Abstract
Sugar metabolism is among the most important metabolic processes in soybean; it not only affects growth and development, but also plays a role in drought resistance. As a plant biological regulator, exogenous melatonin is assumed to regulate sugar metabolism. Here, physiological and transcriptomic approaches were used to determine the effects of melatonin on physiological indices and key genes and pathways associated with soybean sugar metabolism under drought stress. In plants subjected to drought stress, melatonin treatment increased dry matter accumulation, reduced malondialdehyde content, and increased the content of metabolized sugars in leaves, thereby enhancing soybean yield. Transcriptome analysis revealed that the genes differentially expressed in plants subjected to melatonin treatment and drought stress were enriched in the KEGG “sucrose and starch metabolism” pathway related to glucose metabolism. Among the differentially expressed genes, we identified 12 significantly altered genes associated with glucose metabolism that played roles in regulating the activities of glucose metabolism-related enzymes. Collectively, our results indicated that melatonin can enhance the output of glucose metabolites and tolerance of soybean subjected to drought stress by regulating the genes associated with glucose metabolism and activation of the related enzymes. Our findings provide a detailed theoretical basis for analyzing the regulatory effects of melatonin on soybean sugar metabolism under conditions of drought stress.
Introduction
Soybean (Glycine max) is an important source of edible oil and protein worldwide [Citation1]. As in most plant species, glucose metabolism plays important roles during the entire life of soybean plants, since glucose functions as both a nutrient and a regulatory molecule. Glucose metabolism plays pivotal roles in the complex regulatory network of hormones, the generation of reactive oxygen species (ROS), signal transduction, and scavenging systems [Citation2]. Moreover, it affects the development and production of soybean in seed form, along with growth and development processes. Sugar metabolic processes also determine the conversion of photosynthetic assimilates to grain carbohydrates, and thereby directly influence grain quality and yield [Citation3]. Drought is among the most common forms of abiotic stress [Citation4], and has the potential to adversely affect the synthesis and transport of glucose metabolites and thus reduce glucose production [Citation5]. It has been reported that drought stress can promote changes in plant metabolism, thereby directly inhibiting plant growth and yield [Citation6]. Mild drought stress typically induces increases in plant sucrose phosphate synthase activity, which may, in turn, increase the accumulation of sucrose [Citation7] and promote the activity of glucose metabolism-related pathways to alleviate the damage caused by drought. However, prolonged periods of drought predictably cause more pronounced damage to soybean. For example, Ohashi et al. [Citation8] found that long-term drought could reduce the photosynthetic capacity of soybean leaves, thereby inhibiting the growth and related metabolism of soybean. When the conventional endogenous drought-resistance defense mechanisms of plants are insufficient to resist sudden adversity, the exogenous application of selected plant growth regulators can serve as an effective approach to ensure adequate crop growth.
Plant growth regulators are widely recognized as among the most important means of enhancing the drought resistance of crops [Citation9]. In this regard, progress has been made in recent years in the study of the effects of melatonin on plant drought resistance. Under drought stress, the application of melatonin can significantly promote the activity of soluble starch synthase in cotton, increase carbohydrate content, and improve the activity and germination rate of cotton pollen [Citation10]. In addition, it reduces oxidative damage to maize, inhibits chlorophyll degradation and improves photosynthetic rate and PSII reaction centers and quantum yields [Citation11,Citation12]. However, although melatonin is known to enhance the ability of plants to resist drought by regulating complex biological and molecular pathways [Citation13], there have, to date, been comparatively few studies that have undertaken physiological, biochemical or transcriptomic analyses of the effects of exogenous melatonin application on the regulation of glucose metabolism associated with the seed bloating stage of soybean plants subjected to drought stress. Thus, in this study, we sought to gain insights into the effects of melatonin on soybean sugar metabolism with respect to the physiological and biochemical changes under drought stress, and the associated molecular regulatory mechanisms. To this end, we adopted physiological and transcriptomic approaches to examine the effects of exogenously applied melatonin on the glucose metabolism of soybean drum grain in plants subjected to drought stress. We also assessed the changes in related indices, and at the transcriptome level, analyzed sugar metabolic pathways and the expression of related genes.
Materials and methods
Plant materials and determination methods
This study was conducted from 2018 to 2019 at the National Coarse Cereals Center, Daqing City, Heilongjiang Province, China (124°19′–125°12′E, 45°46′–46°55′N). Seeds of the soybean cultivar Suinong 26 were sown in a 1:1 mixture of vermiculite and perlite contained in plastic basins (33 × 30 cm; 8 seeds per basin). From the time of seeding until seedling emergence, the containers were watered daily with Hoagland solution, and twice daily after seedling emergence. At 5-day intervals, the containers were irrigated with distilled water to prevent the accumulation of salt and vermiculite and perlite. At the cotyledon stage, three seedlings with moderate growth were selected from each container and retained for subsequent experiments. Upon reaching the bulging stage, the plants were assigned to either a normal water supply group (control group) or a drought stress group. The relative soil water content (RSWC) of the control group was maintained at 80%, whereas that of the drought group was maintained at 45%. Half of the plants in the drought stress group were sprayed with 45 mL of a 100 µmol·L−1 melatonin solution for three consecutive days [Citation1], whereas the remaining plants in the treatment group were sprayed with distilled water. The weight of each basin was measured daily to ensure that the set RSWC limit was maintained, and samples were collected on the third day after spraying. Thus, the following three treatments were applied: (1) control (WW): full watering (80%) and spraying with distilled water; (2) drought (D): drought stress (45%) and spraying with distilled water; and (3) D + M: drought stress (45%) and spraying of melatonin. The experiment was conducted according to a randomized complete design with three replicates for each treatment. At 72 h after melatonin application, transcriptome and physiological samples were taken, leaf samples were collected from all treated plants, placed in liquid nitrogen, and stored in a refrigerator at −80 °C until subsequent analysis.
Determination of the dry and fresh weights and yield of plant leaves
After the soybean plants had been collected and washed, the harvested leaves were placed in an oven at 80 °C and dried to a constant weight, which was determined using an analytical balance. Yield was measured at the maturity stage, with the yield indicators, such as pod number per plant, grain number per plant, 100-grain weight per plant, and grain weight per plant at maturity, which were determined based on measurements obtained from 15 plants subjected to each treatment.
Determination of plant malondialdehyde content and relative electrolyte leakage
The content of malondialdehyde (MDA) in plants was determined using the thiobarbituric acid method [Citation14], and the method described by Cao et al. [Citation1]. was used to determine relative electrolyte leakage.
Determination of soluble sugar, glucose, fructose, sucrose and starch contents
The contents of sucrose, fructose and glucose in leaves were determined using the method described by Tang [Citation15] and Li [Citation16], and leaf starch contents were analyzed based on anthrone colorimetry [Citation17].
Total RNA isolation and transcriptome analysis
RNA was extracted from samples collected from nine soybean plants subjected to the three experimental conditions of normal water supply (WW), drought stress (D), and simultaneous exogenous melatonin treatment (D + M) under drought stress, using an EasySpinPlus kit (Aidlab, Beijing, China) according to the manufacturer’s instructions. A total of nine RNA-seq libraries were prepared and sequenced, as described previously by Lei et al. [Citation18]. The raw data were deposited in the National Center for Biotechnology Information (NCBI) sequence read database, under the registration number PRJNA576585 (https://www.ncbi.nlm.nih.gov/). After processing the original reads using an internal Perl script, the HISTAT protocol [Citation19] was used to align the reads with the published soybean genome. The HTseq software (version 0.6.1) was then used to calculate the read number of each gene [Citation20], and gene expression levels were determined as the number of fragments per kilo base per millionth transcript [Citation21]. A significant false discovery rate-adjusted P-value (FDR) of <0.05 was used as an empirical parameter to identify differentially expressed genes, as described previously [ Citation22]. In addition, we performed gene ontology and Kyoto Encyclopedia of Genes and Genomes (KEGG) enrichment analyses using the “cluster analysis” package [Citation23] and BLAST software [Citation24], respectively.
Quantitative real-time fluorescence PCR analysis
To further confirm the transcriptome results, all samples were analyzed using quantitative reverse transcription-polymerase chain reaction (qRT-PCR). The RNA samples used for transcriptome analysis were retrotranscribed using a PrimeScript™ RT kit according to the manufacturer’s instructions. qRT-PCR analysis was performed in a real-time PCR system using TBGreen® PremixexTaq™II and specific primer pairs, the sequences of which are listed in . Soybean GAPDH was used as the reference gene, and gene expression was determined relative to that of the GAPDH gene using the 2-ΔΔCt method.
Table 1. qRT-PCR primers.
Determination of enzyme activity related to glucose metabolism
The activities of sucrose phosphate synthase and sucrose synthase were determined according to the methods described by Li et al. [Citation25]. β-Amylase, acid invertase and neutral invertase were determined according to the method described by Du et al. [Citation26], and sucrose invertase was determined according to the procedure by Tomlinson et al. [Citation27].
Statistical analysis
Data obtained were expressed as means and standard deviations (SD) and analyzed using the SPSS17.0 software (SPSS Inc., Chicago, IL, USA). Statistical significance between groups was determined using one-way analysis of variance, followed by Duncan’s multiple-range test. Differences were considered statistically significant at a p < 0.05 level.
Results
Effects of exogenous melatonin treatment on leaf dry and fresh weights of soybean under drought stress
The leaf dry weights of drought-stressed soybean plants were found to be significantly lower in plants subjected to treatment D than in those receiving the WW treatment (by 9.92%, p < 0.05, ). In contrast, compared with those receiving treatment D, the plants receiving the D + M treatment showed a 2.18% increase in leaf dry weight, although this difference was not statistically significant (). Similarly, the leaf fresh weight was significantly higher in plants receiving the WW treatment than in those under drought stress treatments D and D + M, with reductions of 62.70% and 52.36%, respectively (p < 0.05, ). Moreover, the D + M-treated plants were found to have significantly higher fresh weights (27.71%, p < 0.05) than those receiving treatment D.
Figure 1. Effects of exogenous melatonin on dry (A) and fresh (B) weight of soybean leaves under drought stress. WW: normal water supply; D, drought stress treatment; D + M, drought stress treatment + melatonin treatment. These values are the average of three replicates of three independent experiments. The difference between different treatments was significant (p < 0.05).
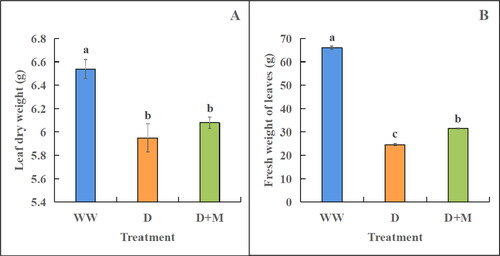
Effects of exogenous melatonin treatment on electrolyte leakage and MDA content in the leaves of soybean plants under drought stress
In soybean plants subjected to drought stress, the electrolyte leakage of leaves was found to be significantly higher than that in the WW group plants (by 42.52%, p < 0.05, ). In contrast, compared with the D group plants, the D + M-treated plants showed a significant reduction of 14.75% in electrolyte leakage (p < 0.05). Similarly, the MDA contents in the leaves of plants subjected to treatments D and D + M were significantly higher than those in the WW group plants, with significant increases of 27.20% and 18.37%, respectively (p < 0.05) (). The levels were, however, significantly lower in the D + M-treated plants (by 10.81%, p < 0.05).
Figure 2. Effects of exogenous melatonin treatment on electrolyte leakage (A) and MDA content (B) of soybean leaves under drought stress. WW: normal water supply; D, drought stress treatment; D + M, drought stress treatment + melatonin treatment. These values are the average of three replicates of three independent experiments. The difference between different treatments was significant (p < 0.05).
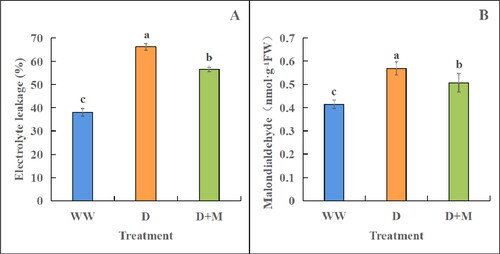
Effects of exogenous melatonin treatment on glucose metabolism-related indices in the leaves of soybean plants under drought stress
We found that the fructose content in the leaves of soybean receiving treatment D was significantly higher (by 44.59%, p < 0.05) than that in the WW group plants (). Compared with the D and WW group plants, those receiving the D + M treatment also had significantly higher fructose contents (by 24.31% and 38.90%, respectively, p < 0.05). In contrast, we detected reductions in the contents of sucrose, starch and glucose in plants subjected to drought stress. Compared with the plants in the WW group, the contents of sucrose, starch and glucose in plants receiving treatment D decreased by 2.90%, 24.96% and 9.68%, respectively, among which only the reduction in starch reached the level of significance (p < 0.05). Notably, however, compared with the D group plants, we observed significantly higher contents of sucrose, starch, and glucose (19.57%, 15.92% and 16.37%, respectively) in plants receiving the D + M treatment (p < 0.05).
Figure 3. Effects of exogenous melatonin treatment on glucose content (A), starch (B), sucrose (C) and fructose (D) in soybean leaves under drought stress. WW: normal water supply; D, drought stress treatment; D + M, drought stress treatment + melatonin treatment. These values are the average of three replicates of three independent experiments. The difference between different treatments was significant (p < 0.05).
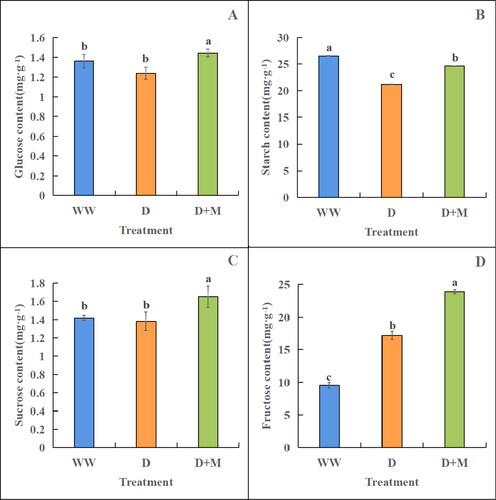
Effects of exogenous melatonin treatment on the activities of sugar metabolism-related enzymes in the leaves of soybean plants under drought stress
Drought stress was observed to significantly reduce the activities of sucrose phosphate synthase, sucrose synthase, acid invertase and neutral invertase in the leaves of plants subjected to treatment D (p < 0.05), by 33.96%, 20.56%, 37.33% and 30.45%, respectively, compared with those in the WW group plants (). Compared with treatment D, the exogenous application of melatonin was found to significantly increase the activities of sucrose phosphate synthase, sucrose synthase and acid invertase in soybean leaves (p < 0.05). The activity of neutral invertase was also increased, albeit non-significantly (). In contrast, drought stress was found to increase the activities of sucrose invertase and β-amylase, with significant increases of 12.43% and 12.18%, respectively, compared with those in the WW group (p < 0.05). Moreover, among the drought-stressed plants, the activities of these two enzymes were higher in the plants receiving exogenous application of melatonin ().
Figure 4. Activity of sucrose phosphate synthase (A), sucrose synthase (B), sucrose invertase (C), β-amylase (D), acid invertase €and neutral invertase (D) in soybean leaves. These values are the average of three replicates of three independent experiments. There were significant differences in the values of different superscripts (p < 0.05).
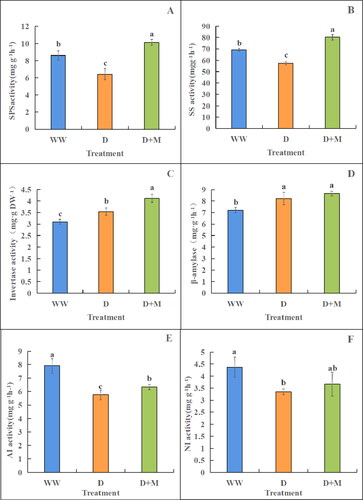
Effects of exogenous melatonin treatment on soybean yield under drought stress
As indices of soybean yield, we undertook determinations of pod number per plant, grain number per plant, 100-grain weight per plant and grain weight per plant, which were all found to be significantly lower in D-treated plants than in those subjected to the WW treatment, by 15.35%, 6.02%, 22.81% and 28.11%, respectively (p < 0.05) (). However, compared with the drought only treatment, exogenously applied melatonin promoted increases of 8.79%, 3.98%, 8.13% and 12.44%, respectively, among which the increases in the number of grains per plant, 100-grain weight per plant, and grain weight per plant were significant (p < 0.05).
Table 2. Effects of melatonin on soybean yield under drought stress.
KEGG enrichment analysis of genes in the leaf transcriptomes of soybean subjected to melatonin treatment under drought stress
We performed KEGG metabolic pathway analysis to assess the biological activities of differentially expressed unigenes. Our results indicated that these differentially expressed genes were involved in the main biochemical metabolic pathways. shows the top 25 pathways, based on P-values > 0.05, among which starch and sucrose metabolism (KO00500) was among the most significantly enriched of the glucose metabolism-related pathways.
Figure 5. Bubble Diagram of KEGG pathway enrichment of differentially expressed genes (DEGs) in WW/D (A) and D/D + M (B) comparison. The eutrophication factor is calculated by dividing the number of DEGs by the cardinal number of any given pathway. The dot size represents the number of genes, and the dot color represents the range of adjusted p value.
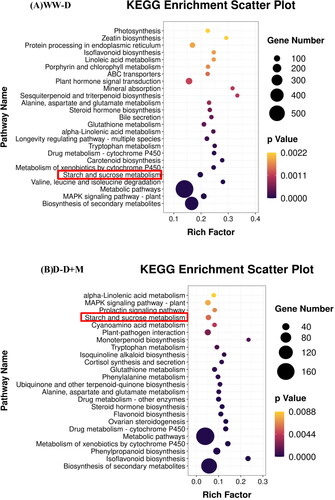
KEGG enrichment of starch and sucrose metabolism pathways in the leaves of soybean plants subjected to melatonin treatment under drought stress
shows the synthetic pathways of starch and sucrose metabolism (KO00500) based on KEGG enrichment analyses, indicating the involvement of the following enzymes: glucosidase [EC:2.4.1.13], sucrose-phosphate synthase [EC:2.4.1.14], trehalose 6-phosphate phosphatase [EC:3.1.3.12], beta-amylase [EC:3.2.1.2], beta-glucosidase [EC:3.2.1.21], glucosidase [EC:3.2.1.21], Beta-fructofuranosidase [EC: 3.2.1.26] and 6-phosphate, trehalose synthase/phosphatase [EC: 2.4.1.15 3.1.3.12].
Expression levels of candidate genes associated with sucrose and starch metabolic pathways and qRT-PCR verification
shows the qRT-PCR verification of 12 genes identified as being differentially expressed under the different treatments: Glyma.09G073600, Glyma.18G108100, Glyma.04G119700, Glyma.17G150100, Glyma.11G129900, Glyma.12G054200, Glyma.09G231500, Glyma.12G005100, Glyma.15G024600, Glyma.16G039300, Glyma.13G278600 and Glyma.17G067800. The qRT-PCR data were consistent with the transcriptome data.
Discussion
Drought stress inhibited normal soybean growth and development, and the changes in morphology and dry matter accumulation were the most direct response to water deficit. Drought stress has previously been reported to inhibit the physiological and biochemical processes in soybean, thereby causing morphological changes [Citation26,Citation28]. In addition, drought conditions can disrupt the carbon balance of plants, resulting in a decline in photosynthetic capacity, metabolic disorders and growth retardation, and thus a serious decline in yield [Citation29]. Zhang et al. [Citation30] found that drought stress can lead to an imbalance of active oxygen metabolism in plants, resulting in a significant increase in membrane lipid peroxidation and MDA content. Melatonin is an important exogenous growth regulator, which can improve the stress resistance of crops following application by soaking seeds [Citation31] and spraying leaves [Citation32]. Studies have shown that melatonin can increase the content of antioxidant enzymes, remove excessive reactive oxygen species, maintain high antioxidant content, enhance the antioxidant capacity of crops, reduce the degree of membrane lipid peroxidation, and alleviate the damage caused by stress to plants [Citation33].
Similarly, in the present study, consistent with the findings of Li et al. [Citation34], we demonstrated that drought stress can inhibit the normal growth of soybean leaves and the accumulation of dry matter, as well as promote increases in leaf electrolyte leakage and MDA content in plants, thereby leading to a significant reduction in the number of pods per plant and 100-grain weight of soybeans, and accordingly reducing yields. Notably, however, we observed significant increases in the dry and fresh weights of leaves sampled from soybean plants treated with melatonin. Moreover, we detected significant reduction in the extent of electrolyte leakage and contents of MDA in vivo, which ultimately contributed to reducing the inhibitory effects of drought stress on yield. These findings are consistent with those reported by Zou et al. [Citation35], who demonstrated the ameliorative effects of melatonin on soybean plants subjected to drought stress.
Most abiotic stress sources can stimulate ROS production, which may lead to oxidative damage, inhibit the metabolic process of photosynthesis, and have adverse effects on crop yield and quality [Citation36–38]. In addition to the recognized classical antioxidant mechanisms, sugar and carbohydrate metabolic enzymes play an important role in antioxidant stress. In this study, we observed that drought stress reduced the sucrose, starch and glucose content in soybean leaves and that plants initiated osmotic adjustment mechanism to increase fructose content. This is in line with the findings of Abdelgawad et al. [Citation39] in maize. In addition, drought stress inhibited sucrose synthase, sucrose phosphate synthase, acid invertase and neutral invertase activity.
However, the exogenous application of melatonin was found to effectively enhance the activities of glucose metabolism-related enzymes (sucrose synthase, sucrose phosphate synthase, sucrose invertase and beta-amylase), acid invertase, and neutral invertase, thereby relieving the inhibitory effects of drought stress on sugar metabolite synthesis. Accordingly, by enhancing the activities of these enzymes, melatonin treatment contributed to increases in soybean sugar, starch and glucose output, thereby further enhancing the contents of soluble sugars and fructose, and alleviating drought-associated damage via augmentation of the soybean osmoregulatory system. These findings are consistent with those obtained by Gupta et al. [Citation40], who assessed the effects of betaine on sugar metabolism in the leaves of wheat plants subjected to long-term drought stress.
Although Bonnefont-Rousselot et al. [Citation41] have previously found that melatonin does not directly eliminate ROS in plants, the authors speculated that this regulator may be effective at enhancing sugar metabolism and production, repairing the membrane damage caused by drought stress, reducing the production of MDA, and rectifying a heightened electrolyte leakage. Collectively, these responses may contribute to a recovery of plant dry matter accumulation, thereby enabling the plants to maintain a stable production ().
Figure 8. Effects of exogenous melatonin on sugar metabolism of soybean under drought stress. Red indicates up-regulated genes and metabolites, green indicates down-regulated genes and metabolites, and blue indicates the composition of up-regulated and down-regulated genes.
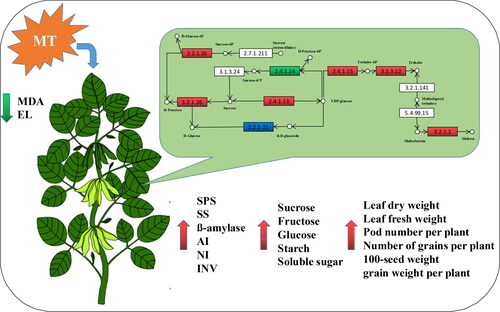
We reasoned that transcriptome analysis would enable us to gain a better understanding of the complex mechanisms of action of the factors assessed in the present study, and we accordingly adopted a transcriptomic approach to analyze the effect of melatonin on the expression of genes in drought-stressed soybean. The results thus obtained can provide an important theoretical basis for elucidating the mechanisms by which exogenous melatonin regulates soybean sugar metabolism under conditions of drought stress. We thus conducted KEGG enrichment analysis of differentially expressed genes, in conjunction with an examination of the biological roles of these genes, and accordingly found the “starch and sucrose metabolism” (KO00500) pathways to be significantly enriched. Moreover, we identified the following differentially expressed allogenes associated with these starch and sucrose metabolism and synthesis pathways (): Glyma.09G073600, Glyma.18G108100, Glyma.04G119700, Glyma.17G150100, Glyma.11G129900, Glyma.12G054200, Glyma.09G231500, Glyma.12G005100, Glyma.15G024600, Glyma 16G039300, Glyma 13G278600 and Glyma 17G067800.
There is still evidence that they play an important role in stress resistance. For example, Glyma.09g073600 and Glyma.18g108100 regulate sucrose-related enzymes to increase resistance to low temperature stress [Citation42]; Glyma.04g119700 regulates maltooligosyltrehalose under salt stress [Citation43]; Glyma.12g054200 is considered to be a factor potentially involved in regulating the salt adaptation of α - galactosidase production [Citation44].
In addition, Baud et al. [Citation45] and Santaniello et al. [Citation46] found that the Arabidopsis thaliana At3G43190 gene homologous to Glyma.09G073600, plays an important role in the regulation of sucrose synthase activity in plant sugar metabolism, whereas Chary et al. [Citation47] found that the At1G68020 homolog of Glyma.04G119700 affects Arabidopsis cellular morphology and plant structure via the activity of trehalose-6-phosphate synthase/phosphatase. Consistent with the findings of the present study, we have also previously established that β-amylase is regulated by the gene At4G17090, which is homologous to Glyma.17G150100 [Citation48]. Our search for Arabidopsis genes homologous to the 12 genes identified in the present study revealed their roles in the regulation of enzymes related to glucose metabolism, which is in line with our present assessment (), and thereby indicates that the direction of this study is correct and the findings reliable. In order to further verify the reliability of our transcriptome data, we performed qRT-PCR to analyze the expression profiles of the starch and sucrose metabolic pathway-related genes, and accordingly confirmed consistency between the qRT-PCR and transcriptome data.
Table 3. Sugar metabolism-related genes and Arabidopsis homologous genes.
Collectively, our experimental results indicate that melatonin is effective in regulating key genes in starch and sucrose metabolic pathways, enhancing the activity of sugar metabolism-related enzymes in soybean leaves, and increasing the accumulation of sugar metabolism-related products in these leaves, thereby contributing to an alleviation of the detrimental effects associated with drought stress ().
Conclusions
Drought stress was found to inhibit the growth and dry matter accumulation of soybean, cause membranous damage via the elevated production of malondialdehyde, and promote electrolyte leakage. However, exogenous application of the regulator melatonin was observed to effectively regulate the expression of sugar metabolism-related genes, enhance the activities of sugar metabolism-related enzymes activity, promote sugar metabolism and production, repair the membrane damage attributable to drought stress, reduce the production of MDA, and rectify electrolyte leakage. Consequently, there was a recovery of plant dry matter accumulation, which enabled soybean plants to maintain a stable yield
Authors’ contributions
Q.B. and L.C. conceived the study and designed and managed the experiments, and performed the trials and collected the data, completed the statistical analysis of the phenotypic data and wrote the paper, Yu Xian Zhang provided the experimental platform and financial support.
Abbreviations | ||
MDA | = | Malondialdehyde |
qRT-PCR | = | Quantitative real-time polymerase chain reaction |
KEGG | = | Kyoto Encyclopedia of Genes and Genomes |
ROS | = | Reactive oxygen species |
EL | = | Electrolyte leakage |
Acknowledgements
The authors thank Cao Liang and Zhang Yuxian for their support and the platform provided by Soybean Research Group of Heilongjiang Bayi Agricultural University.
Disclosure statement
No potential conflict of interest was reported by the authors.
Additional information
Funding
References
- Cao L, Jin X, Zhang Y, et al . Transcriptomic and metabolomic profiling of melatonin treated soybean (Glycine max L.) under drought stress during grain filling period through regulation of secondary metabolite biosynthesis pathways. PLoS One. 2020;15(10):e0239701.
- Bolouri-Moghaddam MR, Roy KL, Xiang L, et al. Sugar signalling and antioxidant network connections in plant cells. Febs J. 2010;277(9):2022–2037.
- Westgate ME, Peterson CM. Flower and pod development in water deficient soybean. J Exp Bot. 1993;44(1):109–117.
- Khazaei Z, Esmaielpour B, Estaji A. Ameliorative effects of ascorbic acid on tolerance to drought stress on pepper (Capsicum annuum L) plants. Physiol Mol Biol Plants. 2020;26(8):1649–1662.
- Getachew M. Influence of soil water deficit and phosphorus application on phosphorus uptake and yield of soybean (Glycine max L.) at Dejen, North-West Ethiopia. AJPS. 2014;5(13):1889–1906.
- Valliyodan B, Nguyen HT. Understanding regulatory networks and engineering for enhanced drought tolerance in plants. Curr Opin Plant Biol. 2006;9(2):189–195.
- Yang J, Zhang J, Wang Z, et al. Activities of starch hydrolytic enzymes and sucrose-phosphate synthase in the stems of rice subjected to water stress during grain Filling. J Exp Bot. 2001;52(364):2169–2179.
- Ohashi Y, Nakayama N, Saneoka H, et al. Effects of drought stress on photosynthetic gas exchange, chlorophyll fluorescence and stem diameter of soybean plants. fluorescence and stem diameter of soybean plants. Biol Plant. 2006;50(1):138–141.
- Peleg Z, Blumwald E. Hormone balance and abiotic stress tolerance in crop plants. Curr Opin Plant Biol. 2011;14(3):290–295.
- Hu W, Cao Y, Loka DA, et al. Exogenous melatonin improves cotton (Gossypium hirsutum L.) pollen fertility under drought by regulating carbohydrate metabolism in male tissues. Plant Physiol Biochem. 2020;151:579–588.
- Li Z, Su X, Chen Y, et al. Melatonin improves drought resistance in maize seedlings by enhancing the antioxidant system and regulating abscisic acid metabolism to maintain stomatal opening under PEG-induced drought. J Plant Biol. 2021:1–14. DOI:10.1007/s12374-021-09297-3
- Guo YY, Li HJ, Liu J, et al. Melatonin alleviates drought-induced damage of photosynthetic apparatus in maize seedlings. Russ J Plant Physiol. 2020;67(2):312–322.
- Sharma A, Wang J, Xu D, et al. Melatonin regulates the functional components of photosynthesis, antioxidant system, gene expression, and metabolic pathways to induce drought resistance in grafted Carya cathayensis plants. Sci Total Environ. 2020;713:136675–136613.
- Kumar G, Knowles NR. Changes in lipid peroxidation and lipolytic and free-radical scavenging enzyme activities during aging and sprouting of potato (Solanum tuberosum) seed-tubers. Plant Physiol. 1993;102(1):115–124.
- Tang ZC. Experimental guide of modern plant physiology. Beijing: Science Press; 1999.
- Li HS. Principles and Techniques of Plant Physiological Biochemical Experiment. Beijing: Higher Education Press; 2000.
- Gao JF. Experimental guide for plant physiology. Beijing: Higher Education Press; 2006.
- Lei Y, Xu Y, Hettenhausen C, et al. Comparative analysis of alfalfa (Medicago sativa L.) leaf transcriptomes reveals genotype-specific salt tolerance mechanisms. BMC Plant Biol. 2018;18(1):35.
- Kim D, Langmead B, Salzberg SL. HISAT: a fast spliced aligner with low memory requirements. Nat Methods. 2015;12(4):357–360.
- Anders S, Pyl PT, Huber W . HTSeq-a Python framework to work with high-throughput sequencing data. Bioinformatics. 2015;31(2):166–169.
- Pertea M, Pertea GM, Antonescu CM, et al . StringTie enables improved reconstruction of a transcriptome from RNA-seq reads. Nat Biotechnol. 2015;33(3):290–295.
- Anders S, Huber W. Differential expression analysis for sequence count data. Genome Biol. 2010;11(10):R106.
- Yu G, Wang LG, Han Y, et al. clusterProfiler: an R package for comparing biological themes among gene clusters. Omics: J Integr Biol. 2012;16(5):284–287.
- Camacho C, Coulouris G, Avagyan V, et al. BLAST+: architecture and applications. BMC Bioinformatics. 2009;10:421.
- Li S, Li Y, Gao Y, et al. Effects of CO2 enrichment on non-structural carbohydrate metabolism in leaves of cucumber seedlings under salt stress. Sci Hortic. 2020;265:109275.
- Du Y, Zhao Q, Chen L, et al. Effect of drought stress on sugar metabolism in leaves and roots of soybean seedlings. Plant Physiol Biochem. 2020;146:1–12.
- Tomlinson KL, McHugh S, Labbe H, et al. Evidence that the hexose-to-sucrose ratio does not control the switch to storage product accumulation in oilseeds: analysis of tobacco seed development and effects of overexpressing apoplastic invertase.. J Exp Bot. 2004;55(406):2291–2303.
- Dong SK, Dong YC, Yang L, et al. Effects of drought stress and re-watering on osmotic adjustment ability and yield of soybean. J Northeast Agric Univ. 2020;27(80(03):3–10.
- Aliche EB, Theeuwen T, Oortwijn M, et al. Carbon partitioning mechanisms in POTATO under drought stress. Plant Physiol Biochem. 2020;146:211–219.
- Zhang M, He S, Zhan Y, et al. Exogenous melatonin reduces the inhibitory effect of osmotic stress on photosynthesis in soybean. PloS One. 2019;14(12):e0226542.
- Nabaei M, Amooaghaie R. Interactive effect of melatonin and sodium nitroprusside on seed germination and seedling growth of Catharanthus roseus under cadmium stress. Russ J Plant Physiol. 2019;66(1):128–139.
- Zheng X, Zhou J, Tan DX, et al. Melatonin improves waterlogging tolerance of Malus baccata (Linn.) Borkh. Seedlings by maintaining aerobic respiration, photosynthesis and ROS migration. Front Plant Sci. 2017;8:483.
- Liu L, Li D, Ma Y, et al. Combined application of arbuscular mycorrhizal fungi and exogenous melatonin alleviates drought stress and improves plant growth in tobacco seedlings. J Plant Growth Regul. 2021;40(3):1074–1087.
- Li H, Wang JQ, Liu Q. Photosynthesis product allocation and yield in sweet potato with spraying exogenous hormones under drought stress. J Plant Physiol. 2020;253:153265.
- Zou JN, Jin XJ, Zhang YX. Effects of melatonin on photosynthesis and soybean seed growth during grain filling under drought stress.Photosynthetica. 2019;57(2):512–520.
- Anwar S, Khalilzadeh R, Khan S, et al. Mitigation of drought stress and yield improvement in wheat by zinc foliar spray relates to enhanced water use efficiency and zinc contents. Int J Plant Prod. 2021. DOI:10.1007/s42106-021-00136-6.
- Chen L, Lu B, Liu L, et al. Melatonin promotes seed germination under salt stress by regulating ABA and GA3 in cotton (Gossypium hirsutum L.). Plant Physiol Biochem. 2021;162(2):506–516.
- Zhang F, Lu K, Gu Y, et al. Effects of low-temperature stress and brassinolide application on the photosynthesis and leaf structure of tung tree seedlings. Front Plant Sci. 2019;10:1767.
- Abdelgawad H, Avramova V, Baggerman G, et al. Starch biosynthesis contributes to the maintenance of photosynthesis and leaf growth under drought stress in maize. Plant Cell Environ. 2020;43(9):2254–2271.
- Gupta N, Thind SK. Foliar application of glycine betaine alters sugar metabolism of wheat leaves under prolonged field drought stress. Proc Natl Acad Sci, India, Sect B Biol Sci. 2019;89(3):877–884.
- Reaction mechanism of melatonin oxidation by reactive oxygen species in vitro. J Pineal Res. 2011;50(3):328–335.
- Bilska-Kos A, Mytych J, Suski S, et al. Sucrose phosphate synthase (SPS), sucrose synthase (SUS) and their products in the leaves of Miscanthus × giganteus and Zea mays at low temperature. Planta. 2020;252(2):23.
- Stracke C, Meyer BH, Hagemann A, et al. Salt stress response of sulfolobus acidocaldarius involves complex trehalose metabolism utilizing a novel trehalose-6-phosphate synthase (TPS)/trehalose-6-phosphate phosphatase (TPP) pathway. Appl Environ Microbiol. 2020;86(24):e01565–20.
- Zhou J, Liu Y, Lu Q, et al. Characterization of a glycoside hydrolase family 27 α-galactosidase from pontibacter reveals its novel salt-protease tolerance and transglycosylation activity. J Agric Food Chem. 2016;64(11):2315–2324.
- Baud S, Vaultier MN, Rochat C. Structure and expression profile of the sucrose synthase multigene family in Arabidopsis. J Exp Bot. 2004;55(396):397–409.
- Santaniello A, Loreti E, Gonzali S, et al. A reassessment of the role of sucrose synthase in the hypoxic sucrose-ethanol transition in Arabidopsis. Plant Cell Environ. 2014;37(10):2294–2302.
- Chary SN, Hicks GR, Choi YG, et al. Trehalose-6-phosphate synthase/phosphatase regulates cell shape and plant architecture in Arabidopsis. Plant Physiol. 2008;146(1):97–107.
- Kaplan F, Guy CL. RNA interference of Arabidopsis beta-amylase8 prevents maltose accumulation upon cold shock and increases sensitivity of PSII photochemical efficiency to freezing stress. Plant J. 2005;44(5):730–743.
- Gaudet P, Livstone MS, Lewis SE, et al. Phylogenetic-based propagation of functional annotations within the Gene Ontology consortium. Brief Bioinform. 2011;12(5):449–462.
- Lao J, Oikawa A, Bromley JR, et al. The plant glycosyltransferase clone collection for functional genomics. Plant J. 2014;79(3):517–529.
- Monroe JD, Storm AR, Badley EM, et al. Amylase1 and β-amylase3 are plastidic starch hydrolases in Arabidopsis that seem to be adapted for different thermal, pH, and stress conditions. Plant Physiol. 2014;166(4):1748–1763.
- Mitsuhashi W, Sasaki S, Kanazawa A, et al. Differential expression of acid invertase genes during seed germination in Arabidopsis thaliana. Biosci Biotechnol Biochem. 2004;68(3):602–608.
- Carter C, Pan S, Zouhar J, et al. The vegetative vacuole proteome of Arabidopsis thaliana reveals predicted and unexpected proteins. Plant Cell. 2004;16(12):3285–3303.
- Sampedro J, Valdivia ER, Fraga P, et al. Soluble and membrane-bound β-glucosidases are involved in trimming the xyloglucan backbone. Plant Physiol. 2017;173(2):1017–1030.
- Escamilla-Treviño LL, Chen W, Card ML, et al. Arabidopsis thaliana beta-Glucosidases BGLU45 and BGLU46 hydrolyse monolignol glucosides. Phytochemistry. 2006;67(15):1651–1660.