Abstract
Ochradenus arabicus is a significant therapeutic plant which grows in desert zones of Saudi Arabia. It has antimicrobial, anticancer, cancer prevention, antidiabetic, allopathic and pabulum properties. The present study evaluated the biosynthesis of silver nanoparticles (AgNPs) utilizing the leaf concentrate of plant O. arabicus having novel ethnomedicinal potential. The incorporated AgNPs were visualized utilizing UV-Visible spectroscopy, X-ray diffraction (XRD), Fourier transform infrared spectroscopy (FTIR) and transmission electron microscopy (TEM). The antimicrobial impact of AgNPs delivering O. arabicus was considered utilizing distinctive pathogenic microorganisms, for example, Escherichia coli, Staphylococcus aureus, Pseudomonas aeruginosa and Streptococcus mutans. From the plate dispersion results, the incorporated silver nanoparticles demonstrated potent antibacterial properties and high antimicrobial action contrasted with the ionic silver.
Supplemental data for this article is available online at https://doi.org/10.1080/13102818.2021.1961609 .
Introduction
Nanotechnology is a quickly developing interdisciplinary field with the sole point of assembling new materials at nanoscale [Citation1]. Metal nanoparticles are of clinical interest because of their reactant, optical, electrical and antimicrobial properties [Citation2]. Silver nanoparticles (AgNPs) have antimicrobial properties (against different pathogens) and cell reinforcement activity [Citation3,Citation4]. Silver is highly toxic at elevated concentration; however a few studies suggest that silver nitrate (AgNO3) seems to perform better in terms of catalytic action, chemical stability, biocompatibility, etc. [Citation5]. The literature on AgNPs is flooded with reports on its antimicrobial, catalytic and anticancer properties [Citation6,Citation7]. AgNPs have an advantage over their metal precursors and salts owing to slow and regulated release of silver [Citation8].
AgNPs are generally utilized in a wide range of applications and exclusively properties of plasmonics [Citation9]. Some reports associate AgNPs with harmful synthetics, high energy requirement, tedious cleaning and little material change [Citation10]. As of now, the validity of biosynthetic nanoparticle synthesis depends on the development of perfect, nontoxic and effective incorporation of strategies combined with safety, negligible wastage of energy and crude materials. Additionally, this type of synthesis of AgNPs is also cost effective, environmentally friendly, does not need much higher temperature or toxic materials and can easily be performed at a larger scale [Citation11]
Ochradenus is a small genus of about eight species growing in the desert and drylands of Southwest Asia and Northeast Africa, with a centre of diversity in the South Arabian Peninsula [Citation12]. The taxonomy of the genus is very intricate, while only one species (O. baccatus Delile) is widely distributed. Different species are limited as endemic in the Arabian Peninsula, particularly in Oman and the Horn of Africa [Citation13]. Ochradenus arabicus Chaudhary Hillc. and A. G. Mill. (Resedaceae) generally grows in sandy, desert and dry areas of Saudi Arabia, the United Arab Emirates, Oman and Yemen [Citation14]. O. arabicus has a multitude of medicinal properties, because of which it is widely used locally for treatment of different ailments in many countries including Saudi Arabia [Citation14–16]. O. baccatus possesses antitumor activity by exhibiting cytotoxicity (ca. 97%) against cultured melanoma cell lines [Citation16]. Plant fractions additionally showed antimalarial properties (protozoa) and growth inhibitory activity to wheat rootlets [Citation16]. The genus Ochradenus has not been largely explored for its metabolomic potential; however, a few natural products, including quercetin 3-gentiobioside, quercetin glycosides, 7-O-a-l-rhamnopyranosyl quercetin 3-O-b-d-glucopyrannosyl-(1!2)-a-l-rhamnopyrannoside, 7-O-al-rhamnopyranosylquercetin 3-O-(6-O-p-coumaroyl-b-d-glucopyrannosyl)-(1!6)-bd-glucopyrannoside, isoquercitrin, kaempferol glycosides, afzelin and astragalin, have been isolated [Citation17]. Limited information is available about the possible biological effects of O. arabicus. Its leaves are deciduous, which suggests morphological adaptations to the water-scarce environment. Its flower is polygamous, and simplification is visually perceived in the structure.
O. baccatus Del. is generally spread, while most other species from this genus are confined as endemic in the Arabian Peninsula, particularly in Oman and the Horn of Africa [Citation13]. O. arabicus has been investigated for its effective spread and protection through tissue culture strategies [Citation14, Citation18]. Some molecular markers have already been studied for its identification. There are numerous reports of bioactive ingredients isolation and description of the genus. There is insufficient information about the probable biological actions of O. arabicus. Since the plant is medicinally significant for local people, in the present study we carried out screening experiments to understand its potential role in the phytosynthesis of AgNPs with antimicrobial potential.
Materials and methods
Selection of plant material
Ochradenus arabicus plants bearing seeds were gathered from the focal locale of Saudi Arabia. The seeds were then surface sanitized with sodium hypochlorite containing 4% accessible chlorine for 10 min and flushed well with double distilled water (D.D.). The surface-sterilized seeds were then cultured in glass Petri dishes (25 seeds per plate). At the point when the plants developed to the two-cotyledonary stage, they were used for in vitro shoot multiplication. The Murashige and Skoog (MS) media [Citation19] enhanced with 2 percent sucrose and benzyl adenine (BA) was used. Shoots multiplication was achieved when nodal explants were transferred onto MS media supplemented with 1.0 μmol L−1 BA. The pH of the medium was maintained at 5.8 up to autoclaving for 15 min at 121 °C and 104 kPa. All samples were placed in a culture room at 55–60% RH (relative humidity) and 25–28 °C under a 16 h photoperiod of 40 μmol m−2s−1 PPFD by cool white fluorescent tube light.
Plant extract preparation
Ochradenus arabicus plant material (10.0 g) was taken from in vitro shoots which had been maintained in vitro in the previous study. The selected plant was cut into small pieces and 50 mL of distilled water was poured over it. The plant material was boiled in 50 mL water and allowed to cool at room temperature. The cooled solution was then filtered through Whatman No 1 filter paper.
Biosynthesis of AgNPs
The constituents of the preparation of AgNPs included silver nitrate, sodium dodecyl sulphate and Ochradenus arabicus shoot extract. To prepare the AgNPs, 1.5 mL of 1.0 mmol L−1 AgNO3 was added to three different volumes of the extract, viz 2.5 mL, 5 mL and 10 mL were added followed by 1 mL of 0.1% sodium dodecyl sulphate (SDS) solution and was subjected to heating in boiling water for 5 min and finally the volume was made up to 25 mL. Upon heating, the yellow coloured extracted solution changed to dark brown colour, indicating the synthesis of silver nanoparticles.
Characterization of silver nanoparticles
The synthesized AgNPs were characterized using spectrophotometry, Fourier transform infrared spectroscopy (FTIR, Thermo Scientific NICOLET 6700 FT-IR Spectrometer FT-IR) and X-Ray diffraction (X-ray Diffractometer (XRD) Rigaku Ultima IV, Germany). To determine the point of maximum production of AgNPs, the synthesized nanoparticle mixture was subjected to UV-vis spectrophotometric analysis where the absorption spectrum of the AgNPs was taken in the range of 300–550 nm with deionized water as a blank sample, while the phase and purity was checked using the spectrophotometer (Evolution 300, Thermo UV-Visible spectrophotometer with matched quartz cuvette).
Preparation of microorganisms
The organisms Escherichia coli (ATCC25922), Staphylococcus aureus (ATCC 25923), Pseudomonas aeruginosa (ATCC27853) and Streptococcus mutans (ATCC25175) were selected in the present investigation. The test organisms were inoculated individually into tubes containing 5 mL of nutrient broth (NB) and grown overnight. Adjustments of the suspension were made in such a way that they were equivalent to 0.5 Mcfarland scale (1.5 × 108 cfu). Freshly prepared bacterial suspensions were swabbed on the Mueller Hinton Agar (MHA).
Antimicrobial activity
Two tests were done to evaluate the antibacterial action of AgNPs. The primary test comprised of an agar well diffusion assay where the zone of inhibition decides the degree of antibacterial effect for all three solutions. The subsequent test was to decide the base inhibitory fixation (MIC) of the AgNPs made.
Suspensions of the test microorganisms were streaked on the medium. Wells were then cut on the plates. Various centralizations of the AgNPs (100 and 150 μg mL−1) were added to the wells. All the plates were placed in an incubator at 37 °C for 24 h to determine the inhibitory potential of the AgNPs on chosen microbes. Doxycycline (30 μg mL−1) was used as positive control and sterile nutrient broth was used as negative control. The methodology was repeated threefold and the mean values were noted for information.
The minimum inhibitory concentration (MIC) and minimum bactericidal concentration (MBC) of AgNPs was determined as the lowest concentration that restrains the noticeable development of the test microbes. We used the microbroth dilution approach [Citation20]: 100 μL of the test culture were added to the wells of 96 well microtiter plates and AgNPs concentrations (100 and 150 μg mL−1) were added to each well. The plates were incubated overnight and observed for development of colonies.
Results and discussion
The present study reports an eco-friendly synthesis of silver nanoparticles, where water extract of O. arabicus was utilized for the reduction of Ag2+. The experiment proceeds with the addition of plant extracts to the aqueous solution of silver nitrate subsequently resulting in the reduction of Ag2+ ions into Ag. The course of the reaction proceeds with the change in the colour of the solution from light yellow to brown. This change in colour can be attributed to formation of silver nanoparticles and subsequently the surface plasmon absorption of the synthesized silver nanoparticles. The nanoparticle suspension was checked spectrophotometrically.
The synthesized nanoparticles were monitored spectrophotometrically. The spectral studies of the synthesized nanoparticles and the blank consisting of silver nitrate and sodium dodesylsulphate (SDS) showed that the blank solution absorbed maximally at 301 nm while with the addition of the plant extract to the blank solution a shift in the λ max and a new peak appeared at 429 nm. This change in shift can be attributed to the formation of silver nanoparticles (). Further, the effect of the change in the volume of the plant extract was tested and it was found that with the increase in the plant extract volume from 2.5 mL to 10 mL there was an increase in the absorbance with respect to the extract volume (). The increase in the absorbance with increase in the extract volume, indicates an increased number of AgNPs. The possible reason for this can possibly be credited to increased number of functional groups reacting with the silver salt; this increased number of functional groups can possibly be directly associated with the higher volume of plant extract. Thus it can be concluded that the increase in the formation of AgNPs was directly related to an increase in the absorbance [Citation21,Citation22]. The temperature also plays an important role in the formation of AgNPs, since the experiment in this study was performed in boiling water. It has been reported that at this temperature, rapid nucleation can be induced in a shorter period of time [Citation23]. This also helps in the synthesis of small sized AgNPs that have too narrow size distribution; this was further confirmed by the TEM image which reveals a size distribution range of 6–27 nm in this case [Citation24].
Figure 1. Absorbance of nano solution with AgNO3 solution. Silver nitrate solution (A); Silver Nano solution (B).
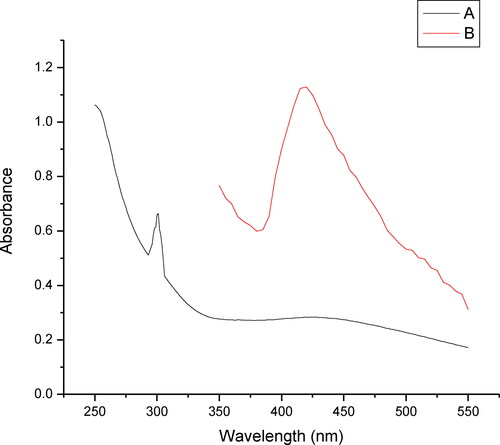
Figure 2. Absorbance of nano solution at different concentrations: plant extract volume of 10 mL (A), 5.0 mL (B) and 2.5 mL (C).
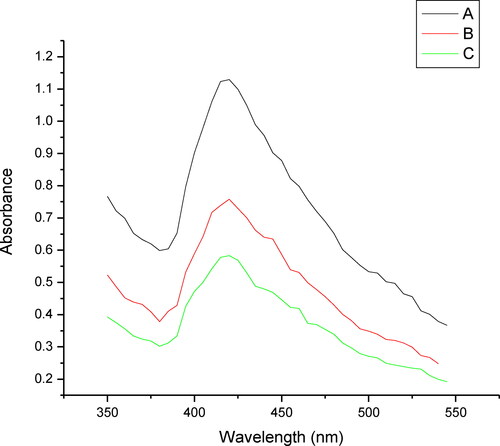
XRD patterns of AgNPs produced from plant extracts were assigned to a face cantered cubic geometry [Citation25]. The allowed Bragg reflections revealed discrete peaks at 2θ values, which can be attributed to all odd (111, and 311) or all even (200 and 220) crystalline planes of AgNPs (). Other peaks in the diffractogram can be attributed to the residues of the organic components of the plant extract and confirm the presence of plant metabolite moieties on AgNPs surface [Citation26]. This suggested the involvement of plant extract components in AgNPs formation. The interaction between various organic components (carboxylate groups) with silver ions causes the bioreduction of silver nitrate, which leads to the formation of AgNPs. All functional groups including –COO−., OH and CO present in the plant materials have greater possibility to attach to Ag+ and are likely responsible for the reduction and as well as stabilization of synthesized NPs [Citation23]. The crystalline size of the nanoparticles was calculated using Scherrar’s equation and was found to be 29 nm.
The FTIR spectrum of biosynthesized AgNPs () showed the presence of metabolites responsible for the reduction of Ag particles. The presence of a typical absorption peak at 3435.8 reveals the occurrence of OH group of phenols and alcohols [Citation27]. The absorption band of the biosynthesized solution in the region of 2359.3 cm−1 was due to the presence of symmetric stretching of COO−. The bands at 2078.9 indicate the presence of alkynes, N–C and N = C groups, while the absorption bands at 1634.7 cm−1 is corresponded to the C = C band in aromatic and amide compounds of proteins [Citation28,Citation29]. The bands at 707.6 cm−1 correspond to the presence of = CH in aromatic compounds. Free COO − groups presented in proteins easily bind to the metal nanoparticles and make them stable [Citation26]. Hence, it was concluded that the OH and CO groups of plant extract compounds have shown a vital role in reducing and stabilization of AgNPs [Citation30].
The morphology and particle size of AgNPs synthesized with the leaf extract were characterized using TEM (). The 100 nm resolution TEM studies of the synthesized nanoparticles showed 6–27 nm size particles with predominantly spherical shape and uniform size distribution (). Some nanoparticles were agglomerated and were not considered for size measurements. The TEM images show the actual size, which was found to be smaller than the crystallite size calculated from the XRD spectra.
Figure 5. TEM micrograph of silver nano particles syntesized by O. arabicus extract. Scale bar = 100 nm.
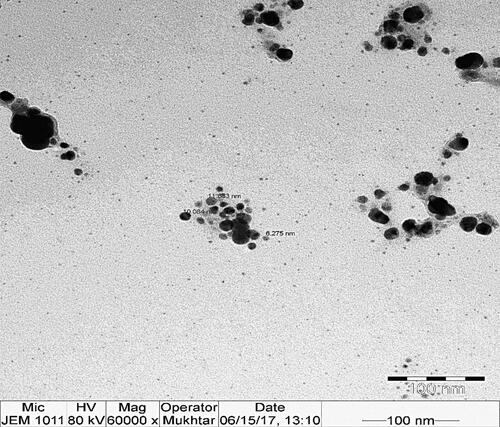
Antibacterial activity of AgNPs
The antimicrobial action of AgNPs was explored against E. coli, S. aureus, P. aeruginosa and S. mutans utilizing the agar well diffusion test. The zones of inhibition (mm) around each well containing AgNPs and AgNO3 solution are shown in . Among the tested microorganisms, P. aeruginosa was generally restraint with an inhibition zone of 12 and 14 mm at 100 and 150 μg/well concentration of AgNPs, respectively. E. coli was generally susceptible, as it displayed the largest zones of inhibition: 14 and 17 mm at 100 and 150 μg/well concentration of AgNPs, respectively. Antibiotic doxycycline was used as a positive control in the present study and demonstrated inhibition zones of 21, 18, 20 and 17 mm against E. coli, S. aureus, P. aeruginosa and S. mutans, respectively. In the present study, the zone of inhibition of AgNPs (150 μg/well) was found comparable with that of Doxycycline (30 μg/well).
Figure 6. Antimicrobial activity of silver nanoparticles by agar well diffusion method. Antimicrobial activity against E. coli (A), S. aureus (B), P. aeruginosa (C) and S. mutans (D). Well (i) is control, i.e. distilled water, well (ii) 100 µg silver NP and well (iii) 150 µg Ag-NP.
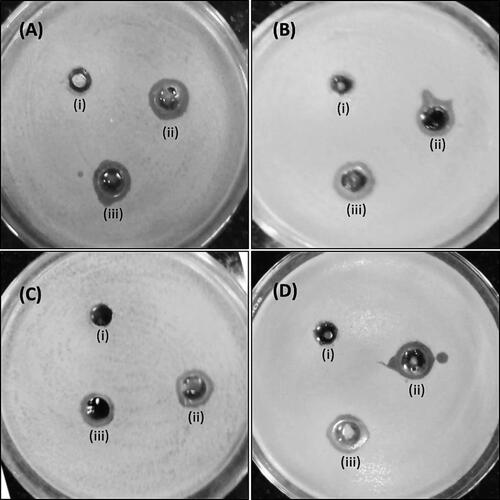
Our findings are in agreement with the observations made with AgNPs synthesized from in vitro grown shoots of Tamarix nilotica [Citation20]. The discoveries indeed show stronger antimicrobial action of AgNPs when compared to AgNO3. This stronger antibacterial action of AgNPs is ascribed to their substantial surface region that gives more surface contact with the microorganisms [Citation31]. Another significant explanation of the improved antibacterial action of AgNPs is the synergistic impact among particles and plant extract [Citation32].
MIC and MBC of AgNPs was additionally decided against the tested gram-positive and gram-negative bacteria ( and ). The MIC values for AgNPs against E. coli, MRSA, P. aeruginosa and S. mutans were 16, 32, 64 and 32 μg/mL, respectively. The MBC value for AgNPs against S. aureus and S. mutans was 64 μg/mL, while those for E. coli and P. aeruginosa were 32 and 128 μg/mL, respectively. Our results find support from the observations made with AgNPs synthesized from extract of Murayya koengii that demonstrated similar MIC and MBC values against MRSA and drug-resistant E. coli [Citation33]. The antibacterial activity associated with AgNPs is attributed to the release of silver ions, direct damage to the cell membrane and/or enhanced intracellular ROS generation [Citation20, Citation34].
Table 1. Antimicrobial activity of Ag-NPs.
Table 2. MIC and MBC determination.
Conclusions
In this examination, an eco-friendly technique was developed for biosynthesis of nanoparticles utilizing the plant extract of O. arabicus. The AgNPs were spheroidal with a molecule size of 5–20 nm. The AgNPs adequately restricted the growth of different microorganisms on Mueller Hinton agar plates with variable zones of inhibition. The MIC values against pathogenic microorganisms, E. coli, S. aureus, P. aeruginosa and S. mutans ranged from 16 to 64 μg mL−1. The produced AgNPs showed a wide effect against both gram-positive and gram-negative microorganisms. Green methodology for production of silver nanoparticles, particularly for antibacterial purposes against human microbes, opens another approach in antibiotic drug development. The present investigations clearly demonstrated the in vitro viability of AgNPs that can be utilized for effective application to MDR microscopic organisms after cautious in vivo examination.
Funding
The authors extend their appreciation to the Researcher Supporting Project No (RSP-2021/73) at King Saud University, Riyadh, Saudi Arabia.
Data available within the article or its supplementary materials
The authors confirm that the data supporting the findings of this study are available within the article [and/or] its supplementary materials.
Disclosure statement
No potential conflict of interest was reported by the authors.
References
- Albrecht MA, Evans CW, Raston CL. Green chemistry and the health implications of nanoparticles. Green Chem. 2006;8(5):417–432.
- Sastry M, Ahmad A, Khan MI, et al. Biosynthesis of metal nanoparticles using fungi and actinomycetes. Curr. Sci. 2003;85:162–170.
- Niraimathi KL, Sudha V, Lavanya R, et al . Biosynthesis of silver nanoparticles using Alternanthera sessilis (Linn.) extract and their antimicrobial, antioxidant activities. Colloids Surf B Biointerfaces. 2013;102:288–291.,
- Shanmugasundaram T, Radhakrishnan M, Gopikrishnan V, et al. A study of the bactericidal, anti-biofouling, cytotoxic and antioxidant properties of actinobacterially synthesised silver nanoparticles. Colloids Surf B Biointerfaces. 2013;111:680–687.,
- Fahimirad S, Ajalloueian F, Ghorbanpour M. Synthesis and therapeutic potential of silver nanomaterials derived from plant extracts. Ecotoxicol Environ Saf. 2019;168:260–278. −
- Haggag EG, Elshamy AM, Rabeh MA, et al. Antiviral potential of green synthesized silver nanoparticles of lampranthus coccineus and malephora lutea. Int J Nanomedicine. 2019;14:6217–6229., −
- Siddiqui MR, Rafiquee MZA, Wabaidur SM, et al. Synthesis of silver nanoparticle: a new analytical approach for the quantitative assessment of adrenaline. Anal Sci. 2015;31(5):437–443.
- Totaro P, Rambaldini M. Efficacy of antimicrobial activity of slow release silver nanoparticles dressing in post-cardiac surgery mediastinitis. Interact Cardiovasc Thorac Surg. 2009;8(1):153–154. −
- Wan Y, Guo Z, Jiang X, et al . Quasi-spherical silver nanoparticles: aqueous synthesis and size control by the seed-mediated Lee-Meisel method. J Colloid Interface Sci. 2013;394:263–268.,
- Begum NA, Mondal S, Basu S, et al. Biogenic synthesis of Au and Ag nanoparticles using aqueous solutions of black tea leaf extracts. Colloids Surf B Biointerfaces. 2009;71(1):113–118.,
- Chintamani RB, Salunkhe KS, Chavan MJ. Emerging use of green synthesis silver nanoparticle: an updated review. Int. J. Pharm. Sci. Res. 2018;9:4029–4055.
- Martin-Bravo S, Rad MA. Ochradenus arabicus (Resedaceae), a new record for the flora of Iran. Iran. J. Bot. 2010;16:84.
- Miller AG. A revision of ochradenus. Notes. Roy. Bot. Gard Edinburgh. 1984;41:491–594.
- Nadeem M, Al-Qurainy F, Khan S, et al. Effect of some chemical treatments on seed germination and dormancy breaking in an important medicinal plant of Ochradenus arabicus. Pak. J. Bot. 2012;44(3):1037–1040.
- Hussain J, Rehman NU, Khan AL, et al. Phytochemical and biological assessment of medicinally important plant Ochradenus arabicus. Pak. J. Bot. 2014;46:2027.
- Vincken J-P, Heng L, de Groot A, et al. Saponins, classification and occurrence in the plant kingdom. Phytochemistry. 2007;68(3):275–297.,
- Barakat HH, El-Mousallamy AMD, Souleman AMA, et al. Flavonoids of ochradenus baccatus. Phytochemistry. 1991;30(11):3777–3779.,
- Khan S, Al-Qurainy F, Nadeem M, et al. Development of genetic markers for ochradenus arabicus (Resedaceae), an endemic medicinal plant of Saudi Arabia. Genet Mol Res. 2012;11(2):1300–1308.
- Murashige T. Plant cell and organ cultures as horticultural practices. Acta Hortic. 1977;78(78):17–30.
- Nasser A, Husain FM, Nadeem M, et al. Bio-inspired facile fabrication of silver nanoparticles from in vitro grown shoots of Tamarix nilotica: explication of its potential in impeding growth and biofilms of Listeria monocytogenes and assessment of wound healing ability. RSC Adv. 2020;10(50):30139–30149.
- Maddinedi SB, Mandal BK, Maddili SK. Biofabrication of size controllable silver nanoparticles - A green approach. J Photochem Photobiol B. 2017;167:236–241.
- Seifipour R, Nozari M, Pishkar L. Green synthesis of silver nanoparticles using tragopogon collinus leaf extract and study of their antibacterial efects. J Inorg Organomet Polym. 2020;30(8):2926–2936.
- Ajitha B, Reddy YAK, Reddy PS. Biogenic nano-scale silver particles by Tephrosia purpurea leaf extract and their inborn antimicrobial activity. Spectrochim Acta A Mol Biomol Spectrosc. 2014;121:164–172.
- Iravani S, Korbekandi H, Mirmohammadi SV, et al. Synthesis of silver nanoparticles: chemical, physical and biological methods. Res Pharm Sci. 2014;9:385–406.,
- Ruíz-Baltazar D. J, Á, Reyes-López SY, Larrañaga D, et al. Green synthesis of silver nanoparticles using a Melissa officinalis leaf extract with antibacterial properties. Results Phys. 2017;7:2639–2643.:
- Shanmuganathan R, MubarakAli D, Prabakar D, et al. An enhancement of antimicrobial efficacy of biogenic and ceftriaxone-conjugated silver nanoparticles: green approach. Environ Sci Pollut Res Int. 2018;25(11):10362–10370.
- Vanaja M, Gnanajobitha G, Paulkumar K, et al. Phytosynthesis of silver nanoparticles by Cissus quadrangularis: influence of physicochemical factors. J. Nanostruct. Chem. 2013;3:1–8.
- Raghunandan D, Bedre MD, Basavaraja S, et al. Rapid biosynthesis of irregular shaped gold nanoparticles from macerated aqueous extracellular dried clove buds (Syzygium aromaticum) solution. Colloids Surf B Biointerfaces. 2010;79(1):235–240.
- Stehfest K, Toepel J, Wilhelm C . The application of micro-FTIR spectroscopy to analyze nutrient stress-related changes in biomass composition of phytoplankton algae. Plant Physiol Biochem. 2005;43(7):717–726.
- Sre PR, Reka M, Poovazhagi R, et al. Antibacterial and cytotoxic effect of biologically synthesized silver nanoparticles using aqueous root extract of Erythrina indica lam. Spectrochim Acta A Mol Biomol Spectrosc. 2015;135:1137–1144.
- Logeswari P, Silambarasan S, Abraham J. Synthesis of silver nanoparticles using plants extract and analysis of their antimicrobial property. J Saudi Chem Soc. 2015;19(3):311–317.
- Dur’An N, Dur’An M, de Jesus MB, et al. Silver nanoparticles: a new view on mechanistic aspects on antimicrobial activity. Nanomedicine. 2016;12(3):789–799.
- Qais FA, Shafiq A, Khan HM, et al. Antibacterial effect of silver nanoparticles synthesized using Murraya koenigii (L.) against multidrug-resistant pathogens. Bioinorg Chem Appl. 2019;2019:4649506 )
- Prabhu S, Poulose EK. Int. Nano Lett. 2012;2:32–41.