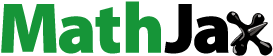
Abstract
With CRISPR/Cas9 gene editing technology, human umbilical cord mesenchymal stem cells (HUMSCs) were directionally induced to differentiate into parathyroid cells. The study provides the theoretical and experimental basis for the application of HUMSCs in the clinical treatment of parathyroid hypofunction. In this study the CRISPR/Cas9 gene editing technology was used to introduce the PTH gene into HUMSCs to induce the differentiation of HUMSCs. After 28 days of induction culture, HUMSCs edited by CRISPR/Cas9 showed morphological changes. PTH expression was detected by quantitative real-time PCR (qRT-PCR), western blotting and immunohistochemistry, the expression level of PTH in parathyroid adenoid cells obtained after CRISPR/Cas9 gene editing was significantly increased. The expression levels of CASR, GCM2 and PTH were 98.9%, 93.6% and 97%, respectively. This experiment verified that editing the PTH gene with the CRISPR/Cas9 system could directionally induce human umbilical cord mesenchymal stem cells to differentiate into parathyroid cells.
Introduction
Due to the advances in the epidemiology of complications and clinical studies [Citation1, Citation2], the understanding of chronic hypoparathyroidism (HP) has been gradually improved and it is believed that anterior cervical surgery is the most common cause for HP and accounts for about 75% of HP cases[Citation3]. Even though the majority of the parathyroid is reserved in situ, permanent HP is often observed [Citation4]. It is estimated that 25.4 ∼ 83% of neck surgery patients are subjected to 6-month common HP [Citation5]. Permanent post-operative HP (defined as more than 6 months) occurs in approximately 0.12%-14.3% of cases [Citation6–8]. The complications of HP include chronic kidney diseases, kidney stones, renal calcification, epilepsy, subcapsular cataracts, intracerebral calcification, severe hypocalcaemia and even laryngospasm or epileptiform grand mal episodes in case of rapid decrease in blood calcium levels.
Parathyroid autologous transplantation can prevent permanent HP [Citation9]. However, the effect of parathyroid autologous transplantation after the thyroid resection remains controversial [Citation10, Citation11]. In order to solve the controversy, in 2019, Wang et al. [Citation12] reviewed 25 independent studies involving 10,531 participants, explored the relationship between parathyroid autologous transplantation and the risk of HP, and indicated that parathyroid autologous transplantation was associated with the increased risk of HP.
When cryopreserved parathyroid tissues cannot be transplanted, an allogeneic transplantation of cultured parathyroid cells without immunosuppression should be considered as an alternative to calcium and vitamin D3 therapy. From 1990 to 2016, in 316 cases of parathyroid allogeneic transplantation and redo allogeneic transplantation in Warsaw, Poland, 55.1% of the patients showed the improved serum parathyroid hormone (PTH) level and normal blood calcium level observed under the oral administration condition of calcium and vitamin D3 substitutes, and the ratios of the cases with the functional recovery in 1 ∼ 6 months, 6 ∼ 12 months, 12 ∼ 24 months, and 24 ∼ 127 months after transplantation were respectively 34.5%, 10.2%, 6.2% and 1.9% [Citation13], displaying a declining trend. However, considering the side effects of life-long immunosuppression, HP patients are unwilling to accept this treatment outcome.
The standard treatment for HP is calcium supplements and active vitamins, which can lead to hypercalciuria, hypercalcemia or hypocalcaemia. Parathyroid hormone replacement therapy has been used as an auxiliary treatment to adult patients with chronic low parathyroid hormone, but it cannot adequately control these patients. To date, the data from randomized clinical trials (RCTS) only demonstrated that parathyroid hormone therapy reduced the serum phosphorus levels and the dose of calcium supplements and active vitamin D required to maintain normal serum calcium [Citation14, Citation15]. In randomized controlled trials, improved quality of life or documented reduction of urinary calcium was not obtained. Currently, only a once-daily injection regimen is available. It causes a sharp increase in the plasma parathyroid hormone levels. However, due to the short plasma half-life, parathyroid hormone is only circulated 8 to 12 h after injection and the normal physiological plasma distribution is not achieved.
Due to the low immunogenicity, unlimited proliferation and multi-directional differentiation potentials, stem cells enable the repair and regeneration of various tissues and organs [Citation16–18], but the traditional induction method of stem cells has some disadvantages: such as the less harvested target cells and low purity. In addition, the long-term survival of transplanted stem cells and stable PTH secretion for maintaining constant physiological blood calcium level remain to be confirmed. Therefore, in order to support the development of new regenerative therapies for patients with parathyroid-related diseases, we sought to develop a protocol to guide the differentiation of pluripotent stem cells into parathyroid cells. Due to the development in precise targeting therapy in recent years, cell therapy combined with genetic modifications and gene editing (for example: the CRISPR/Cas9 technology) has been proposed as a new treatment mode with significant curative effects. Through in vitro culture technology and genetic modification technology, stem cell transplantation is expected to be applied in tissue repair since it can avoid the disadvantage of traditional treatment and improve the therapeutic effect and the quality of life of patients [Citation19, Citation20].
Materials and methods
Culture and identification of HUMSCs
Primary HUMSCs huxub-01001 (provided by Guangzhou Cyagen Biosciences Co., Ltd.) were maintained in human umbilical cord blood mesenchymal stem cell medium (huxub-900111, a complete medium) in an incubator (37 °C and 5% CO2). HUMSCs were collected and analyzed by flow cytometry. The expression levels of CD29 (FITC Conjugated Anti-human CD29, Integrin beta 1, eBioscience), CD44 (FITC Conjugated Anti-mouse/human CD44, Pgp-1, Ly-24, eBioscience), CD73 (Anti-human CD73 FITC, eBioscience), CD105 (Anti-Human CD105 PE, eBioscience), CD166 (Anti-human CD166 PerCP-eFluor®710, eBioscience), CD34 (Human CD34 FITC, BD Biosciences), and CD45 (CD45 Monoclonal Antibody-APC, ImmunoWay) in HUMSCs were respectively determined.
CRISPR gene editing induced the differentiation of HUMSCs
HUMSCs were inoculated in 6-well culture plates. When the cells grew to a fusion rate of 40-80%, PTH Lentiviral Activation Particles (h) (Santa Cruz sc-401280-LAC) mixed with 5 μg/mL polybrene were added. After centrifugation at 25 °C and 800 g for 30 min, the samples were placed at 37 °C and cultured in an incubator (5% CO2). After 24 h, the fresh complete medium was replaced. In order to screen out the cell lines expressing PTH stably, puromycin was used for clonal screening and cell morphology was observed under an inverted microscope(OLYMPUS CKX41, U-CTR30-2).
Morphological observation
HUMSCs were induced and cultured for 28 days. During the whole culture period, cell morphology and growth state were observed dynamically.
PTH expression detection by qRT-PCR
After cell collection, total RNA was extracted with Trizol reagent, and cDNA was synthesized by reverse transcription with Oligo(dT) as the primers and purified RNA as the template. Primers were designed as follows: PTH-F:TCAGCATCAGCTACTAACATACC; PTH-R: TCTCTTCTTAACAGATTTCCCATCC; β-actin-F: TGGATCAGCAAGCAGGAGTA; β-actin -R: TCGGCCACATTGTGAACTTT. The fluorescence quantitative PCR was performed with the SYBR Green qPCR SuperMix Kit (Invitrogen Company). PTH mRNA levels were normalized to β-actin levels and expressed as relative levels.
PTH expression detection by western blotting
Parathyroid adenoid cells were carefully removed from the culture medium and then washed with PBS 3 times. Then 100 μL M-PER Reagent were added to each plate well, followed by centrifugation at 500 g for 10 min. Then the cell lysis supernatant was collected and transferred to a new tube. BCA kit was used to determine the protein concentration in the lysis supernatant and the protein concentration in each group was adjusted to the same concentration. Sodium dodecyl sulfate polyacrylamide gel electrophoresis (SDS-PAGE) was performed and proteins were transferred onto a PVDF membrane (Millipore) by using Tris-glycine transfer buffer(Sangon Biotech). After transfer, the membrane was blocked with 5% non-fat dry milk and then incubated with anti-PTH antibody (abcam, ab154792) and anti-β-actin antibody (abcam, ab8226) at room temperature for 1 h. The membrane was developed using an EasyBlot ECL kit (Sangon Biotech) with X-ray film in dark. The relative greyscale values of the PTH protein bands were measured using Image-J software and normalized to β-actin.
Immunohistochemical test of PTH
The cells were fixed with 4% paraformaldehyde and permeated at room temperature with 0.5%Triton x-100. Then the cells were washed with PBS and incubated with 3% H2O2 for 15 min. After H2O2 was removed, the cells were washed with PBS, blocked with the blocking solution for 30 min, incubated with the primary (anti-parathyroid Hormone (ab40630)) and secondary (goat anti-rabbit IgG H&L (HRP) (ab205718)) antibodies. After the cells were washed with PBS, the substrate diaminobenzidine (DAB) was added for colour development. The cells were washed with PBS 3 times, and then stained with Harris haematoxylin. Finally, the cells were observed under a microscope.
Detection of CASR, PTH and GCM2 by flow cytometry
Parathyroid adenoid cells were collected. After the cells were immobilized and transfused, anti-CASR antibody (abcam, ab19347), anti-parathyroid antibody (abcam, ab215856) and anti-GCM2 antibody (bioss, bs-13314r) were added and incubated at 4 °C for 1 h. The cells were washed with PBS and secondary anti-rabbit lgG-FITC antibody (sigma, 018K60572) was added. The isotype control was set and incubated at 4 °C for 30 min. The cells were then washed for flow cytometry analysis according to the manufacturer’s protocol (FACSCanto II, BD, USA, https://www.bdbiosciences.com/zh-cn/instruments/clinical-instruments/clinical-cell-analyzers/facscanto-ii).
Statistical analysis
Results were expressed as mean values with standard deviation (± s) and analyzed by Statistical Package for Social Science (SPSS) 16.0 software (SPSS Inc., Chicago, IL, USA). Student’s t test was used for statistical analysis. Differences were considered statistically significant at the p < 0.05 level.
Results
Identification of HUMSCs
The flow cytometry results showed that the positive percentages of the indicators of CD29, CD44, CD73 and CD105 of the HUMSCs were higher than 95%. The positive percentage of CD166 was higher than 60%, and the negative percentages of CD34 and CD45 were lower than 5% (). The test results were consistent with the expression characteristics of surface markers of MSCs.
Expression of PTH detected by qRT-PCR and western blotting
After CRISPR/Cas9 gene editing, the expression levels of PTH were detected by qRT-PCR and western blotting. The results showed that the expression levels of PTH mRNA and PTH protein in parathyroid adenoid cells in the gene editing group were significantly higher than HUMSCs ().
Expression of PTH detected by immunohistochemical test
The results of the immunohistochemical test indicated that HUMSCs showed PTH expression after the induction. After induction and culture, parathyroid adenoid cells edited by CRISPR/Cas9 gene editing technology showed active growth, deep staining and high expression level of PTH ().
Detection of cell surface markers in parathyroid adenoid cells
Parathyroid adenoid cells induced from human umbilical cord blood mesenchymal stem cells edited with CRISPR could overexpress PTH. The expression levels of CASR, GCM2 and PTH were detected by flow cytometry. The results indicated that the expression levels of CASR, GCM2 and PTH were respectively 98.9%, 93.6% and 97%, which were consistent with the characteristics of parathyroid cells ().
Discussion
Any errors in synthesis, release and binding of parathyroid hormone in target cells may cause parathyroid hypofunction, accompanied by increased neuromuscular excitability, muscle spasm, convulsion and even serious life risk. Hypoparathyroidism is a rare disease and the epidemiological data of hypoparathyroidism have not been reported in many countries. However, in recent years, the number of patients with hypoparathyroidism after clinical surgery has increased gradually and it has become one of the main clinical problems in thyroid, parathyroidism, head and neck surgeries [Citation21]. Neither the traditional treatment regimen, nor PTH alternative therapy can restore the physiological calcium and phosphorus levels, and the high price of PTH alternative therapy also limits its application. Therefore, it is still urgent to explore a new treatment method. The potential of human pluripotent stem cells for tissue repair and regeneration has been demonstrated in many animal disease models, but their application in the treatment of hypoparathyroidism is seldom reported [Citation22]. Traditional induction methods can induce mesenchymal stem cells to differentiate into parathyroid adenoid cells, but our results suggested that the purity and vigour of the cells harvested by traditional induction methods could not meet the clinical requirements. CRISPR/Cas9 gene editing directed PTH gene into umbilical cord mesenchymal stem cells, which maintained the pluripotency and differentiation ability of mesenchymal stem cells. Parathyroid cells expressing high levels of PTH, CASR and GCM2 were harvested through specific induction differentiation, which showed the higher targeting efficiency and accuracy and avoided the shortcomings of traditional induction methods.
The CRISPR/Cas9 gene editing system which can selectively modify specific genes is a powerful editing tool for studying gene function, gene therapy, specific gene mutations, disease eradication, and the modifications of cells and organisms. Mesenchymal stem cells have the potential to differentiate into particular downstream cells [Citation22–25]. The studies on stem cell differentiation confirmed the reliable function of the cells. Stem cell transplantation may cure diseases caused by specific types of cell damage, but traditional induction methods have low differentiation efficiency and often fail finally. The CRISPR/Cas9 system can realize highly efficient and reversible genome editing, edit stem cell genome at different scales, and specifically induce stem cells to differentiate into cells with stable functions [Citation26–28].
In this experiment, the CRISPR/Cas9 gene editing system was used to introduce the PTH gene into HUMSCs to induce their differentiation. The obtained parathyroid adenoid cells could highly express PTH, CASR and GCM2 and the expression levels of CASR, GCM2 and PTH were respectively 98.9%, 93.6% and 97%. We believe that the differentiated parathyroid adenoid cells may have the ability to cure parathyroid injuries. The parathyroid gland is the only organ that synthesizes and secretes PTH. PTH, as a specific peptide hormone secreted by parathyroid chief cells, is one of the surface markers of parathyroid gland cells and can maintain the normal calcium and phosphorus levels in the body. In mammalian cells, GCM2 is one of the transcription factors that determine the differentiation of neural progenitor cells and its high expression is mainly confined to the parathyroid gland. Both the thyroid and the thymus originate from the "thymus/parathyroid primordium" of the third pharyngeal sac, in which the primordium expressing GCM2 develops into the parathyroid gland [Citation29]. After the GCM2 gene was knocked out in mice, the parathyroid gland would be missing in mice, indicating that GCM2 plays an important role in the development of parathyroid gland cells in the embryonic stage [Citation30]. Yamada et al. [Citation31] found that parathyroid gland cells play an important role in cell proliferation and maintenance in mature individuals. GCM2 directly regulates the expression of calcium ion sensitive receptors and PTH, so GCM2 is also one of the specific markers of parathyroid gland cells. Calcium-sensing receptors (CASR) belong to the G-protein-coupled receptor family and can participate in the synthesis and secretion of PTH, promote the reabsorption of calcium by the kidney, and maintain the electrolyte balance in the body. CASR exists on the surface of parathyroid cells and can convert extracellular calcium ion concentration into signals to regulate the physiological functions of parathyroid cells [Citation32]. When CASR is absent, there will be parathyroid hyperplasia and hypertrophy. Therefore, CASR can be considered as another specific marker of CASR parathyroid cells [Citation33].
Conclusions
In this study, with CRISPR/Cas9 gene editing technology, high purity umbilical cord mesenchymal stem cells differentiated into parathyroid cells with highly and stably expressed PTH, CASR and GCM2. The study made a new breakthrough for the directional differentiation of mesenchymal stem cells into parathyroid gland cells and may also bring new hope to hypoparathyroidism patients. However, a series of problems still need to be solved in order to translate the study results into clinical achievements. The mechanism of parathyroid cell differentiation, the differentiation potential of HUMSCs into parathyroid cells with normal physiological functions, the long-term survival of transplanted cells and the PTH secretion activity need to be further demonstrated by disease models.
Author contributions
(I) Conception and design: H Zhang; (II) Administrative support: W Zhu; (III) Provision of study materials: C Qiu; (IV) Collection and assembly of data: H Zhang; (V) Data analysis and interpretation: H Zhang; (VI) Manuscript writing: All authors; (VII) Final approval of manuscript: All authors.
Disclosure statement
All authors declare they have no other competing interests.
Data availability
All data that support the findings reported in this study are available from the corresponding author upon reasonable request.
Additional information
Funding
References
- Astor MC, Løvås K, Debowska A, et al. Epidemiology and health-related quality of life in hypoparathyroidism in Norway. J Clin Endocrinol Metab. 2016; 101(8):3045–3053.
- Brandi ML, Bilezikian JP, Shoback D, et al. Management of hypoparathyroidism: summary statement and guidelines. J Clin Endocrinol Metab. 2016; 101(6):2273–2283.
- Bilezikian JP, Khan A, Potts JT, et al. Hypoparathyroidism in the adult: epidemiology, diagnosis, pathophysiology, target-organ involvement, treatment, and challenges for future research. J Bone Miner Res. 2011; 26(10):2317–2337.
- Lorente-Poch L, Sancho JJ, Ruiz S, et al. . Importance of in situ preservation of parathyroid glands during total thyroidectomy. Br J Surg. 2015; 102(4):359–367.
- Page C, Strunski V. Parathyroid risk in total thyroidectomy for bilateral, benign, multinodular goitre: report of 351 surgical cases. J Laryngol Otol. 2007; 121(3):237–241.
- Asari R, Passler C, Kaczirek K, et al. Hypoparathyroidism after total thyroidectomy: a prospective study. Arch Surg. 2008; 143(2):132–137; discussion 138.
- White ML, Gauger PG, Doherty GM. Central lymph node dissection in differentiated thyroid cancer. World J Surg. 2007; 31(5):895–904.
- Ahmed N, Aurangzeb M, Muslim M, et al. Routine parathyroid autotransplantation during total thyroidectomy: a procedure with predictable outcome. J Pak Med Assoc. 2013; 63(2):190–193.
- El-Sharaky MI, Kahalil MR, Sharaky O, et al. Assessment of parathyroid autotransplantation for preservation of parathyroid function after total thyroidectomy. Head Neck. 2003; 25(10):799–807.
- Sitges-Serra A, Lorente-Poch L, Sancho J. Parathyroid autotransplantation in thyroid surgery. Langenbecks Arch Surg. 2018; 403(3):309–315.
- Su A, Gong Y, Wu W, et al. Effect of autotransplantation of a parathyroid gland on hypoparathyroidism after total thyroidectomy. Endocr Connect. 2018; 7(2):286–294.
- Wang B, Zhu C-R, Liu H, et al. The effectiveness of parathyroid gland autotransplantation in preserving parathyroid function during thyroid surgery for thyroid neoplasms: a Meta-analysis. PLoS One. 2019; 14(8):e0221173.
- Barczyński M, Gołkowski F, Nawrot I. Parathyroid transplantation in thyroid surgery. Gland Surg. 2017; 6(5):530–536.
- Akkan T, Dagdeviren M, Koca AO, et al. Alternate-day calcium dosing may be an effective treatment option for chronic hypoparathyroidism. J Endocrinol Invest. 2020; 43(6):853–858.
- Zavatta G, Clarke BL. Challenges in the management of chronic hypoparathyroidism. Endocr Connect. 2020;:9(10R229–40.
- Lou G, Chen Z, Zheng M, et al. Mesenchymal stem cell-derived exosomes as a new therapeutic strategy for liver diseases. Exp Mol Med. 2017; 49(6):e346.
- Vizoso FJ, Eiro N, Cid S, et al. Mesenchymal stem cell secretome: toward Cell-Free therapeutic strategies in regenerative medicine. Int J Mol Sci. 2017; 18(9):1852
- Marcucci G, Masi L, Cianferotti L, et al. Chronic hypoparathyroidism and treatment with teriparatide. Endocrine. 2021; 72(1):249–259.
- Yingjun X, Yuhuan X, Yuchang C, et al. CRISPR/Cas9 gene correction of HbH-CS thalassemia-induced pluripotent stem cells. Ann Hematol. 2019; 98(12):2661–2671.
- Luther DC, Lee YW, Nagaraj H, et al. Delivery approaches for CRISPR/Cas9 therapeutics in vivo: advances and challenges. Expert Opin Drug Deliv. 2018; 15(9):905–913.
- Edafe O, Balasubramanian SP. Incidence, prevalence and risk factors for post-surgical hypocalcaemia and hypoparathyroidism. Gland Surg. 2017; 6(Suppl 1):S59–s68.
- Fukushima H, Yoshioka M, Kawatou M, et al. Specific induction and long-term maintenance of high purity ventricular cardiomyocytes from human induced pluripotent stem cells. PLoS One. 2020; 15(11):e0241287.
- Hu W, Zi Z, Jin Y, et al. CRISPR/Cas9-mediated PD-1 disruption enhances human mesothelin-targeted CAR T cell effector functions. Cancer Immunol Immunother. 2019; 68(3):365–377.
- Jafari N, Kim H, Park R, et al. CRISPR-Cas9 mediated NOX4 knockout inhibits cell proliferation and invasion in HeLa cells. PLoS One. 2017; 12(1):e0170327.
- Meca-Cortés O, Guerra-Rebollo M, Garrido C, et al. CRISPR/Cas9-Mediated knockin application in cell therapy: a non-viral procedure for bystander treatment of glioma in mice. Mol Ther Nucleic Acids. 2017; 8:395–403.
- Lyu C, Shen J, Wang R, et al. Targeted genome engineering in human induced pluripotent stem cells from patients with hemophilia B using the CRISPR-Cas9 system. Stem Cell Res Ther. 2018; 9(1):92.
- Qu SW, Xue YN, Li P, et al. Advances in the research of stem cell tissue-engineering. Zhonghua Shao Shang Za Zhi. 2020;36(6):510–515.
- Hsu M-N, Chang Y-H, Truong VA, et al. CRISPR technologies for stem cell engineering and regenerative medicine. Biotechnol Adv. 2019; 37(8):107447.
- Gordon J, Bennett AR, Blackburn CC, et al. Gcm2 and Foxn1 mark early parathyroid- and thymus-specific domains in the developing third pharyngeal pouch. Mech Dev. 2001; 103(1-2):141–143.
- Günther T, Chen ZF, Kim J, et al. Genetic ablation of parathyroid glands reveals another source of parathyroid hormone. Nature. 2000; 406(6792):199–203.
- Yamada T, Tatsumi N, Anraku A, et al. Gcm2 regulates the maintenance of parathyroid cells in adult mice. PLoS One. 2019; 14(1):e0210662.
- Fan Y, Liu W, Bi R, et al. Interrelated role of Klotho and calcium-sensing receptor in parathyroid hormone synthesis and parathyroid hyperplasia. Proc Natl Acad Sci U S A. 2018; 115(16):E3749–e3758.
- Ritter CS, Finch JL, Slatopolsky EA, et al. Parathyroid hyperplasia in uremic rats precedes down-regulation of the calcium receptor. Kidney Int. 2001;60(5):1737–1744.