Abstract
Antibodies are key elements in routine immunological tests, having a long history in detection of plant pathogens due to their specificity, reliability and fast performance. Heavy chain-only antibodies (HCAbs), circulating in the blood of camels are IgG isotypes that lack the light (L) chains and the heavy (H) chain is depleted of the first constant domain (CH1). The HCAbs interact with the antigen by virtue of only one single variable domain, referred to as VHH or nanobody (Nb). Owing to their unique properties: nanometer scale size, robust structure, stable and soluble behaviour in aqueous solution, high affinity and specificity for only one cognate target, as well as a sustainable source, Nbs are an ideal research tool in plants. There are several rapid and robust techniques for isolating highly specific Nbs. Antigen-specific Nbs are selected from constructed nanobody libraries (immune, naïve, synthetic) and specific binders are retrieved by phage display, yeast display or ribosome display. Their small size, easy folding in monomeric units and the possibility to express Nbs in the cytosol, make them attractive for their application to engineer resistance against plant pathogens, and the effect of multiple Nbs combined by gene stacking to engineer plant resistance can be studied. We summarize the current methodologies for isolation and production of Nbs, their application in detection of plant pathogens and nanobody-mediated resistance against plant pathogens. In addition, we explore novel implementations of VHHs in agricultural research.
Supplemental data for this article is available online at https://doi.org/10.1080/13102818.2021.1974943 .
Introduction
Immunoglobulins (Ig), also known as antibodies (Ab), are produced by B lymphocytes and have the N- region with variable domains (V-region) for antigen binding and the C- terminal region for effector functions. Ig are arranged in 3 globular regions forming a Y-shaped architecture, have a molecular mass of approximately 150 KDa and are roughly 10 nm in size. Ig are tetrameric polypeptides composed of two identical pairs of H (50 kDa) and L polypeptide chains (25 kDa), linked by interchain disulphide bonds [Citation1]. The L chain consists of one variable domain (VL) at the N-terminal end and a single constant domain (CL) at the C-terminal end, whereas the H chain contains four or five domains: one variable domain (VH) at the N-terminal end followed by three or four constant domains (CH1, CH2, CH3, and possibly CH4) [Citation1] ()
Figure 1. Schematic representation of the conventional antibody, the antigen-binding domains of conventional antibodies obtained after proteolysis Fab or after cloning, and expression of the gene VH and VL fragments are shown. A synthetic linker introduced between the VH and VL stabilises the VH-VL dimer and forms the scFv.
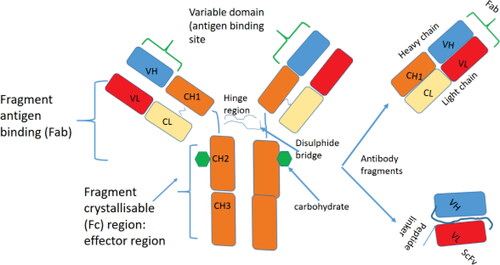
The Ab chains are synthesized after DNA rearrangements in B-cells and this process of joining gene segments contributes to the diversity of antigen binding sites [Citation2]. Several studies have shown that there are a large number of gene segments encoding the V region and a smaller number of exons encoding the C region. L-chain V region is encoded by a DNA sequence assembled from two gene segments—a long V germline gene segment and a short joining, or JL gene segment, whereas the H-chain V region is encoded by a DNA sequence assembled from three gene segments—a V germline gene segment, a JH segment and a diversity segment, or D gene segment. Further diversity of the V region is increased by imprecise joining of the gene segments which also occurs in conjunction with deletion or insertion of nucleotides at the splice junctions of the recombining VH-D-JH or VL-JL gene segments. Each B cell produces antibodies with an identical antigen binding site (monospecific).
Antibodies can be elicited against all possible targets, binding them with high affinity and specificity. Furthermore, antibodies can be produced in a monoclonal form. These properties make them a preferred tool in many applications in research, diagnosis and therapy. In addition, affinity purification of antibodies on protein A or protein G has been well established. The IgG class of antibodies is the most important for biotechnological and medical applications, since it is the most abundant isotype in the serum of mammals [Citation3].
Unfortunately, the large size and complex architecture of the antibodies impose practical limitations to their applications [Citation4]. In addition, the production process which involves the use of antiserum raised in mouse, rabbit, goat or chicken is time consuming, only available in limited amounts, of variable and unpredictable quality and can be costly due to the exhaustive tests required for quality checks in every batch produced [Citation5]. Upon expression of the recombinant antibody genes, they will not fold into antigen-binding entities in the reducing environments of the cytoplasm since disulphide bonds, needed for effective assembly, will not be formed [Citation6].
With the rapid development of molecular genetic engineering techniques, scientists developed monoclonal antibodies developed by hybridoma technology and antigen-binding fragments (Fabs, 50 KDa) and single-chain variable fragments (scFvs, 27 KDa) expressed in the periplasm of bacteria as an alternative to classical antibodies [Citation7]. The monoclonal antibody technology developed by Kohler and Milstein (1975) has been very significant in the development of diagnostics and human therapeutics [Citation8]. Despite its importance, the applicability of the technology on a wide scale suffers from practical limitations including: expensive production methods based on mammalian expression systems; inability to optimise the antibody using genetic engineering techniques; and the potential immunogenicity of the mouse antibody when administered to humans [Citation9]. The scFvs (), have a reduced affinity compared to the parent antibody, are less stable and have poor solubility.
With continued research and exploration, by serendipity scientists discovered a unique type of antibody with deviations from the well conserved conventional antibody found in the sera of mammalian species [Citation10]. The unique antibody type () is composed of the homodimer of the heavy chain antibody (HCAbs), naturally devoid of the light chain and occurring in the sera of Camelidae species (Camelus dromedarius, Camelus Bactrianus, Lama glama, Lama guanicoe, Vicugna pacos and Vicugna vicugna) [Citation3]. Genetic evidence shows that the Camelidae HCAbs evolved from adaptive changes occurring in the compartment of conventional antibodies and they are bona fide antibodies contributing to the immune response [Citation3].
Figure 2. Schematic representation of the Heavy chain antibody present in the Camelidae serum, lacking light chain and the CH1. The recombinant VHH is obtained after cloning and expression. VHH is the smallest intact antigen-binding fragment that can be obtained.
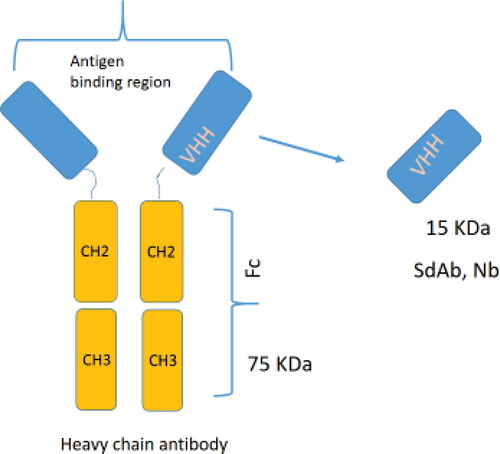
The antigen-binding fragment of the HCAb is composed of one single domain (the variable domain of the H-chain of HCAbs), referred to as VHH to distinguish it from the classic VH. Experimental evidence has proved that VHH is a monomeric single domain antigen binding entity, which was also named nanobody (Nb) due to its prolate shape and size in the nm range (diameter of ≈ 2.5 nm and height of ≈ 4 nm) [Citation10]. Therefore, nanobodies (Nbs) are the smallest intact antigen-binding fragments derived from antibodies. Nbs bind their antigens with good affinities in the range of 0.5 to 100 nmol/L [Citation11]. Due to their small size, single domain nature, Nbs can be easily cloned in phage display vectors, antigen binders selected by biopaning, and the selected Nbs are expressed in bacteria in high yields (2–10 mg/L in bacterial culture) in a soluble form [Citation11].
A study done by [Citation12] comparing the physical chemical properties of antigen-specific llama VHH fragments to antigen specific mouse monoclonal antibodies, showed the llama VHH to be highly stable being able to bind antigen specifically at temperatures as high as 90 °C. In addition, Nbs have been shown to retain their antigen-binding capabilities in the presence of ammonium thiocyanate and in the presence of up to 50% ethanol [Citation12]. The production of Nbs is cost effective, the production cost being reported as one-tenth the cost of producing monoclonal antibodies [Citation13].
Nbs are increasingly being used in diagnostics, therapeutics, and biotechnology due to their unique properties. Mostly, Nbs are used in applications similar to conventional antibodies. However, due to their unique and special properties, they have also been applied in settings where the use of conventional antibodies would be impractical or impossible. Nbs are amenable to genetic engineering due to their monomeric structure, hence different proteins can be fused with Nbs conveniently to achieve specificity, facilitating multifunctional molecular operation [Citation6]. Nbs can recognize cryptic concave epitopes, such as enzyme active sites, which are inaccessible for conventional antibodies [Citation14].
In this review, we summarize the Nb production approach, their biophysical properties and structural features as well as their application in the detection of plant pathogens and their antiviral properties against plant diseases.
Biophysical properties and structural features of nanobodies
Nanobodies represent the next-generation antibody-derived biologics due to their biophysical and biochemical properties with significant advances over conventional antibodies [Citation15]. Nb () is the recombinant single domain, antigen-binding variable region of the homodimeric HCAb. Conventional antibodies have a tedious production and purification process at a high cost. Their recombinant expression in microorganisms is not feasible due to their heterotetrameric structure. In addition, the cellular effects mediated by the Fc region are not needed in some applications such as engineering resistant plants with antibodies. However, smaller fragments can be engineered from conventional antibodies: such as the scFv (minimum recombinant antibody with antigen-binding activity, in which the VH and VL are connected by a flexible linker peptide), which can be produced in plants and in microorganisms. Unfortunately, during the construction of the scFv libraries, the original pairing of VH and VL as it was affinity matured in the animal is lost. Therefore, libraries constructed from polyclonal B cell populations will contain sizable numbers of scFvs composed of mispaired VH and VL that may be expressed poorly in recombinant systems, be unstable and be incapable of antigen recognition [Citation16].
HCAbs present no such drawbacks and can be minimized into a single domain (Nb) that can serve as a stand-alone recognition unit when expressed recombinantly as a single-domain protein, or when part of a fusion [Citation14]. The folding and stability of Nbs is less dependent on the formation of the disulphide bonds, hence they can be produced and function in the reducing cytoplasm of plant cells. Nanobodies have been expressed in the apoplast of tobacco with yields reaching up to 30% of total cell protein [Citation17]. In addition, for long-term storage of the Nbs, they can be produced in plant seeds which is effective, convenient, cost efficient and allows for transportation without cold chain maintenance [Citation18]. This is especially convenient in low economy settings with limited infrastructure.
Nbs fold efficiently and retain their functionality after chemical or thermal denaturation [Citation19]. Their stability is due to multiple factors such as the extended antigen binding loops or complementarity determining regions (CDR) and changes in amino acid compositions in the Framework region 2 (FR 2) [Citation3]. X ray crystallography studies have shown that FR2 of VH from conventional antibodies forms a conserved hydrophobic surface comprising Val47, Gly49, Leu50 and Trp52 to facilitate VH-VL pairing, which are substituted for more hydrophilic amino acids (Phe42, Glu49, Arg50 and Gly52) in VHH [Citation16].
The stability of Nbs gives significant advantages for their applications in food chemistry. For example, an immunoassay with high specificity, affinity, prolonged shelf life and the ability to withstand extended working periods in extreme environmental conditions based on Nbs was developed for detection of Salmonella [Citation20]. Salmonella enterica is a common cause of foodborne outbreaks, hence, sensitive and reliable detection of Salmonella in food and environmental sources is essential to safeguard food supply, as well as ensure public health [Citation20]. Recently, Nbs have been used to develop an immuno-assay for the detection of Staphylococcus aureus (S. aureus) in foods. In this case, a sandwhich enzyme-linked immunosorbent assay (ELISA) employing the Nb147 and biotinylated Nb147 pair was used to detect S. aureus with a detection limit of 1.4 × 105 colony forming units (CFU)/mL in milk [Citation21]. The thermal stability of the Nbs (high thermal stability with melting temperatures (Tm) ranging from 57 to 75 °C) was determined by a thermofluor assay in the CFX 370 ConnectTM Real-Time PCR Detection System [Citation21]. The use of conventional immunoglobulins for detection of S. aureus leads to false positives due to the unwanted and unavoidable interaction between the immunoglobulin (Ig) binding proteins on S. aureus and the crystallizable fragment (Fc) region of intact antibodies; however the use of Nbs overcomes this challenge.
Nbs can be easily engineered to construct multidomain constructs spaced by a linker such as a (G4S)3-5, or a natural hinge of an immunoglobulin (e.g. the llama IgG2c hinge or the human IgA hinge), due to their small size and monomeric behaviour [Citation22]. A compelling example includes Nb against the grapevine fanleaf virus that was fused to green fluorescent protein and expressed as a full length protein [Citation23]. Therefore, they can be applied in the study of the expression levels of plant proteins in vivo. In addition, Nbs can recognize epitopes that are usually not antigenic for classical antibodies, such as the catalytic site of enzymes and canyons in viral and infectious disease biomarkers. Interference with protein functioning has been demonstrated by generating an inhibitory VHH against potato starch branching enzyme [Citation24]. Upon targeting the VHH to potato plastids, the amylose content in tuber extracts increases due to specific VHH-based enzyme inhibition, and the increase is even higher compared to that of the antisense control lines [Citation25].
Nanobody production approach
Following the striking observation of the unique HCAbs in sera of Camelidae by Hamers-Casterman and colleagues in 1993 [Citation26], research has been done to optimize the isolation and production of the VHH for various applications and to characterize them (study the biochemical properties).
Heavy chain antibodies
HCAbs have been described in the sera of patients suffering from heavy chain disease and these are not functional in antigen binding. Functional heavy chain only antibodies have been described in the sera of Camelidae [Citation3]. Much research has focussed on the HCAbs found in the sera of Camelidae. Previous studies have reported the isolation of 3 distinct subclasses of IgG (IgG-1 contains conventional antibodies whereas IgG-2 and IgG-3 contains HCAbs) from the serum of immunized camels by differential absorption on Protein A and protein G [Citation26]. Purification of HCAbs and conventional Abs from different camelid species have reported the variability of their ratios among the camelid species [Citation27]. For example, the percentage of HCAbs and conventional IgG in camels might reach 50–80%, whereas in South American camelid species, it totals up to 10–25% [Citation28].
HCAbs recognize the antigen by one single domain known as VHH or single domain antibody (sdAb) () [Citation3]. Despite the lack of an associated light chain variable domain, VHHs have acquired important adaptations to remain soluble and functional [Citation29]. There are amino acid substitutions in the FR2 region to more hydrophilic amino acids [Citation10], therefore lowering its propensity to form a heterodimer with VL domain and also explaining the soluble behaviour of the VHH. The VHH paratope (that portion of an immunoglobulin or its fragments that interacts with the target antigen) is enlarged to achieve the requisite buried surface area when contacting antigen [Citation16]. These unique adaptations of VHH allow them to target enzymes, proteases and conserved inner regions of surface proteins from pathogens, frequently less accessible to the larger conventional Abs [Citation30].
Generation of nanobodies
To isolate Nbs with high affinity, several approaches have been proposed to prepare Nb libraries. Nbs can be isolated from an immune library, which is preferred because somatic maturation in lymphocytes of immunized Camelidae will give antibody libraries more specific and with higher affinity to antigens of interest. Alternatively, transgenic mice and rats that can be used for immunization as a first step to identify antigen specific HCAbs have been generated [Citation31]. Nbs can also be obtained from a naive library using blood samples from nonimmunised animals or the semisynthetic and synthetic libraries which saves on time or when the antigen is toxic to animals [Citation32]. In the immune library 106 individual clones per library is representative of the immune VHH repertoire of lymphocytes present in a small sample of blood from the immunized animal, while at least 109 individual clones are needed for the naive, synthetic and semisynthetic library to allow the retrieval of high-affinity binders to a given antigen [Citation32]. Antigen-specific Nbs are selected using various screening methods which are used based on the final application of the Nb. The screening methods used include: phage display, yeast display and ribosome display.
To generate a VHH immune library requires several steps which include: camel immunisation with antigen, recovery of peripheral blood lymphocytes and amplification of the VHH gene fragments by polymerase chain reaction (PCR) from the lymphocyte complementary deoxyribonucleic acid (cDNA), ligation of the VHH repertoire in a phage display vector and transformation in bacteria [Citation22]. Golden gate cloning and ligation of VHH to pMECS-GG vector have been reported to yield libraries that exceed 108 individual transformants and close to 100% of the clones harbour a phage display vector having a VHH insert [Citation33]. Moreover, multiple antigens can be mixed for immunization, and panning against several antigens can be performed in parallel [Citation11].
Phage display (a selection method that displays a recombinant protein on the phage surface while encoding its sequence within the phage) is commonly used to pan for Nbs. Selection of VHHs by phage display involves binding to antigen on an immobilized support with multiple rounds of adsorption and elution being performed to achieve significant enrichment of antigen-specific VHHs [Citation33]. After 2 to 4 rounds of panning, about 50% of the individual clones express antigen specific Nb as screened by enzyme-linked immunosorbent assays (ELISAs) [Citation31]. Nucleotide sequences of ELISA-positive clones are sequenced to deduce the amino acids of the Nbs. Nucleotide sequencing reveals multiple Nbs that can be categorized into approximately 5–10 families with Nbs of the same family sharing a homologous CDR3 sequence but might have a few different amino acids in their framework or even completely different CDR1 and/or CDR2 sequences [Citation31].
Yeast display is mainly applied for the identification of Nbs that bind to specific conformations of cell surface proteins, such as G protein–coupled receptors (GPCRs), and specific binders can be detected by flow cytometry (fluorescence-activated cell sorting, FACS) [Citation16]. Yeast display is particularly significant for those Nbs that are difficult to express in prokaryotic cells [Citation15]. Furthermore, once the sorting is finished, the cells can just be put in culture medium to proliferate; there is no Ag elution, neutralisation or infection step required as with phage display [Citation34]. Nbs that bind to cell membrane proteins in specific conformations are obtained using ribosome display where the translated Nb sequence and the RNA that encodes it remain tethered to the ribosome [Citation6].
Many other methods have been proposed and utilized to retrieve antigen-specific Nbs. For example [Citation35] demonstrated the production of high affinity Nbs against GFP (green fluorescent protein) and mCherry based on high-throughput DNA sequencing of a marrow lymphocyte VHH cDNA library from an immunised llama combined with mass spectrometric (MS) identification of high-affinity VHH regions derived from serum of the same animal. In this method, since HCAbs and conventional antibodies have different affinities to Protein A and Protein G, serial fractionation of the serum obtained from the immunised llama was performed to obtain HCAbs. The VHH containing fraction was affinity purified over an antigen coupled resin, and digested with papain (to cleave away the constant regions and leave behind the desired minimal fragments of the VHH variable region). Antigen bound VHH fragments were then eluted and separated by sodium dodecyl sulphate polyacrylamide gel electrophoresis (SDS-PAGE). The gel-purified bands were trypsin digested and analysed by liquid chromatography (LC)–MS and tandem MS (MS/MS).
An animal specific antibody sequence database was created from individual immunised llamas by high-throughput sequencing to create a searchable peptide database for MS analysis. Bioinformatics pipeline was developed to identify specific VHH sequences based on MS sequence coverage of CDR3 as well as CDR1 and CDR2 coverage, total VHH coverage, sequencing counts, mass spectral counts and the expectation values of matched peptides [Citation35]. Codon optimised genes for high probability hits for Nbs against the antigen of interest are synthesized and cloned in a bacterial expression vector; after which Nbs with robust expression, high and specific affinity for the antigen are selected [Citation35].
The bacterial 2-hybrid system has been proposed as a Nb selection system, which is significant when the Nbs selected are to be subsequently used as intrabodies [Citation34]. In this system, cells containing the plasmid antigen are transformed with the plasmid containing the Nb genes and then allowed to grow in selective media. The colonies are picked, and the Nb gene is amplified by PCR, followed by Restriction fragment length polymorphisms (RFLP) analysis; following nucleotide sequencing, the clones covering all RFLP patterns are amplified separately and recloned into an expression vector [Citation36].
In another study [Citation37] developed an innovative selection and identification technology, named NestLink, which centres on genetically encoded barcoding peptides, termed flycodes, which are designed for maximal detectability by mass spectrometry and support accurate deep sequencing. The flycodes are genetically linked to a library of binding proteins. In this approach, the Nb library (sequences amplified directly from the B- cells of immunised animal) is ligated to flycodes generating the nested library, which is purified and subsequently mixed with antigen before separation by size exclusion chromatography (SEC) [Citation37]. The flycodes are cleaved from the Nb-Antigen complex by proteases for identification by LC-MS/MS. Capturing and washing of the Nb-Ag complex and scoring the barcode frequency of occurrence in washed and retained fractions allowed the ranking of the binders with best koff rates [Citation34]. This technology was applied to identify Nbs as crystallisation chaperones for the bacterial ABC transporter TM287/288: the authors identified 9 Nbs with specific binding to the antigen and having affinities in the picomolar range (as reported by surface plasmon resonance (SPR) [Citation37]. Interestingly, this soluble monomeric barcoded Nb library opens the possibility for the selection of binders in tissues or in whole organisms.
Nanobodies for detection of plant pathogens and toxins
Antigen-specific Nbs are readily identified after a short immunisation of a llama, a camel or a dromedary with antigen in the presence of adjuvant, cloning of the VHH repertoire from the immunised animal in a phage display vector after RT-PCR of the drawn blood with VHH-specific primers in a nested PCR protocol and identification of individual clones producing virions harbouring the antigen-specific VHH following several rounds of panning against the antigen of interest [Citation33]. The good expression in microbial systems and the beneficial biochemical properties (good solubility, good stability in harsh conditions, high affinity and specificity for the antigen, small size and strict monomeric behaviour) [Citation10] make Nbs an ideal tool for immunodetection.
Recently, Nbs have been gaining attention for application in the detection of plant derived toxins and plant pathogens (). The genes of crystalline (Cry) toxins have been widely applied in transgenic rice, corn and cotton for pest control against lepidopteran and coleopteran insects. Unfortunately, their residues have been detected in transgenic plants and have been shown to be harmful to human health and the environment [Citation38]. Therefore, it is essential that scientists monitor and control the levels of these toxins. Hence, Nbs have been isolated for the sensitive and specific detection of various types of Cry toxins. A novel sandwich ELISA for Cry1B detection based on the biotin–streptavidin system using Nbs has been established with high specificity (low limit of detection 3.46 ng/mL, lower than that of other conventional sandwich ELISAs) and good thermal stability (average value of melting temperature (Tm) of the Nbs was 65 °C) [Citation38]. A Nb-based immunoassay for monitoring trace levels of 2,4-dichlorophenoxyacetic acid (2,4-D), (a widely used herbicide in agriculture and forestry) have been established with no cross-reactivity to 2,4-D analogues [Citation39].
Table 1. Examples of application of Nbs as a diagnostic tool in agriculture.
In addition to detection of toxins, Nbs are also isolated for the early detection of plant pathogens, which is significant for monitoring of plant pathogens in farmers’ fields, seed fields, epidemiological studies, exchange of disease-free materials across regions and globally, for effective disease management and prevention. Tulip virus X (TuVX) is a positive-stranded RNA virus affecting tulips and is considered by most governments as a pathogen of potential quarantine concern on flower bulb imports [Citation5] isolated Nbs for immunological detection of TuVX by immunising an alpaca with TuVX particles. The isolated Nbs were sensitive for TuVX and distinguished TuVX-infected tulip leaf material from uninfected leaves with no cross-reactivity to related Flexiviridae, maintained their reactivity upon storage at −20 °C for over a year and retained activity after several weeks of storage at 37 °C [Citation5].
In another research, Nbs were isolated against the coat protein (CP) of Zucchini yellow mosaic virus (ZYMV). ZYMV is a member of the genus Potyvirus in the family Potyviridae and is a commonly detected virus in cucurbit species in Saudi Arabia. ZYMV has been reported to result in complete yield loss when it occurs early in the season [Citation40] isolated Nbs against the ZYMV-CP by phage display panning of a naïve nanobody library with good reactivity and specificity to ZYMV infected leaves [Citation40]. These advancements show potential for the use of Nbs to develop versatile and affordable diagnostic assays to detect plant pathogens and toxins. In addition, owing to the robustness of Nbs, they can be easily tailored to develop lateral flow assay for use in field tests in remote settings.
Engineering resistant plants with nanobodies
Plant pathogens cause significant economic losses and reduce food security by reducing the yield and quality of agricultural production [Citation41]. Development of virus-resistant varieties is an economically viable and environmentally sustainable approach for disease control ([Citation42]Beyene et al., 2017). Therefore, there is need to develop effective and sustainable strategies to control plant diseases. Experiments reported that plants expressing an ectopic antibody targeting the viral structural coat protein could provide plant resistance against the cognate virus [Citation43]. In addition, antibody targeting conserved domain in the viral polymerase have been reported to provide plant viral resistance. For example, [Citation44] reported high levels of resistance against infections with Tomato bushy stunt virus (TBSV) in transgenic N. benthamiana lines expressing scFvs directed against the conserved motif E of viral RNA-dependent RNA polymerases.
The lengthy process to generate scFvs and their low stability in the plants cytosol limits their use in developing virus resistant plants. Fortunately, Nbs can be used as an interesting alternative. Nbs, because of their single domain nature, are less dependent on cellular chaperones and more tolerant to reducing environments. The latter property is of particular importance when Nbs are applied in establishing resistance against plant pathogens (to reduce crop loss) as plant viruses are generally located within the cytosol [Citation40]. The mechanisms by which Nb mediate resistance in plants include: Nb binding to the key proteins of pathogens preventing their cell attachment, invasion, reproduction and movement, thus interrupting their life cycles, or by directly neutralizing the toxins secreted by pathogens, reducing the damage to plants [Citation14].
Broad bean mottle virus (BBMV), of the genus Bromovirus is a seed-borne plant virus infecting Cicer arietinum (chickpea), Pisum sativum (field pea) and Vicia faba (faba bean or broad bean), causing possible loss in grain yield of 35–55%, depending on the time of infection [Citation43] Ghannam et al. [Citation43] isolated Nbs against BBMV by phage display and panning following the immunisation of C. dromedarius with glutaraldehyde-treated BBMV mixed with adjuvant. The authors demonstrated that in vitro pre-incubation of the identified BBMV specific Nbs (NbBBMV01, NbBBMV12 or NbBBMV22) and their viral target inhibited the BBMV infectivity and arrested its spreading in plants. This was demonstrated by lack of symptoms of viral infection (15 days post mechanical inoculation), in plants pre-blocked with any of the three nanobodies [Citation43]. Wild type V. faba plant leaves were infiltrated with agro- bacteria containing a vector expressing anti-BBMV nanobody genes under the control of CaMV 35S promoter. The expressed Nbs were shown to be able to reduce in vivo virus infectivity probably by direct contact between BBMV and the cognate nanobody [Citation43].
Nb (Nb23) stably expressed in Nicotiana benthamiana (model plant) as well as in the grapevine rootstock (natural host of the virus) were shown to have strong and specific resistance against Grapevine fanleaf virus (GFLV), which is a nematode-transmitted picorna-like plant virus affecting vineyards worldwide [Citation23]. Seven transformed lines encoding Nb23:EGFP (transgenic rootstocks of the 41B genotype) were used to experimentally test the antiviral activity of Nb23. Epifluorescence imaging of leaves revealed the accumulation of the Nb all over the leaf surface but highly concentrated in the vasculature, while the immunoblotting results revealed the expression of a full-length protein. Resistance was assessed by micrografting of transgenic canes onto GFLV-infected grapevine material. GFLV remained undetectable in all canes from lines expressing Nb23:EGFP by DAS-ELISA [Citation23].
The structure of GFLV-Nb23 was determined by high-resolution single particle cryo-electron microscopy (Cryo-EM) to gain molecular insights into the mechanism of GFLV recognition by Nb23 [Citation45]. CDR2 and CDR3 of Nb23 interacts with the domains A, B and C of the GPFV capsid protein (CP) by inducing conformational changes. In addition, the interface of Nb23-CP interaction also involves the C-terminal CP residue Val504 that is recognized by Lys54Nb23 [Citation45]. GFLV was detected at only low frequency (3.2%) in Nicotiana benthamiana line (23EG38-4) expressing Nb23:EGFP [Citation23]. The resistance breakdown was due to mutations at the C-terminus of the CP; however, through High throughput sequencing, these mutations were shown to pre-exist in natural viral populations at low frequencies as part of the viral quasispecies [Citation45]. These mutations were also shown to interfere with GFLV transmission by nematodes [Citation45]. Since Nbs have been shown to demonstrate antiviral activity against plant pathogens, they can be considered as a reliable source of resistance against plant pathogens including viruses, bacteria and fungi. There is huge potential for development of Nb based resistant plants and for their commercialisation ().
Table 2. Representative applications of nanobodies for engineering virus-resistant plants.
Nanobody based products in the market (agricultural application)
Engineered antibodies account for > 30% of the biotechnology market [Citation4]. Single domain antibodies are stable, soluble and can be expressed in high quantities in microbial or plant systems. As a result of the beneficial properties of these stable recombinant entities, they are currently highly valued proteins for multiple applications, including resistance ().
Table 3. Examples of nanobody based products in agricultural application.
Agrosavfe (http://www.agrosavfe.be/), a company established in 2013 (now called Biotalys), aims to a more sustainable food supply by transforming food and crop protection with unique, effective and safe protein-based biocontrol solutions. It exploits Nbs as they can withstand harsh field conditions, such as variable pH, temperature and salt concentrations as carriers for the efficient and more specific delivery of existing chemicals to crops and weeds [Citation47]. The agrocapsules are engineered covalently coupled to plant specific VHHs (specifically bind trichomes, stomata, cuticle, lenticels, thorns, spines, root hairs or wax layer), which improve the chemical retention time at the target site, therefore reducing the amounts of chemicals used [Citation48]. Microcapsules with antigen-specific VHH covalently linked to carboxyl or amine anchor groups of microcapsules by means of different linking methods are specifically binding to leaves and are suitable for delivery of agrochemicals on greenhouse or field crops in the range of 24 gAS/ha to 8.5 kgAS/ha [Citation46]. The agrocapsules approach has been utilized to develop a biofungicide (BioFun-1) that demonstrated a dose-dependent control of economically important plant diseases like Botrytis and powdery mildew in the open field trials. The biofungicide is expected to be released in the market by 2022, in the USA before being marked globally.
The agrocapsule approach has also been applied for the VHH-based specific delivery of insecticides to insects. A study by [Citation46] identified VHH capable of binding to the insect surface and can be used to bind and retain a compound, preferably a biologically active agent, to the insect by isolating antigen binding domains from llamas, immunized with complex insect homogenates and then selecting the antigen binding domains on entire, intact insects. To pursue good efficacy, the VHH phage selections are performed against living aphids and even whole-insect ELISAs have been developed for subsequent VHH characterisation translated [Citation47].
Fall armyworm (FAW, Spodoptera frugiperda J.E. Smith) is an invasive lepidopteran pest established in most of sub-Saharan Africa since 2016 [Citation49], and yield losses due to FAW infestation of 32% in Ethiopia and 47% in Kenya have been reported [Citation50]. Pesticide use is the most used approach for fall armyworm management, with a prevalent tendency for farmers not to adhere to safety precautions. In addition, several studies have suggested the limited efficacy of pesticides in controlling this pest or reducing its damage and impacts [Citation51]. Therefore, use of FAW specific VHH and agrocapsule delivery of the biologically active compound against the pest would be an area of interest to be explored that would minimise the amount of pesticide used for the management of FAW.
Animal African trypanosomiasis (AAT) is widespread in Sub-Saharan Africa, where it continues to impose a heavy socio-economic burden as it renders the development of sustainable livestock rearing very strenuous. Sensitive and specific diagnostics are needed for the identification of infected animals prior to initiation of drug treatment and also after treatment to test the effectiveness of the treatment (test of cure tests). Immunisation of an alpaca with a secretome mix from two T. congolense strains resulted in the identification of a Nb pair targeting the pyruvate kinase enzyme (Nb44/Nb42) that was used for the development of a lateral flow assay (LFA) that can be employed to detect parasitaemia in plasma samples from experimentally infected mice and cattle [Citation52]. Further studies are needed for the scale-up of these results and commercialization of the rapid tests, as they are of primary interest in the healthcare context where results can be rapidly available.
The toolbox of Nb-based products needs further development for target validation and to facilitate cutting-edge science. Additional developments for Nbs as highly specific capturing agents for development of sensitive, specific diagnostics for phytosanitary applications (facilitate safe exchange of germplasms), epidemiological studies, germplasm screening, monitoring of plant pathogens in farmers’ fields, seed lots and seed fields are needed.
Production of Nb based therapeutics in plants (molecular farming)
There has been much research done to study the application of Nbs in human diseases as therapeutics and for targeted drug delivery. For example, a lactamase fusion construct comprising a Nb directed against carcino-embryonic antigen (CEA), a human tumour marker was shown to eradicate solid tumours in mice, while a construct of the truncated ApoLI attached to a Nb that recognizes the Trypanosome rhodesiensis, (a parasite that causes sleeping sickness in East Africa), generated a strong trypanolytic compound that eliminated the parasites from blood [Citation53]. The production of the Nbs for use as human therapeutics are mostly produced in conventional mammalian manufacturing platforms. However, with the introduction of novel molecular biology tools and the landscape of antibody discovery ever expanding, plant expression systems are gaining traction among scientists.
Plant expression systems are suitable for manufacturing Nbs which are difficult to express or commercially unviable in mammalian expression systems or requiring rapid production in case of public health emergencies. For example, secretory IgAs which are better suited for protection at the mucosal surfaces are difficult to produce in mammalian expression systems due to their inherent complex nature. However [Citation18] successfully produced anti enterotoxigenic Escherichia coli (ETEC) VHHs grafted onto porcine IgA in Arabidopsis thaliana seeds. In addition, the seeds are easy to store, and the antibodies in the seeds are stable for more than five years [Citation18].
Passive immunity is the prophylaxis of choice in highly endemic areas where vaccine responses may be poor or in selected groups of patients (hospitalized children, immunocompromised individuals, and older infants and children) in whom vaccination is contraindicated and is provided when a person is given antibodies to a disease rather than producing them through his or her own immune system. Rotavirus is a leading cause of diarrhea in infants and young children worldwide and only 2 vaccines are available in the market licensed for use only within a very narrow age window (>6 weeks and ≤26 weeks of age) to avoid the risk of intussusception [Citation54]. Nbs have a high binding capacity, are resistant to pepsin, acid, heat and can be produced as a recombinant protein with an intact spatial structure due to their small size. With the same rationale, Tokuhara et al. [Citation54] developed llama VHH against rotavirus (ARP1). Using a binary vector ARP1 gene combined with a RNAi suppression cassette was transfected into rice plants using Agrobacterium tumefaciens–mediated transformation to produce transgenic rice (MucoRice-ARP1) [Citation54].
MucoRice-ARP1 was produced at high yields (11.9% of total protein in rice seeds) and it was shown to be highly water soluble. In addition, using mass spectrometry it was shown to contain no aminoacid modifications (Tokuhara et al., 2013). The authors proved that oral administration of MucoRice-ARP1 powder provided protection against rotavirus in both immunocompetent and immunodeficient mice (Tokuhara et al., 2013). Therefore, plant expression systems which are easily scalable are an efficient system for the production of VHHs for application as therapeutics.
Conclusions
Nanobodies represent the next-generation antibody-derived biologics with significant advantages over conventional antibodies. Nb expression is less dependent on the formation of disulphide bonds, hence can be produced and function in the reducing cytoplasm of plant cells, which is not conducive to disulphide bond formation. Therefore, Nbs can be expressed in plant leaves, which facilitates easy scale-up and low risk of contamination, or in plant seeds, which enables the immunogenicity to be preserved during long-term storage and transportation, even at ambient temperature with high protein concentrations.
Plants can be used in production of Nbs (molecular farming) for application in diagnostics and therapeutics, as evidenced by some studies in animals. Hence, plants are considered excellent expression systems for therapeutic proteins especially during the outbreak of a pandemic when therapeutics and vaccines are urgently needed.
Nanobodies are promising tools for application in plant pathogen detection and can be directed against key proteins of plant pathogens and interfere with their life cycles and enhance plant resistance. Currently, only limited studies have been done in regard to the application of Nbs as detection agents, and much research is needed. In addition, the application of Nbs against plant pathogens has focussed on BBMV and GFLV (viral pathogens). More applications aiming at viruses, bacteria and fungi are worth looking forward to. In addition, with the original patents on Nbs encompassing the structure, composition and use-specific applications of the single domain antibodies (regardless of which Camelidae host is used) having expired in Europe in 2014 and in the USA in 2017 (https://abcore.com/arent-llama-antibodies-patented/), more academic and industrial groups are expected to explore innovative applications with nanobodies.
Acknowledgements
The author (F.N…) would like to acknowledge the Partnership for Skills in Applied Sciences, Engineering and Technology (PASET), Regional Scholarship and Innovation Fund, (RSIF) for support for my Ph.D. project. I (Faith Njeru…) acknowledge Prof. Serge MUYLDERMANS for review of the paper.
Data availability statement
The authors confirm that the data supporting the findings of this study are available within the articles referenced and their supplementary materials.
Disclosure statement
No potential conflict of interest was reported by the author(s).
References
- Hsu DC. Janeway’S immunobiology. Shock. 2008;29(6):770.
- Alberts B, Johnson A, Lewis J, et al. Molecular Biology of the Cell. 4th edition. New York: Garland Science; 2002. The Generation of Antibody Diversity. Available from: https://www.ncbi.nlm.nih.gov/books/NBK26860/.
- Vincke C, Muyldermans S. Introduction to heavy chain antibodies and derived nanobodies. Methods Mol Biol. 2012;911(1):15–26.
- Holliger P, Hudson PJ. Engineered antibody fragments and the rise of single domains. Nat Biotechnol. 2005;23(9):1126–1136.
- Beekwilder J, Van Houwelingen A, Van Beckhoven J, et al. Stable recombinant alpaca antibodies for detection of tulip virus X. Eur J Plant Pathol. 2008;121(4):477–485.
- Cheloha RW, Harmand TJ, Wijne C, et al. Exploring cellular biochemistry with nanobodies. J Biol Chem. 2020;295(45):15307–15327.
- Yan J, Wang P, Zhu M, et al. Characterization and applications of nanobodies against human procalcitonin selected from a novel naïve nanobody phage display library. J Nanobiotechnol. 2015;13(1):1–11.
- Köhler G, Milstein C. Continuous cultures of fused cells secreting antibody of predefined specificity. Nature. 1975;256(5517):495–497. doi:10.1038/256495a0. 1172191
- Kuus R. Will immunogenicity limit the use, efficacy, and future development of therapeutic monoclonal antibodies?. Clin Diagn Lab Immunol. 1994;4(1):365–372. doi:10.1128/cdli.1.4.365-372.1994.
- Muyldermans S, Baral TN, Retamozzo VC, et al. Camelid immunoglobulins and nanobody technology. Vet Immunol Immunopathol. 2009;128(1-3):178–183.
- Arbabi Ghahroudi M, Desmyter A, Wyns L, et al. Selection and identification of single domain antibody fragments from camel heavy-chain antibodies. FEBS Lett. 1997;414(3):521–526.
- Van Der Linden RHJ, Frenken LGJ, De Geus B, et al. Comparison of physical chemical properties of llama V(HH) antibody fragments and mouse monoclonal antibodies. Biochim Biophys Acta Protein Struct Mol Enzymol. 1999;1431(1):37–46.
- Wolfson W. Ablynx makes nanobodies from llama bodies. Chem Biol. 2006;13(12):1243–1244.
- Wang W, Yuan J, Jiang C. Applications of nanobodies in plant science and biotechnology. Plant Mol Biol. 2021;105(1-2):43–53.
- Liu W, Song H, Chen Q, et al. Recent advances in the selection and identification of antigen-specific nanobodies. Mol Immunol. 2018;96:37–47. (November 2017),
- Ingram JR, Schmidt FI, Ploegh HL . Exploiting nanobodies’ singular traits. Annu Rev Immunol. 2018;36(January):695–715.
- Teh YHA, Kavanagh TA. High-level expression of camelid nanobodies in Nicotiana benthamiana. Transgenic Res. 2010;19(4):575–586.
- Virdi V, Juarez P, Depicker A. Plant expression systems for early stage discovery and development of lead therapeutic antibodies. Hum Antibodies. 2015;23(3-4):37–43.
- Dumoulin M, Conrath K, Van Meirhaeghe A, et al. Single-domain antibody fragments with high conformational stability. Protein Sci. 2009;11(3):500–515.
- He Y, Ren Y, Guo B, et al. Development of a specific nanobody and its application in rapid and selective determination of Salmonella enteritidis in milk. Food Chem. 2020;310:125942
- Hu Y, Sun Y, Gu J, et al. Selection of specific nanobodies to develop an immuno-assay detecting Staphylococcus aureus in milk. Food Chem. 2021;353:129481
- Vincke C, Gutiérrez C, Wernery U, et al. Generation of single domain antibody fragments derived from camelids and generation of manifold constructs. Method Mol Biol (Clifton, N.J.). 2012;907:145–176.
- Hemmer C, Djennane S, Ackerer L, et al. Nanobody-mediated resistance to grapevine fanleaf virus in plants. Plant Biotechnol J. 2018;16(2):660–671.
- Jobling SA, Jarman C, Teh MM, et al. Immunomodulation of enzyme function in plants by single-domain antibody fragments. Nat Biotechnol. 2003;21(1):77–80.
- Anamika W. Inception of nano bodies as implantation of targeted protein degradation: Protein-Interference rejuvenated – a review. SSRN Electron J. 2020. doi:10.2139/ssrn.3549080.
- Hamers-Casterman C, Atarhouch T, Muyldermans S, et al. Naturally occurring antibodies devoid of light chains. Nature. 1993;363(6428):446–448.
- Blanc MR, Anouassi A, Abed MA, et al . A one-step exclusion-binding procedure for the purification of functional heavy-chain and mammalian-type gamma-globulins from camelid sera. Biotechnol Appl Biochem. 2009;54(4):207–212.
- Haddad M, Soukkarieh C, Khalaf HE, et al. Purification of polyclonal IgG specific for camelid’s antibodies and their recombinant nanobodies. Open Life Sciences. 2016;11(1):1–9.
- Salema V, Fernández LÁ. Escherichia coli surface display for the selection of nanobodies. Microb Biotechnol. 2017;10(6):1468–1484. doi:10.1111/1751-7915.12819.
- Muyldermans S . Nanobodies: Natural single-domain antibodies. Annu Rev Biochem. 2013;82(1):775–797.
- Muyldermans S. Applications of nanobodies. Annu Rev Anim Biosci. 2021b;9:401–421.
- Wang Y, Fan Z, Shao L, et al. Nanobody-derived nanobiotechnology tool kits for diverse biomedical and biotechnology applications. Int J Nanomedicine. 2016;11:3287–3303.
- Romão E, Poignavent V, Vincke C, et al. Construction of high-quality camel immune antibody libraries. Methods Mol Biol. 2018;1701:169–187.
- Muyldermans S. A guide to: generation and design of nanobodies. FEBS J. 2021;288(7):2084–2102. doi:10.1111/febs.15515.
- Fridy PC, Li Y, Keegan S, et al. A robust pipeline for rapid production of versatile nanobody repertoires. Nat Methods. 2014;11(12):1253–1260.
- Pellis M, Pardon E, Zolghadr K, et al. A bacterial-two-hybrid selection system for one-step isolation of intracellularly functional nanobodies. Arch Biochem Biophys. 2012;526(2):114–123.
- Egloff P, Zimmermann I, Arnold FM, et al. Engineered peptide barcodes for in-depth analyses of binding protein libraries. Nat Methods. 2019;16(5):421–428.
- Zhong W, Li G, Yu X, et al. Sensitive detection of Bacillus thuringiensis Cry1B toxin based on camel single-domain antibodies. MicrobiologyOpen. 2018;7(4):1–7.
- Li ZF, Dong JX, Vasylieva N, et al. Highly specific nanobody against herbicide 2,4-dichlorophenoxyacetic acid for monitoring of its contamination in environmental water. Sci Total Environ. 2021;753:141950
- Zakri AM, Al-Doss AA, Sack M, et al. Cloning and characterisation of nanobodies against the coat protein of zucchini yellow mosaic virus. Plant Protection Science. 2018;54(4):215–221.
- Beyene Y, Gowda M, Suresh LM, et al. Genetic analysis of tropical maize inbred lines for resistance to maize lethal necrosis disease. Euphytica. 2017;213(9). doi:10.1007/s10681-017-2012-3.
- Savary S, Willocquet L, Pethybridge SJ, et al. The global burden of pathogens and pests on major food crops. Nat Ecol Evol. 2019;3(3):430–439.
- Ghannam A, Kumari S, Muyldermans S, et al. Camelid nanobodies with high affinity for broad bean mottle virus: a possible promising tool to immunomodulate plant resistance against viruses. Plant Mol Biol. 2015;87(4-5):355–369.
- Boonrod KJ, Galetzka D, Nagy PD, et al. Single-chain antibodies against a plant viral RNA-dependent RNA polymerase confer virus resistance. Nat Biotechnol. 2004;22(7):856–862.
- Orlov I, Hemmer C, Ackerer L, et al. Structural basis of nanobody recognition of grapevine fanleaf virus and of virus resistance loss. Proc Natl Acad Sci U S A. 2020;117(20):10848–10855.
- Verheesen P, De Jonghe C. Manufacturing of specifically targetting microcapsules. WO Patent 2,013,050,594.
- De Meyer T, Muyldermans S, Depicker A. Nanobody-based products as research and diagnostic tools. Trends Biotechnol. 2014;32(5):263–270.
- Jongedijk E, Verheesen P. 2013. Specific delivery of agrochemicals. Patent No US 8.598,081 B2
- Baudron F, Zaman-Allah MA, Chaipa I, et al. Understanding the factors influencing fall armyworm (Spodoptera frugiperda J.E. Smith) damage in African smallholder maize fields and quantifying its impact on yield. A case study in Eastern Zimbabwe. Crop Prot. 2019;120(June):141–150.
- Groote HD, Kimenju SC, Munyua B, et al . Spread and impact of fall armyworm (Spodoptera frugiperda J.E. Smith) in maize production areas of Kenya. Agric Ecosyst Environ. 2020;292:106804(December 2019),
- Kansiime MK, Mugambi I, Rwomushana I, et al. 2019. Farmer perception of fall armyworm (Spodoptera frugiderda J. E. Smith) and farm-level management practices in Zambia, (May)..
- Torres JEP, Goossens J, Ding J, et al. Development of a nanobody-based lateral flow assay to detect active trypanosoma congolense infections. Scientific Reports, (April). 2018;(1):15.
- Baral TN, Magez S, Stijlemans B, et al. Experimental therapy of african trypanosomiasis with a nanobody-conjugated human trypanolytic factor. Nat Med. 2006;12(5):580–584. doi:10.1038/nm1395. 16604085
- Tokuhara D, Álvarez B, Mejima M, et al. Rice-based oral antibody fragment prophylaxis and therapy against rotavirus infection. J Clin Invest. 2013;123(9):3829–3838. doi:10.1172/JCI70266. 23925294