Abstract
This study investigated the performance of GE11 peptide-modified superparamagnetic iron oxide nanoparticles (SPION) targeting the epidermal growth factor receptor (EGFR) on the surface of hepatocellular carcinoma (HCC) cells for magnetic resonance imaging (MRI) diagnosis in HCC. GE11-CSO-SPION micelles were prepared and characterized. MTT (3-(4,5-Dimethylthiazol-2-yl)-2,5-diphenyltetrazolium bromide) was used to analyze micellar cytotoxicity. Fluorescence analysis of cellular uptake was used to analyze the targeting of GE11 peptides to liver cancer cells. Prussian blue staining was used to observe the binding of cells and micelles, and the imaging ability was tested by MRI in vitro and in vivo. GE11-CSO-SPION micelles were spherical, with uniform size and no obvious aggregation of nanoparticles. No obvious micellar cytotoxicity was observed. Cellular uptake showed that GE11 peptide was specific to SMMC-7721 cells and HepG2 cells with high EGFR expression. Prussian blue staining showed that the uptake of SPION micelles by HepG2 cells was good. In vitro and in vivo MRI showed that GE11-CSO-SPION micelles had good imaging effects. GE11-CSO-SPION micelles can be used as an MRI contrast agent to detect HCC with high EGFR expression, providing reliable data for early diagnosis of HCC.
Introduction
Hepatocellular cancer (HCC) is the fifth common cancer and the fourth leading cause of cancer-related deaths worldwide [Citation1,Citation2]. It also has increasing morbidity and mortality. The 5-year survival rate of HCC is low due to unsatisfactory early diagnosis and poor prognosis. Therefore, the early diagnosis of HCC has important clinical significance [Citation3]. MRI characterized by non-radiation damage, noninvasiveness, good soft tissue contrast, high temporal resolution and spatial resolution images has been widely used in early detection of HCC. However, conventional contrast agents have low specificity and high-dose contrast agents are required. The contrast agents may accumulate in tissues and induce toxic effects. Therefore, targeted contrast agents are needed in clinic.
In recent years, superparamagnetic iron oxide nanoparticle (SPION) as a contrast agent for MRI T2 sequence has attracted much attention in the field of molecular imaging, which has good superparamagnetism and biocompatibility [Citation4,Citation5]. It can be degraded and removed from the circulation through the endogenous iron metabolism pathway. The synthesis method of SPION is simple; its size and shape can be adjusted; and its surface properties can be easily modified [Citation6–8]. Nano-scale SPION can be accumulated in tumor sites through passive targeting resulting from enhanced permeability and retention effect (EPR) of solid tumors [Citation9]. Moreover, SPION has been approved by the United States Food and Drug Administration (FDA) for clinical diagnosis [Citation10].
Polymer micelles, which can accumulate at tumor sites through the EPR effect of tumors, have received widespread attention. They can be modified through their surface to achieve active targeting of tumor tissues. Surface modification of nanoparticles is very important for improving the EPR effect and reducing the aggregation of nanoparticles and proteins in body fluids [Citation11]. Chitosan oligosaccharide (CSO) is a naturally occurring, low-cost cationic polysaccharide with a variety of functional groups on the surface, which makes it convenient for modification [Citation12]. Its sustained/controlled release characteristics enable chitosan to load imaging agents and therapeutic drugs or conjugate with other polymer nanoparticles [Citation13]. Targeting peptides are used to modify polymer micelles due to their ease of synthesis, small size, low immunogenicity and strong tumor penetration [Citation14]. Some targeting peptides have been approved by the FDA to be used in patients [Citation15]. Targeting molecules are very important in the design of molecular imaging probes, which should be highly expressed in cancer cells, but not present or under-expressed in normal adjacent tissues [Citation16]. Epidermal growth factor receptor (EGFR) is a transmembrane receptor tyrosine kinase that is activated by several ligands, leading to the activation of multiple signaling pathways for proliferation, differentiation and survival [Citation17]. It is highly expressed in various epithelial tumors, such as non-small cell lung cancer, kidney cancer, breast cancer, and head and neck squamous cell carcinoma [Citation18]. EGFR plays an important role in the development of liver cancer, which can prompt the aggressiveness of HCC [Citation19]. It has been shown that the dodecapeptide GE11 (YHWYGYTPQNVI) has high affinity for EGFR and can specifically target SMMC-7721 cells and HepG2 cells with high expression of EGFR on the cell surface [Citation20, Citation21]. However, GE11 peptide does not induce the activation of EGFR signaling pathway [Citation22].
In this study, GE11 was used as a targeting peptide to link CSO-SPION to form GE11-CSO-SPION micelles. The GE11-CSO-SPION micelles were characterized and evaluated. They were used to target liver cancer SMMC-7721 and HepG2 cell lines. Their cytotoxicity, targeting ability and MRI imaging effect in vitro and in vivo were evaluated.
Materials and methods
Cells and reagents
L-O2 cells were obtained from the American Type Culture Collection (ATCC, USA). HepG2 and SMMC-7721 cells were purchased from Jikai Gene Co., Ltd. (Shanghai, China). All cells were cultured in an incubator with a temperature of 37 °C and a CO2 concentration of 5%. L-O2 and SMMC-7721 cells were cultured with RPMI1640 medium supplemented with 10% fetal bovine serum and penicillin/streptomycin (100 U mL−1/100 U mL−1), while HepG2 cells were cultured with Dulbecco’s Modified Eagle medium (DMEM) supplemented with 10% fetal bovine serum and penicillin/streptomycin (100 U mL−1/100 U mL−1).
The 25% trypsin digestion solution and DMEM were from HyClone. RPMI1640 medium was from Gibco and fetal bovine serum was from Biological Industries Israel Beit Haemek Ltd; Chitosan and Prussian blue staining kit were purchased from Solarbio Science & Technology Co., Ltd (Beijing, China); 3-(4,5-Dimethylthiazol-2-yl)-2,5-diphenyltetrazolium bromide (MTT), 1.5 mol L−1 Tris-HCL (pH 8.8), and 1 mol L−1 Tris-HCL (pH 6.8) were purchased from Beyotime Institute of Biotechology (Beijing, China); GE11 peptides and GE11-FITC were purchased from Sangong Biotech Co., Ltd. (Shanghai, China); GAPDH and EGFR Rabbit monoclonal antibody were purchased from Cell Signaling Technology (USA); secondary antibody was purchased from Proteintech (USA); Enhanced chemiluminescence (ECL) Luminescent Solution Kit was purchased from BIO-RAD (USA); cell lysate and BCA protein quantification kit were purchased from Promega (USA). Reagents without special instructions were purchased from Comeo Chemical Reagent Co., Ltd. (Tianjin, China).
Synthesis of SPION
SPION was prepared via a co-precipitation method following a previously reported protocol [Citation23]. In short, FeCl3·6H2O and FeC12·4H2O were dissolved in ultrapure water, mixed, and stirred with NHOH under nitrogen protection. The resulting black precipitate was washed with pure water to pH = 7 and then added with HNO3 and Fe(NO3)3.9H2O. After reflux for 1 h, the supernatant was magnetically separated and 4 mL ultrapure water was added. Then, the mixture was dialyzed against 0.01 mol L−1 HNO3 solution for 48 h. After freeze-drying, the black solid obtained was SPION.
Synthesis of CSO-Fe3O4 and GE11-CSO- Fe3O4
DCC (dicyclohexylcarbodiimide) (71.0 mg; 0.34 mmol) and NHS (N-hydroxysuccinimide) (39.6 mg; 0.34 mmol) were dissolved in 20 mL of DMF (N,N-dimethylformamide). After stirring, 100 μL of oleic acid was added slowly and incubated at room temperature under nitrogen for 24 h. The resulting turbid solution was filtered with a 0.25 μm organic filter membrane to remove the precipitate, and the clear solution was collected. SPION (40 mg) dispersed in 10 mL dimethyl sulfoxide (DMSO) was quickly added to the clear solution and incubated at room temperature by stirring for 48 h under nitrogen. After magnetic separation of the supernatant and freeze-drying, the O-SPION was obtained.
One gram of chitosan (viscosity <200 mPa.s) was dissolved in 100 mL of 3% acetic acid solution for 3 h. The 30 mg of oil phase iron was dissolved in 3 mL of chloroform, and then mixed with 10 mL of the above chitosan solution. After sonicating the probe for 10 min to form an emulsion, the emulsion was stirred at high speed overnight to fully volatilize the chloroform. To improve the dispersion of chitosan magnetic nanoparticles, a small amount of non-ionic surfactant (Triton) was added and stirred for 1 h. The excess chitosan was washed with sodium chloride solution and formic acid several times, and the excess sodium chloride was dialyzed. The 4 mL of CSO-Fe3O4 (30 mg) nanoparticles were mixed with 3 mg of GE11 and stirred for 0.5 h, cross-linked with 0.6 mg of EDC (1-(3-dimethylaminopropyl)-3-ethylcarbodiimide hydrochloride) for 3 h, and dialyzed overnight to obtain GE11-CSO-Fe3O4 micelles.
Characterization of GE11-CSO-SPION
The X-ray diffractometer (D/max 2200VPC RIGAKU, Japan) was used to analyze the crystal properties of the synthesized magnetic nanoparticles at room temperature, with a scanning speed of 0.02°, and a duration of 6 s. The peak shape in the XRD (X-ray diffraction) pattern was analyzed using a standard card. The morphology of synthesized CSO-SPION and GE11-CSO-SPION was evaluated by transmission electron microscopy (TEM) (JSM-100S; Electronics Co. LTD, Japan). KBr tablet method was used to measure the infrared spectra of SPION, CSO-SPION and GE11-CSO-SPION on the infrared spectrometer (Specode75; Shimadzon, Japan) using a scan range of 4000–400 cm−1. The particle size distribution and potential of SPION, CSO-SPION and GE11-CSO-SPION were evaluated by using highly sensitive Zeta potential and particle size analyzer (NanoBrook 90Plus Zeta, Brookhaven Instruments, USA) at a temperature of 25 °C and scattering angle of 90°. The magnetic hysteresis regression curve of CSO-SPION and GE11-CSO-SPION was measured with a vibrating sample magnetometer (7404, Lake Shore, USA). The magnetic field intensity range was −4000–4000 kOe.
Western blotting
Total proteins were extracted from L-O2, HepG2, SMMC-7721 cells and the protein concentration was determined with a BCA kit. Proteins were separated by 8% SDS-PAGE (sodium dodecyl sulfate polyacrylamide gel electrophoresis), and transferred to the PVDF membrane. The membrane was blocked with 5% (w/v) skimmed milk at room temperature for 2 h, and incubated with the primary antibody overnight at 4 °C (anti-EGFR, 1:800; anti-GAPH, 1:1000). The membrane was washed 3 times in TBST, and incubated with appropriate horseradish peroxidase-conjugated secondary antibodies (1:3000) for 2 h at room temperature. The membrane was then developed by using ECL and exposed to X-ray film.
Fluorescence microscope observation and flow cytometry analysis of GE11 peptide uptake
Cells were seeded in a 6-well plate (6 × 105 cells/well for HepG2 cells; 4 × 105 cells/well for L-O2 cells and SMMC-7721 cells) and incubated at 37 °C for 18–24 h. The fluorescein isothiocyanate-labeled GE11 polypeptide (FITC-GE11) (100 μg mL−1) was added to each well and incubated at 37 °C for 30 min in darkness. The serum-free medium was added to the blank control group. After washing, the cellular uptake was first observed by a fluorescence microscope (IX73; OLYMPUS, Japan) and then analyzed on a flow cytometer (BA51450; Beckman Coulter, USA).
MTT assay
The cytotoxicity of SPION, CSO-SPION and Ge11-CSO-SPION in L-O2, SMMC-7721 and HepG2 cells was detected by the MTT method. The cells were inoculated in 96-well plates at densities of 2 × 104/well (HepG2 cells) and 1 × 104/well (L-O2 cells and SMMC-7721 cells), and cultured at 37 °C for 18–22 h. SPION, CSO-SPION, GE11-CSO-SPION at concentrations of 3.125, 6.25, 12.5, 25, 50, 100 μg mL−1 were added to each well and cultured at 37 °C for 24 h. The blank control group was added with serum-free medium. Then, 20 μL MTT solution (5 mg mL−1) was added to each well and incubated in darkness. After incubation for 4 h, 150 μL DMSO was added to each well and incubated for 10 min. The optical density (OD) at 490 nm was measured with a microplate reader (1510, Thermo Fisher, USA). The cell survival rate (%) was calculated as (OD experiment/OD blank) × 100%.
Prussian blue staining
Cells were seeded in a 6-well plate at a density of 6 × 105 cells/well (HepG2 cells) and 4 × 105 cells/well (L-O2 cells), and cultured at 37 °C for 18-22 h. Then, they were incubated with CSO-SPION and GE11-CSO-SPION with 50 μg mL−1 iron concentration for 1 h. After washing, cells were fixed with paraformaldehyde for 30 min, and stained according to the Prussian blue kit.
In vitro MRI
L-O2, SMMC-7721 cells and HepG2 cells were inoculated in a 6-well plate at a density of 4 × 105 cells/well and 6 × 105 cells/well, respectively, and cultured for 18-24 h. After aspirating the medium, CSO-SPION and GE11-CSO-SPION solution (0, 6.25, 12.5, 25, 50, 100 μg mL−1) were added to each well. After incubation for 1 h, cells were collected and mixed with 1.8% agarose. A 3.0 T MR scanner (MAGNETOM Skyra, SIEMENS, Germany) was used to analyze the sample (TR = 3700 ms, TE = 117 ms, FOV = 12 × 12).
Animals
SPF BALB/c nude mice (n = 20), 5–6 weeks old, weighing 15–18 g, were purchased from Beijing Vital River Laboratory Animal Technology Co. Ltd. (license number: SCXK (Beijing) 2016-0006). They were housed in independent ventilated cages (with 5 mice per case), at room temperature of 23–25 °C and humidity of 50%–60%, and with water and standard chew ad libitum.
Ethics statement
All animal experiments were performed strictly in accordance with Yanbian University Animals Center management practices for breeding. This study was approved by the Ethics Committee of Yanbian University. All care was taken to minimize animal suffering.
In vivo MRI
A 0.2 mL mixture of 1 × 108 HepG2 cells with Matrigel (1:1) was subcutaneously injected into the right axilla of Balb/c nude mice. When the volume of the tumor reached 150 mm3, all mice were randomly divided into the CSO-SPION group and GE11-CSO-SPION group. Before the MRI scan, CSO-SPION and GE11-CSO-SPION were injected into the tail vein. The injection amount was equivalent to the iron concentration of 15 mg kg−1. MRI scans (wrist coil Scan and line conventional T2WI scan) were performed 4 h after injection using German Siemens 3.0 T MRI scanner (MAGNETOM Skyra, SIEMENS, Germany) under anesthesia by intraperitoneal injection of 20% urethane (1 mg kg−1). The specific parameters of T2WI were: TSE sequence, TE 116 ms, TR3100, inversion angle 89 degrees, layer thickness 2 mm, matrix 256 × 1256, and voxel 0.3 × 0.3 × 0.9 (mm). The T2WI horizontal axis image was obtained. The tissue signal intensity of the tumor area was measured before and after the administration of agents, the 20 mm2 circular cursor was used as the region of interest. The largest level of the tumor tissue was selected and measured 3 times, and an average value was obtained and recorded.
Histology analysis
Twelve hours after the injection, the nude mice were sacrificed by cervical dislocation. The tumor tissues were collected. The liver, spleen and kidney were embedded in paraffin, sectioned (thickness: 5 μm), routinely dewaxed and hydrated, and analyzed with Hematoxylin-Eosin staining and Prussian blue staining.
Statistical analysis
SPSS 16.0 (International Business Machines Corporation, USA) was used for statistical analysis. Data were presented as means ± SEM, and comparisons were made using Student’s t-test. p < 0.05 (two-sided) was considered statistically significant.
Results
Characterization of SPION, CSO-SPION and GE11-CSO-SPION
As shown in , SPION’s X-ray diffraction pattern had six characteristic peaks (2θ = 30.1°, 35.4°, 43.1°, 56.9°, 65.7°, 74.9°) corresponding to (220), (311), (400), (333), (531), (622) crystal planes of cubic phase Fe3O4, respectively. After comparing with the standard card 01-074-0748, it was confirmed that the magnetic substance was pure Fe3O4.
Figure 1. Characterization of SPION, CSO-SPION and GE11-CSO-SPION. (A) XRD pattern of SPION. (B) TEM image of CSO-SPION and GE11-CSO-SPION. (C) FT-IR images of SPION, CSO-SPION and GE11-CSO-SPION. (D) Size distribution study of SPION, CSO-SPION and GE11-CSO-SPION. (E) Zeta potential of SPION, CSO-SPION and GE11-CSO-SPION. (F) Magnetic hysteresis loops of CSO-SPION and GE11-CSO-SPION.
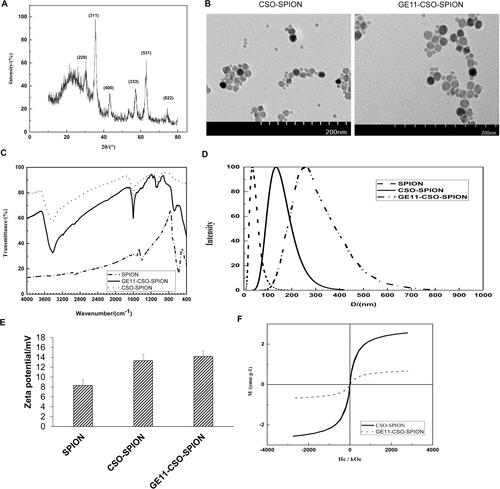
TEM images of CSO-SPION and GE11-CSO-SPION showed that the particle size was uniform and there was no obvious aggregation (). showed that the carbonyl peak of OA was near 1708 cm−1. The peaks near 2854 cm−1 and 2924 cm−1 represented the methylene of OA. The Fe-O characteristic peak of Fe3O4 nanoparticles was near 578 cm−1 n. Weak characteristic peaks of OA @Fe3O4 appeared in the CS-modified OA-Fe3O4 spectrum. The difference between OA-Fe3O4 and the blend was the hydroxyl peak near 3414 cm−1, while 1051 cm−1 and 1081 cm−1, 1156 cm−1 was the COC on the glycosidic bond, 1593 cm−1 was the -NH2 on the amino group, 1401 cm−1 was the hydroxyl group on the ring, and 1235 cm−1 was the CO group. After coupling with GE11, the peak at 665 cm−1 was significantly weakened. In summary, GE11-CSO-SPION was successfully synthesized.
As shown in , SPION hydrated particle size was 35.60 ± 1.51 nm and PDI was 0.19; CSO-SPION hydrated particle size was 135.27 ± 3.21 nm and PDI was 0.19; GE11-CSO-SPION hydrated particle size was 295.41 ± 12.56 nm and PDI was 0.20.
The Zeta potentials of SPION, CSO-SPION and GE11-CSO-SPION were all positive: + (8.32 ± 1.12) mV, + (13.32 ± 1.25) mV and + (14.18 ± 1.11) mV, respectively (). showed that both CSO-SPION and GE11-CSO-SPION had superparamagnetism without residual magnetization and hysteresis. The saturation magnetic value of CSO-SPION was 2.563 emu g−1. After the GE11 peptide was bound, the saturation magnetization was slightly decreased. The saturation magnetization value of GE11-CSO-SPION was 0.677 emu g−1, which is still higher than other reported imaging saturation magnetization values [Citation24–26].
Expression level of EGFR in L-O2, HepG2 and SMMC-7721 cells
Western blot was used to detect EGFR expression in L-O2, HepG2 and SMMC-7721 cells. There was no EGFR protein expression in L-O2 cells, whereas the EGFR protein expression was observed in HepG2 and SMMC-7721 cells (). Moreover, the level of EGFR protein expression on HepG2 cells was higher than that on SMMC-7721 cells.
Cellular uptake of GE11 peptide
To determine the cellular uptake of GE11 peptide, we first observed the fluorescence of GE11. As shown in , there was FITC fluorescence in the HepG2 and SMMC-7721 cells, indicating the binding of FITC-GE11 polypeptide to the EGFR protein on the cell surface. There was no obvious green fluorescence in the L-O2 cells. Then, we analyzed the binding of GE11 peptide to EGFR by flow cytometry. Similarly, the fluorescence labeling curve shifted to the right in the HepG2 and SMMC-7721 cells, but not in the L-O2 cells (). Statistically, the uptake rates of GE11 peptides by L-O2, HepG2 and SMMC-7721 cells were 0.15%, 16.19% and 6.63%, respectively. Compared with the L-O2 cell group, there was a significant difference in both HepG2 and SMMC-7721 cells (both p < 0.05) (). These results suggest that GE11 polypeptide has a high affinity for cells with a high expression of EGFR.
Figure 3. Fluorescence analysis of in vitro cellular uptake of FITC-GE11 in different cell lines. (A) Images of L-O2, HepG2 and SMMC-7721 cells incubated with FITC-GE11 respectively. 200X magnification; 50 μm scale. (B) Flow cytometry data of fluorescence in L-O2, HepG2 and SMMC-7721 cells after incubation using 100 μg mL−1 FITC-GE11 for 30 min. (C) Fluorescence intensity of FITC in L-O2, SMMC-7721 and HepG2 cells. (*p < 0.05).
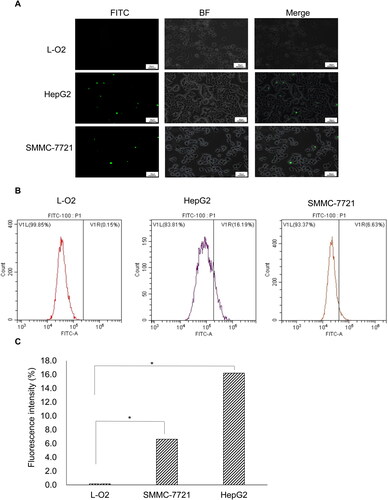
In vitro cytotoxicity
MTT assay was used to measure the cytotoxicity of SPION, CSO-SPION and GE11-CSO-SPION. When the iron concentrations of SPION, CSO-SPION and GE11-CSO-SPION were increased to 100 μg mL−1, the survival rates of L-O2, SMMC-7721 and HepG2 cells were greater than 80%, indicating that the prepared nanomaterials had no obvious cytotoxicity in these cell lines ().
In vitro Prussian blue staining
The uptake of micelles was evaluated in vitro by Prussian Blue Staining. As shown in , uptake of GE11-CSO-SPION micelles was more than CSO-SPION micelles in HepG2 cells at the same iron concentration. Moreover, HepG2 cells could uptake more GE11-CSO-SPION micelles than L-O2 cells under the same GE11-CSO-SPION micellar culture (). These results indicate that GE11 peptide-labeled CSO-SPION can specifically bind to EGFR on the surface of HepG2 cells.
Figure 5. Cellular uptake of CSO-SPION and GE11-CSO-SPION micelles. Prussian blue staining images of micelles in HepG2 cells after incubation with CSO-SPION (A) and GE11-CSO-SPION (B) (50 μg mL−1) for 1 h. Prussian blue staining images of micelles in L-O2 (C) and HepG2 cells (D) after incubation with GE11-CSO-SPION (50 μg mL−1) for 1 h. 200X magnification; 50 μm scale.
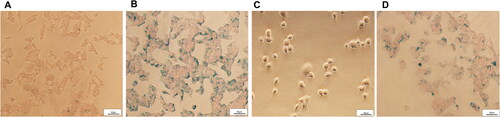
In vitro MRI
To determine the potential of GE11-CSO-SPION as an MRI magnetic resonance contrast agent, in vitro MRI was performed. With the increase of iron concentration, the T2 signal intensity of L-O2, SMMC-7721 and HepG2 cells on MRI gradually decreased (). When the concentration of GE11-CSO-SPION micelles was 50 μg mL−1, the T2 signal intensity of HepG2 cells was consistent with the background signal and could not be distinguished (), indicating that the GE11 polypeptide could bind to the EGFR protein on the cell surface to enhance the uptake of GE11-CSO-SPION micelles.
Figure 6. In vitro MRI study of CSO-SPION and GE11-CSO-SPION micelles. (A) T2-weighted MRI images of Ge11-CSO-SPION micelles with different iron concentrations in L-O2, SMMC-7721 and HepG2 cells. (B) T2-weighted MRI images of GE11-CSO-SPION micelles with different iron concentrations in HepG2 cells.
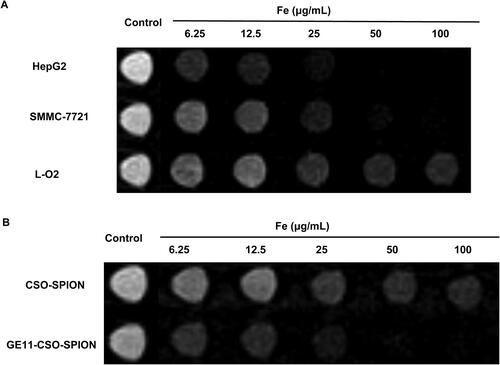
In vivo MRI
In vivo MRI of tumor-bearing nude mice showed that the signal value of the tumor area was not significantly decreased at 4 h after injection of CSO-SPION (). However, the significantly decreased signal value of the tumor area was observed following injection of GE11-CSO-SPION 4 h (p < 0.05, ). The change of signal intensity showed that GE11-CSO-SPION micelles had excellent T2 imaging performance. Therefore, we confirmed that targeting using GE11 peptide could effectively improve the efficiency of MRI.
In vivo distribution of SPION micelles
HE staining showed that there was no obvious difference in liver, spleen and kidney between groups (), indicating that GE11-CSO-SPION and CSO-SPION were non-toxic. The results of Prussian blue staining showed obvious blue-stained particles in the liver and spleen but not in the kidneys (). More blue-stained particles could be seen in the tumor tissue of the GE11-CSO-SPION injection group.
Discussion
EGFR is composed of four protein tyrosine kinases that play an important role in tumor proliferation, namely EGFR (HER1), HER2, HER3 and HER4. EGFR is involved in the activation of a series of signal pathways, which can further promote cell proliferation, angiogenesis, metastatic spread and apoptosis inhibition [Citation27]. It has been shown that EGFR is overexpressed in HCC but not in normal liver tissues [Citation28–30]. In this study, we also found that the expression of EGFR on the cell membrane of liver cancer cells (SMMC-7721 and HepG2) was up-regulated compared with normal human liver cells (L-O2). The CSO-SPION modified by chitosan has better biocompatibility without changing its super paramagnetic properties [Citation31, Citation32]. In this study, the CSO-SPION micelles were modified by targeting EGFR, and the contrast agent GE11-CSO-SPION specifically targeting SMMC-7721 and HepG2 cell lines was prepared and evaluated by electron microscope, dynamic light scattering (DLS), Zeta potential and Fourier infrared spectrometer. The hydrated particle size of the micelles modified by GE11 was 295.41 ± 12.56 nm; PDI was 0.2; and Zeta potential was + (14.18 ± 1.11) mV. These results indicate that the GE11 polypeptide was successfully modified to the surface of CSO-SPION, which has uniform size, stable structure, no obvious aggregation, and still has the crystal structure of ferroferric oxide. The particle size of the micelle measured by DLS was slightly larger than that measured by TEM. The difference between the DLS and TEM may be caused by the swelling of the peptides in the micelles in the aqueous solution and the collapse under dry conditions [Citation33]. Furthermore, when L-O2 cells that did not express EGFR were incubated with GE11-FITC for 30 min, the L-O2 cells did not show an observable green signal due to the lack of EGFR on the cells. All these results confirmed the targeted recognition and binding ability of GE11 peptide to EGFR high-expressing cells.
The in vitro cytotoxicity test verified that SPION, CSO-SPION and GE11-CSO-SPION were low-toxic. Nanoparticles can accumulate in cells through active and passive means. It has been confirmed that the amount of nanoparticles entering tumor cells through receptor-mediated active targeting is much higher than the amount of nanoparticles entering cells through EPR effect passive targeting [Citation33–35].
Prussian blue staining can stain trivalent iron blue, which can directly show the uptake of micelles by cells. In this study, we found that blue dye particles in HepG2 cells cultured in micellar medium containing GE11-CSO-SPION were significantly increased compared with the control group. The deposition of trivalent iron in cells provides potential for the application of multifunctional nanoparticles in molecular imaging and drug delivery [Citation36].
In vitro MRI showed the targeting ability and imaging effect of GE11 peptide as a targeting peptide. In vivo MRI proved that the GE11-CSO-SPION group had a higher MR contrast enhancement effect than the GE11 peptide group 4 h after injection. And there was no bleeding at the tumor site in the tissue section, indicating that the signal reduction of the tumor tissue was caused by the accumulation of SPION at the tumor site. This may be due to the synergy of GE11-CSO-SPION through EPR and targeting-mediated endocytosis. The effect makes it accumulate faster and in greater quantity at the tumor site. Prussian blue staining of tissue sections also showed that GE11-CSO-SPION existed for a longer time at the tumor site than CSO-SPION. This may be because the GE11 peptide could increase the residence time of micelles in the tumor tissue.
Prussian blue staining of tumor tissues also confirmed that a large number of blue-stained particles were still visible at 12 h after the injection of GE11-CSO-SPION micelles. The specific targeting of GE11-CSO-SPION micelles to EGFR high-expressing cells leads to enhanced uptake of tumor tissues, showing that GE11-CSO-SPION micelles can serve as MR T2 negative contrast agents. Prussian blue staining of the liver and spleen showed more blue-stained particles, indicating that GE11-CSO-SPION micelles are mainly metabolized though the liver and spleen. This is similar to the previously reported results [Citation35, Citation37, Citation38], and may be related to the clearance mechanism of the reticuloendothelial system.
This study has several limitations. Firstly, the short time imaging effect (less than 4 h) of GE11-CSO-SPION was not evaluated. Secondly, the MRI effect of tumors in situ was not determined. Further studies are needed.
Conclusions
We successfully synthesized GE11-CSO-SPION micelles for MR negative contrast agents. The results of in vitro and in vivo experiments showed that GE11-CSO-SPION had better specific targeting and higher MRI sensitivity to target HCC with high EGFR expression, and could reduce the dose required for MRI and extend the residence time at the tumor site. The results of this study demonstrate that these micelles may provide ideas for noninvasive molecular MRI diagnosis of HCC with high EGFR expression in the future.
Abbreviations | ||
HCC | = | Hepatocellular cancer |
SPION: | = | superparamagnetic iron oxide nanoparticle |
EPR: | = | enhanced permeability and retention |
FDA: | = | Food and Drug Administration |
CSO: | = | chitosan oligosaccharide |
EGFR: | = | epidermal growth factor receptor |
SDS-PAGE: | = | sodium dodecyl sulfate polyacrylamide gel electrophoresis |
MTT: | = | 3-(4,5-Dimethylthiazol-2-yl)-2,5-diphenyltetrazolium bromide |
DCC: | = | dicyclohexylcarbodiimide |
NHS: | = | N-Hydroxysuccinimide |
DMF: | = | N,N-dimethylformamide |
EDC: | = | 1-(3-Dimethylaminopropyl)-3-ethylcarbodiimide hydrochloride |
XPD: | = | X-ray diffraction |
TEM: | = | transmission electron microscopy |
ECL: | = | enhanced chemiluminescence |
FITC-GE11: | = | Fluorescein isothiocyanate-labeled GE11 polypeptide |
DMSO: | = | dimethyl sulfoxide |
OD: | = | optical density |
DLS: | = | dynamic light scattering |
Data availability statement
Data are available from the corresponding author upon reasonable request.
Disclosure statement
No potential conflict of interest was reported by the authors.
Additional information
Funding
References
- Sia D, Villanueva A, Friedman SL, et al. Liver cancer cell of origin, molecular class, and effects on patient prognosis. Gastroenterology. 2017;152(4):745–761.
- Bray F, Ferlay J, Soerjomataram I, et al. Global cancer statistics 2018: GLOBOCAN estimates of incidence and mortality worldwide for 36 cancers in 185 countries. CA Cancer J Clin. 2018;68(6):394–424.
- Zheng XC, Ren W, Zhang S, et al. The theranostic efficiency of tumor-specific, pH-responsive, peptide-modified, liposome-containing paclitaxel and superparamagnetic iron oxide nanoparticles. Int J Nanomed. 2018;13:1495–1504. PubMed PMID: 29559778; PubMed Central PMCID: PMC5856286.
- Wen L, Yang S, Zhong J, et al. Thermoacoustic imaging and therapy guidance based on ultra-short pulsed microwave pumped thermoelastic effect induced with superparamagnetic iron oxide nanoparticles. Theranostics. 2017;7(7):1976–1989. PubMed PMID: 28638483; PubMed Central PMCID: PMC5479284.
- Shen X, Li T, Chen Z, et al. Luminescent/magnetic PLGA-based hybrid nanocomposites: a smart nanocarrier system for targeted codelivery and dual-modality imaging in cancer theranostics. Int J Nanomed. 2017;12:4299–4322. PubMed PMID: 28652734; PubMed Central PMCID: PMC5473604.
- Singh N, Jenkins GJ, Asadi R, et al. Potential toxicity of superparamagnetic iron oxide nanoparticles (SPION). Nano Rev. 2010;1(1):5358. PMID: 22110864; PubMed Central PMCID: PMC3215220.
- Huang G, Li H, Chen J, et al. Tunable T1 and T2 contrast abilities of manganese-engineered iron oxide nanoparticles through size control. Nanoscale. 2014;6(17):10404–10412.
- Gao Z, Ma T, Zhao E, et al. Small is smarter: nano MRI contrast agents – advantages and recent achievements. Small. 2016;12(5):556–576.
- Zhou Z, Wu C, Liu H, et al. Surface and interfacial engineering of iron oxide nanoplates for highly efficient magnetic resonance angiography. ACS Nano. 2015;9(3):3012–3022.
- Tang S, Du Q, Liu T, et al. In vivo magnetic resonance imaging and microwave thermotherapy of cancer using novel chitosan microcapsules. Nanoscale Res Lett. 2016;11(1):334. PubMed PMID: 27422776; PubMed Central PMCID: PMC4947076.
- Maeda H, Tsukigawa K, Fang J. A retrospective 30 years after discovery of the enhanced permeability and retention effect of solid tumors: next-generation chemotherapeutics and photodynamic therapy-problems, solutions, and prospects. Microcirculation. 2016;23(3):173–182.
- Xiao Y, Lin ZT, Chen Y, et al. High molecular weight chitosan derivative polymeric micelles encapsulating superparamagnetic iron oxide for tumor-targeted magnetic resonance imaging. Int J Nanomed. 2015;10:1155–1172. PubMed PMID: 25709439; PubMed Central PMCID: PMC4330038.
- Gholami L, Tafaghodi M, Abbasi B, et al. Preparation of superparamagnetic iron oxide/doxorubicin loaded chitosan nanoparticles as a promising glioblastoma theranostic tool. J Cell Physiol. 2019;234(2):1547–1559.
- Chi YH, Hsiao JK, Lin MH, et al. Lung cancer-targeting peptides with multi-subtype indication for combinational drug delivery and molecular imaging. Theranostics. 2017;7(6):1612–1632. PubMed PMID: 28529640; PubMed Central PMCID: PMCPmc5436516. eng.
- D’Aguanno S, Del Bufalo D. Inhibition of anti-apoptotic Bcl-2 proteins in preclinical and clinical studies: current overview in cancer. Cells. 2020;9(5):1287. PubMed PMID: 32455818; PubMed Central PMCID: PMC7291206.
- Oerlemans C, Bult W, Bos M, et al. Polymeric micelles in anticancer therapy: targeting, imaging and triggered release. Pharm Res. 2010;27(12):2569–2589. PubMed PMID: 20725771; PubMed Central PMCID: PMC2982955.
- Yang L, Mao H, Cao Z, et al. Molecular imaging of pancreatic cancer in an animal model using targeted multifunctional nanoparticles. Gastroenterology. 2009;136(5):1514–1525.e2. PubMed PMID: 19208341; PubMed Central PMCID: PMCPmc3651919. eng.
- Scaltriti M, Baselga J. The epidermal growth factor receptor pathway: a model for targeted therapy. Clin Cancer Res. 2006;12(18):5268–5272.
- Garousi J, Andersson KG, Mitran B, et al. PET imaging of epidermal growth factor receptor expression in tumours using 89Zr-labelled ZEGFR:2377 affibody molecules. Int J Oncol. 2016;48(4):1325–1332. PubMed PMID: 26847636; PubMed Central PMCID: PMC4777594.
- Komposch K, Sibilia M. EGFR signaling in liver diseases. IJMS. 2015;17(1):30. PubMed PMID: 26729094; PubMed Central PMCID: PMC4730276.
- Zhou C, Xia Y, Wei Y, et al. GE11 peptide-installed chimaeric polymersomes tailor-made for high-efficiency EGFR-targeted protein therapy of orthotopic hepatocellular carcinoma. Acta Biomater. 2020;113:512–521.
- Chang L, Wang G, Jia T, et al. Armored long non-coding RNA MEG3 targeting EGFR based on recombinant MS2 bacteriophage virus-like particles against hepatocellular carcinoma. Oncotarget. 2016;7(17):23988–24004. PubMed PMID: 26992211; PubMed Central PMCID: PMC5029679.
- Mickler FM, Mockl L, Ruthardt N, et al. Tuning nanoparticle uptake: live-cell imaging reveals two distinct endocytosis mechanisms mediated by natural and artificial EGFR targeting ligand. Nano Lett. 2012;12(7):3417–3423.
- Ling D, Hyeon T. Chemical design of biocompatible iron oxide nanoparticles for medical applications. Small. 2013;9(9–10):1450–1466.
- Saesoo S, Sathornsumetee S, Anekwiang P, et al. Characterization of liposome-containing SPIONs conjugated with anti-CD20 developed as a novel theranostic agent for central nervous system lymphoma. Colloids Surf B Biointerfaces. 2018;161:497–507.
- Tom G, Philip S, Isaac R, et al. Preparation of an efficient and safe polymeric-magnetic nanoparticle delivery system for sorafenib in hepatocellular carcinoma. Life Sci. 2018;206:10–21.
- Baxi SM, Tan W, Murphy ST, et al. Targeting 3-phosphoinoside-dependent kinase-1 to inhibit insulin-like growth factor-I induced AKT and p70 S6 kinase activation in breast cancer cells. PloS One. 2012;7(10):e48402. PubMed PMID: 23119004; PubMed Central PMCID: PMC3485233.
- Huan H, Wen X, Chen X, et al. C/EBPα short-activating RNA suppresses metastasis of hepatocellular carcinoma through inhibiting EGFR/β-Catenin signaling mediated EMT. PloS One. 2016;11(4):e0153117. PubMed PMID: 27050434; PubMed Central PMCID: PMCPmc4822802. eng.
- Guo Y, Wu Z, Shen S, et al. Nanomedicines reveal how PBOV1 promotes hepatocellular carcinoma for effective gene therapy. Nat Commun. 2018;9(1):3430.
- Liu X, Tian S, Liu M, et al. Wogonin inhibits the proliferation and invasion, and induces the apoptosis of HepG2 and Bel7402 HCC cells through NF‑κB/Bcl-2, EGFR and EGFR downstream ERK/AKT signaling . Int J Mol Med. 2016;38(4):1250–1256.
- Fan Y, Huang Y. The effective peroxidase-like activity of chitosan-functionalized CoFe2O4 nanoparticles for chemiluminescence sensing of hydrogen peroxide and glucose. Analyst. 2012;137(5):1225–1231.
- Fernandez-Lucas J, Harris R, Mata-Casar I, et al. Magnetic chitosan beads for covalent immobilization of nucleoside 2’-deoxyribosyltransferase: application in nucleoside analogues synthesis. J Ind Microbiol Biotechnol. 2013;40(9):955–966.
- Jiang J, Li J, Zhou B, et al. Fabrication of polymer micelles with zwitterionic shell and biodegradable core for reductively responsive release of doxorubicin. Polymers. 2019;11(6):1019. PubMed PMID: 31181866; PubMed Central PMCID: PMC6631697.
- Situ JQ, Wang XJ, Zhu XL, et al. Multifunctional SPIO/DOX-loaded A54 homing peptide functionalized dextran-g-PLGA micelles for tumor therapy and MR imaging. Sci Rep. 2016;6:35910. PubMed PMID: 27775017; PubMed Central PMCID: PMC5075939.
- Wang Z, Qiao R, Tang N, et al. Active targeting theranostic iron oxide nanoparticles for MRI and magnetic resonance-guided focused ultrasound ablation of lung cancer. Biomaterials. 2017;127:25–35. PubMed PMID: 28279919; PubMed Central PMCID: PMC5400286.
- Jain TK, Morales MA, Sahoo SK, et al. Iron oxide nanoparticles for sustained delivery of anticancer agents. Mol Pharm. 2005;2(3):194–205.
- Liu Y, Huang W, Xiong C, et al. Biodistribution and sensitive tracking of immune cells with plasmonic gold nanostars. Int J Nanomed. 2019;14:3403–3411. PubMed PMID: 31190799; PubMed Central PMCID: PMC6514259.
- Chen X, Zhou H, Li X, et al. Plectin-1 targeted dual-modality nanoparticles for pancreatic cancer imaging. EBioMedicine. 2018;30:129–137. PubMed PMID: 29574092; PubMed Central PMCID: PMC5952251.