001.
- Mostafanezhad H, Sahebani N, Nourinejhad Zarghani S. Control of root–knot nematode (Meloidogyne javanica) with combination of Arthrobotrys oligospora and salicylic acid and study of some plant defense responses. Biocontrol Sci Tech. 2014;24(2):203–215.
- Mukherjee A, Babu SS, Mandal F. Potential of salicylic acid activity derived from stress-induced (water) tomato against Meloidogyne incognita. Arch Phytopathol Plant Prot. 2012;45(16):1909–1916.
- Molinari S. Effect of salicylic acid on Meloidogyne–tomato interaction. Proc. of the 11th Congress of the Mediterranean Phytopathological Union., September 17–20, Evora. Portugal. 2001a. 412–413.
- Molinari S. Inhibition of H2O2-degrading enzymes in the response of Mi-bearing tomato to root–knot nematodes and salicylic acid treatment. Nematol Medit. 2001b;29:235–239.
- Nandi B, Sukul NC, Babu SPS. Exogenous salicylic acid reduces Meloidogyne incognita infestation of tomato. Allelopathy J. 2000;7:285–288.
- Nandi B, Sukul NC, Banerjee N. Salicylic acid enhances resistance in cowpea against Meloidogyne incognita. Phytopathol Mediterr. 2002;41:39–44.
- Ghareeb RY, Alfy H, Fahmy AA, et al. Utilization of Cladophora glomerata extract nanoparticles as eco-nematicide and enhancing the defense responses of tomato plants infected by Meloidogyne javanica. Sci Rep. 2020;10(1):19968
- Fraissinet-Tachet L, Baltz R, Chong J, et al. Two tobacco genes induced by infection, elicitor and salicylic acid encode glucosyl transferases acting on phenylpropanoids and benzoic acid derivatives, including salicylic acid. Fed Eur Biochem Soc Lett. 1998;437(3):319–323.
- Ding F, Dokholyan NV, Buldyrev SV, et al. Direct molecular dynamics observation of protein folding transition state ensemble. Biophys J. 2002;83(6):3525–3532.
- Thulke OU, Conrath U. Salicylic acid has a dual role in the activation of defence-related genes in parsley. Plant J. 1998;14(1):35–42.
- Klessig DF, Durner J, Noad R, et al. Nitric oxide and salicylic acid signaling in plant defense. Proc Natl Acad Sci USA. 2000;97(16):8849–8855.
- Canet JV, Canet A, Dobon F, et al. Resistance and biomass in Arabidopsis: a new model for salicylic acid perception. Plant Biotechnol J. 2010a;8(2):126–141.