Abstract
Antibiotics (ATBs) induce dysbiosis of the gut microbiota by altering the diversity and composition of the microbiota, which mediates the efficacy and toxicity of cancer therapy. The influence of dysbiosis induced by ATB administration on primary resistance to chemotherapy in patients with advanced gastric cancer (GC) has been rarely studied. We evaluated the effect of ATB administration on chemotherapy efficacy in patients with advanced GC. Patients with GC were divided into two groups according to the status of ATB administration: ATB-treated and control groups. Tumor responses, progression-free survival (PFS) and overall survival (OS) were assessed. We found that the incidence of progressive disease in the ATB-treated group was significantly higher when compared with that in the control group that received no ATB treatment (72.73% vs. 29.55%, p = 0.007). In addition, ATB administration was associated with shorter PFS [median PFS: 1.47 vs. 4.97 months, hazard ratio (HR): 2.296, 95% confidence interval (CI): 1.214 − 4.342, p = 0.011] and reduced OS (median OS: 9.97 vs. 13.3 months, HR: 2.101, 95% CI: 1.030 − 4.286, p = 0.041) based on univariate analysis. Subsequent multivariate analysis also indicated that ATB administration was an independent prognostic factor for PFS (HR: 3.361, 95% CI: 1.592 − 7.097, p = 0.001) and OS (HR: 2.280, 95% CI: 1.042 − 4.991, p = 0.039). ATB administration is associated with reduced chemotherapy efficacy and poor prognosis in patients with advanced GC. Modulations in ATB-related dysbiosis and gut microbiota composition improve the clinical outcomes of chemotherapy.
Supplemental data for this article is available online at https://dx.doi.org/10.1080/13102818.2021.1953400 .
Keywords:
Introduction
Gastric cancer (GC) is the fifth most common cancer and third leading cause of cancer mortality worldwide. In China, nearly one million individuals are diagnosed annually [Citation1]. Most patients present with inoperable advanced or metastatic disease with a 5-year survival rate of 5 − 20% and median overall survival (OS) of less than 1 year [Citation2]. The general treatment for advanced GC is a fluoropyrimidine-based and platinum-based combination with or without a third drug, such as docetaxel or epirubicin.
The microbiota participates in host metabolism, immunomodulation, neuronal development, and maintenance of the gut mucosal barrier [Citation3]. Gut bacteria modulate the pharmacological effects of chemotherapeutic agents (5-fluorouracil, cyclophosphamide, irinotecan, oxaliplatin, gemcitabine and methotrexate) through a mechanistic framework as follows: translocation, metabolism, immunomodulation, enzymatic degradation, reduced diversity and ecological variation [Citation4,Citation5]. Antibiotics (ATBs) induce microbiota dysbiosis characterized by the loss of distinct species and expansion of pathogens by initiating changes in bacterial metabolites, disruption of bacterial signals, dysregulation of gut immune cells and dysfunction of systemic immunity [Citation6]. In the present study, we explored the possibility that dysbiosis induced by ATB administration influences primary resistance to chemotherapy in patients with advanced GC.
Subjects and methods
Ethics statement
All procedures were supervised and approved by the Ethics Committee of the Fourth Hospital of Hebei Medical University (2020KS001). Written informed consent was obtained from the participants.
Patients
Patients with advanced GC, including 80 male and 19 female patients who received palliative chemotherapy at the Fourth Hospital of Hebei Medical University between 1 March 2011 and 15 July 2019, were enrolled in this study (). Patients were excluded if they had GC combined with other malignant tumors at the same time. All patients received chemotherapy alone or in combination with other treatments, including radiation therapy, upper gastrointestinal stenting, palliative surgery, or targeted therapy. The palliative surgery mainly refers to intraperitoneal perfusion, palliative gastrectomy and resection or transarterial chemoembolization of liver metastasis.
Table 1. Clinical characteristics of patients.
Medical records of all patients were reviewed to collect data regarding the presence, specific time, class, indications, route and duration of ATB administration (). Patients who were administered ATBs within 30 days after initiating chemotherapy were defined as the ATB-treated group, and the others were defined as the control group. Clinical characteristics including age, sex, tumor location, histological type, performance status of the Eastern Cooperative Oncology Group, detailed treatment regimen and metastatic status were also collected.
Computed tomography and gastroscopy (if necessary) data were reviewed regularly to evaluate the tumor response according to the Response Evaluation Criteria in Solid Tumors version 1.1 [Citation7]. All patients were followed up every three months until death or until the database was closed (15 July 2019).
Data analysis
Clinicopathological features and tumor response were compared using Fisher’s exact test or the Chi-squared test. Progression-free survival (PFS) was defined as the time interval from the initiation of chemotherapy to the date of disease progression (PD). OS was defined as the time interval from the initiation of chemotherapy to the date of death. The PFS and OS curves were estimated using the Kaplan -Meier method and compared using the log-rank test. The Cox proportional hazard model was used to calculate the hazard ratios (HRs) and 95% confidence intervals (CIs) in univariate and multivariate analyses. Subgroup analysis using the univariate Cox proportional hazard model estimated the hazard ratios (HRs) of ATB group versus control group in specific patients subgroups (the HR values of ATB group vs. control group in older patients for instance), and a forest plot was created to better present each prognostic factor’s effect on PFS and OS. All statistical analyses were performed using SPSS statistical software, version 19.0 (IBM Corporation, Armonk, NY). Differences were considered statistically significant at the p < 0.05 level.
Resigults
A total of 99 patients with metastatic GC with the main histological subtype of adenocarcinoma (93.94%) were enrolled in this study (). Of these, 68 (68.69%) patients received a fluoropyrimidine-based combination with platinum therapy, whereas 31 (31.31%) received paclitaxel alone or combined with other drugs, including fluoropyrimidine and platinum (). According to the TNM staging, the patients were mainly distributed in stage IV and a few patients, in stage III, and no differences were found between the ATB and the control group. There was no major statistical difference in the baseline clinicopathological characteristics between the ATB group and control group with the most frequently administered ATB of β-lactam ± inhibitors (, ).
In patients with GC, recent ATB administration was associated with an increased PD rate (72.73% vs. 29.55%, p = 0.007) compared to that in the control group (). In addition, ATB administration was associated with both shorter PFS (median PFS: 1.47 vs. 4.97 months, HR: 2.296, 95% CI: 1.214 − 4.342, p = 0.011) and reduced OS (median OS: 9.97 vs. 13.3 months, HR: 2.101, 95% CI: 1.030 − 4.286, p = 0.041) ( and , ).
Figure 1. The impact of antibiotics administration on the clinical outcome of advanced GC patients: Progression-free survival, PFS (a); Overall survival, OS (b); Tumor response (c), no patients experienced complete response in two groups, Partial response: PR, Stable disease: SD, Progressive disease: PD.
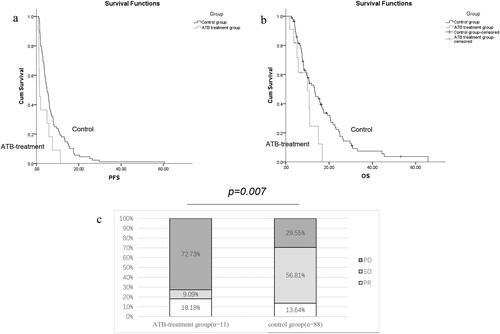
Table 2. Univariate and multivariate for PFS in advanced GC patients.
Table 3. Univariate and multivariate for OS in advanced GC patients.
We next investigated the impact of ATBs on PFS and OS within individual subgroups of patients based on stratified analysis. As noted in , the ATB group displayed a trend of reduced PFS and OS within nearly every subgroup. The interaction within subgroups including gender (p = 0.020), age (p = 0.011), histology (p = 0.012), ECOG performance status (p = 0.003), or targets therapy (p = 0.007) and radiotherapy (p = 0.007) were significantly different referring to PFS. And the interaction within subgroups including histology (p = 0.017) and ECOG performance status (p = 0.015) were significantly different referring to OS.
We finally performed multivariate analysis including all clinical characteristics, and ATB administration was identified as an independent prognostic factor for PFS (HR: 3.361, 95%CI: 1.592 − 7.097, p = 0.001) and OS (HR: 2.280, 95%CI: 1.042 − 4.991, p = 0.039) at statistically significant levels ( and ).
Discussion
We investigated the potential clinical impact of ATB use on chemotherapy efficacy in patients with advanced GC, and we found that ATB administration was associated with a decrease in PFS (1.47 vs. 4.97 months) and OS (9.97 vs. 13.3 months) compared with the control group. These decreases are explained at least partly by the higher PD rate in the ATB group. The subgroups analysis including gender, age, histology, ECOG performance status, or targets therapy and radiotherapy displayed a significant interaction for PFS. In addition, subgroups analysis including histology and ECOG performance status displayed a significant interaction for OS. The subgroup analysis further confirmed the deleterious effect of ATB on chemotherapy. These results are consistent with our previous observation that the antibiotic administration was associated with reduced chemotherapy efficacy and poor prognosis in patients with esophageal cancer [Citation8]. Almost 10% of patients with cancer undergoing chemotherapy require ATB treatment due to the immunosuppressive nature of malignancies and lymphodepletion induced by anticancer therapies [Citation9,Citation10]. Recent studies support the viewpoint that the microbiota regulates the efficacy of anticancer therapy and that the corresponding microbiota targeting may change the drug efficacy [Citation11]. Our data demonstrated this viewpoint in patients with advanced GC that ATB modulates the chemotherapy efficacy by changing the equilibrium of the gut microbiota to modify the GC outcomes.
The mechanism by which ATB-induced loss of diversity of the microbiota modulates the chemotherapy efficacy remains unclear. The chemotherapy efficacy of oxaliplatin or cisplatin dramatically decreases in germ-free mice due to reduced oxaliplatin-induced DNA damage [Citation12]. The absence of microbiota decreases reactive oxygen species production and attenuates oxaliplatin-induced DNA damage by tumor-infiltrating myeloid cells via reduced nicotinamide adenine dinucleotide phosphate oxidase 2 [Citation12]. In addition, the microbiota modulates the adaptive immune response for immunogenic cancer cell death induced by oxaliplatin [Citation13]. Furthermore, ATBs downregulate BAX and cyclin-dependent kinase inhibitor 1B, while upregulating vascular endothelial growth factor, thereby attenuating the efficacy of cisplatin in a lung cancer mouse model [Citation14]. Regarding the chemotherapeutic agent 5-fluorouracil, ATB administration-related microbiota dysbiosis with increased pathogenic bacteria. Escherichia spp., Shigella spp. and Enterobacter spp. impair its efficacy by downregulating the expression of genes involved in amino acid metabolism [Citation15]. The fact that the chemotherapeutic agent itself exacerbates the dysbiotic state renders the ATB-related dysbiosis difficult to treat.
The gut microbiota exert their effect on chemotherapy efficacy through medicating chemical signaling transduction [Citation4]. Some probiotic bacterial species may promote antitumor effects, as well as prevent the toxic side effects of chemotherapeutic drugs [Citation14]. The ATB-induced chemotherapy inefficacy of cisplatin may be rescued by concomitant supplementation of Lactobacillus bacteria [Citation14,Citation16]. These results imply that ATB-related dysbiosis may be neutralized with specific probiotic bacterial species. Longitudinal chemotherapy studies focusing on host–microbiota interactions using metagenomic, metabolomic and metatranscriptomic approaches are needed to identify probiotic bacterial species for treatment of ATB-induced dysbiosis. Further studies including clinical trials with larger sample sizes are warranted to confirm the deleterious effects of ATBs on chemotherapy. The identification of the key microbiota species capable of neutralizing unfavorable ATB-associated dysbiosis will help build future therapeutic concepts, whereby probiotic bacterial species-related modulation of the gut microbiota may increase chemotherapy efficacy.
Conclusions
ATB administration is associated with reduced chemotherapy efficacy and poorer prognosis in patients with advanced GC. Modulations in ATB-related dysbiosis and gut microbiota composition might improve the clinical outcomes of chemotherapy.
List of abbreviations
ATBs: antibiotics; GC: gastric cancer; PFS: progression-free survival; OS: overall survival; HR: hazard ratio; CI: confidence interval; ECOG: Eastern Cooperative Oncology Group; PD: disease progression.
Ethics approval and consent to participate
All procedures were supervised and approved by the Ethics Committee of the Fourth Hospital of Hebei Medical University (2020KS001). Written informed consent was obtained from the participants.
Consent for publication
Not applicable.
Availability of data and materials
Anonymized research data supporting the results of this paper are available from the corresponding author upon reasonable request.
Disclosure of interest
The authors declare that they have no competing interests.
Funding
The Natural Science Foundation of China of Hebei Province (Grant No. H2019206428) kindly provided research grants for our study.
Authors’ contributions
Z.X. collected the data of patients and wrote the paper, J.H and J.B. followed up the patients, Y.Z., L.R, Z.J. and Z.Q. performed statistical analysis, collected the data, Z.G. designed the experiments, conceived the concept and wrote the paper. All authors were involved in writing the paper and had final approval of the submitted and published versions.
Acknowledgements
Not applicable.
References
- Bray F, Ferlay J, Soerjomataram I, et al. Global cancer statistics 2018: GLOBOCAN estimates of incidence and mortality worldwide for 36 cancers in 185 countries. CA Cancer J Clin. 2018;68(6):394–424.
- Huang C, Chen R, Zheng F, et al. Inhibitory role of ATF3 in gastric cancer progression through regulating cell EMT and stemness. Cancer Cell Int. 2021;21(1):127.
- Chen Y, Zhou J, Wang L. Role and mechanism of gut microbiota in human disease. Front Cell Infect Microbiol. 2021;11:625913.
- Alexander JL, Wilson ID, Teare J, Marchesi JR, et al. Gut microbiota modulation of chemotherapy efficacy and toxicity. Nat Rev Gastroenterol Hepatol. 2017;14(6):356–365.
- Rooty B, Le Chatelier E, Derosa L, et al. Gut microbiome influences efficacy of PD-1-based immunotherapy against epithelial tumors. Science. 2018;359(6371):91–97.
- Zhang S, Chen D-C. Facing a new challenge: the adverse effects of antibiotics on gut microbiota and host immunity. Chin Med J (Engl)). 2019;132(10):1135–1138.
- Eisenhauer EA, Therasse P, Bogaerts J, et al. New response evaluation criteria in solid tumours: revised RECIST guideline (version 1.1). Eur J Cancer. 2009;45(2):228–247.
- Wu C, Lai R, Li J, et al. Antibiotics modulate chemotherapy efficacy in patients with esophageal cancer. CMAR. 2020;12:4991–4997.
- Zhao S, Gao G, Li W, et al. Antibiotics are associated with attenuated efficacy of anti-PD-1/PD-L1 therapies in Chinese patients with advanced non-small cell lung cancer. Lung Cancer. 2019;130:10–17.
- Teillant A, Gandra S, Barter D, et al. Potential burden of antibiotic resistance on surgery and cancer chemotherapy antibiotic prophylaxis in the USA: a literature review and modelling study. Lancet Infect Dis. 2015;15(12):1429–1437.
- Roy S, Trinchieri G. Microbiota: a key orchestrator of cancer therapy. Nat Rev Cancer. 2017;17(5):271–285.
- Iida N, Dzutsev A, Stewart CA, et al. Commensal bacteria control cancer response to therapy by modulating the tumor microenvironment. Science. 2013;342(6161):967–970.
- Tesniere A, Schlemmer F, Boige V, et al. Immunogenic death of colon cancer cells treated with oxaliplatin. Oncogene. 2010;29(4):482–491.
- Gui QF, Lu HF, Zhang CX, et al. Well-balanced commensal microbiota contributes to anti-cancer response in a lung cancer mouse model. Genet Mol Res. 2015;14(2):5642–5651.
- Yuan L, Zhang S, Li H, et al. The influence of gut microbiota dysbiosis to the efficacy of 5-Fluorouracil treatment on colorectal cancer. Biomed Pharmacother. 2018;108:184–193.
- Chitapanarux I, Chitapanarux T, Traisathit P, et al. Randomized controlled trial of live Lactobacillus acidophilus plus Bifidobacterium bifidum in prophylaxis of diarrhea during radiotherapy in cervical cancer patients. Radiat Oncol. 2010;5(1):31.