Abstract
Callose plays an important role in plant development and response to multiple biotic and abiotic stresses. Callose synthase (CalS; EC 2.4.1.34), the main enzyme in callose synthesis, has been studied in a number of crops but not in cucumber (Cucumis sativus L.). In this study, 10 genes encoding putative callose synthase were identified and classified into four clades. Expression levels of the 10 CsCalS genes, which varied considerably, were detected in most analyzed organs. Transcription of CsCalS1 and CsCalS8 was obviously induced by downy or powdery mildews, which are pathogenic fungi of cucumber. CsCalS4, which was highly expressed in male flowers, was chosen for further study. Expression analysis revealed that CsCalS4 was mainly expressed in anther, and highly expressed at the stage of anther meiosis (St10), and uninuclear pollen appearance stage (St11). Histochemical and subcellular localization analyses indicated that CsCalS4 was mainly present in pollen grains and primarily localized in the plasma membrane. The results of this study provided a basic understanding of CsCalS genes and suggest the involvement of CsCalS4 in pollen development.
Introduction
Callose, a β-1,3-glucan polysaccharide with several β-1,6-branches, surrounds pollen mother cells, cell walls, cell plates and sieve plates in a wide variety of higher plants [Citation1,Citation2]. Callose is important in plant development and responses to biotic and abiotic stresses [Citation3]. According to current consensus, callose is produced by callose synthase (CalS; EC 2.4.1.34; UDP-glucosyl transferase), which uses UDP-glucose as a substrate. CalS genes have been isolated from many crops, such as Arabidopsis thaliana [Citation4], barley (Hordeum vulgare) [Citation5], rapeseed (Brassica napus) [Citation6], pepper (Capsicum annuum) [Citation7] and maize (Zea mays) [Citation8]. Genes encoding putative callose synthases in the model plant A. thaliana have been designated as CalS [Citation4] or GSL (glucan synthase-like) [Citation9]. In this paper, we use the CalS nomenclatural system. In plants, callose synthase is usually encoded by a relatively large family of genes. The encoded amino acids comprise approximately 2000 residues and have a molecular weight of approximately 200 kDa, which is larger than the products of most plant genes [Citation10–12].
Callose synthase is involved in various biological processes associated with plant growth, development and stress responses. First, CalS is necessary for the normal structure and normal functioning of the material transport system. As mentioned above, 12 AtCalS genes have been identified and characterized in Arabidopsis [Citation9]. AtCalS7 (AtGSL7) participates in sieve plate pore formation and directly affects the transport function of phloem [Citation13,Citation14]. In addition, almost all cells are connected by plasmodesmata, which controls the transport of nutrients, signalling molecules and so on [Citation1]. Compared with the wild type, the Arabidopsis mutant AtCalS10 (gsl8/chorus) showed reduced callose deposition in the plasmodesmata and cell plate, indicating that AtCalS10 (GSL8) was required for cytokinesis and cell patterning [Citation15–17]. Second, CalS participates in pollen development. Mutational and correlative analyses have suggested or proven that AtCalS5, AtCalS9, AtCalS10, AtCalS11 and AtCalS12 encode isoforms with important functions in pollen development [Citation18–21]. Although all five of these genes participate in pollen development, their functions in this process are different. AtCalS5 plays a major role in callose wall synthesis during pollen formation and participates in callose barrier and pollen tube wall formation during pollen tube elongation [Citation19,Citation22]. AtCalS9 is required for symmetric and asymmetric mitosis during gamete formation [Citation20,Citation21,Citation23]. AtCals10 is directly involved in the initial positioning of cell plates in asymmetric mitosis during pollen microgamete formation and directly affects stomatal formation in plant leaves [Citation17,Citation20]. AtCals11 and AtCals12 play a crucial role in callose plate synthesis during microspore tetrad formation [Citation18]. The third, CalS plays an important role in the response to stress. Callose deposition occurs within minutes of damage, as well as during physiological or biotic stress. CalS12 (GSL5) reportedly accounts for callose synthesis in sporophytic tissues during injury or pathogen invasion [Citation24–26]. The gsl5 mutant was unable to synthesize callose at papillae and made plants more resistant to pathogens. It suggested that callose deficiency in gsl5 mutants may enhance SA signalling, thereby enhancing resistance to pathogens [Citation24].
Cucumber is one of the world’s most widely cultivated and consumed vegetable crops. Nevertheless, no reports have appeared concerning CalS homologs in cucurbitaceous crops. In this study, we identified and isolated CalS family members, analyzed their expression levels and predicted their functions, with a special focus on CsCalS4. Our findings could aid future studies on cucumber growth, development and stress resistance.
Materials and methods
Plant material
Seeds of wild-type cucumber (Cucumis sativus ‘Xintaimici’) were sown in 20 × 20 cm plastic pots containing vermiculite and then incubated at 25/18 °C (day/night) under a 16-h/8-h light/dark regime in a phytotron. The length of floral bud served as a criterion to define the developmental stages of cucumber male flowers, as described by Sun et al. [Citation27].
Arabidopsis ecotype Col-0 was grown in potting soil in a growth chamber under short-day (8-h light/16-h dark) or long-day (16-h light/8-h dark) conditions at 22 °C and 55% relative humidity. Arabidopsis transformation was performed with Agrobacterium tumefaciens strain GV3101 [Citation28] using the floral dip method [Citation29].
Identification of CalS sequences
CalS family genes of cucumber were identified by BLASTP searching against the Arabidopsis genome (https://www.arabidopsis.org). Sequences of the different CsCalS genes were obtained from the Cucurbit Genomics databases (http://cucurbitgenomics.org/). Synteny analyses between cucumber and Arabidopsis CalS sequences were based on identity percentages returned using BLASTN (https://blast.ncbi.nlm.nih.gov/). The resulting 10 putative cucumber CalS genes were named CsCalS1–10.
The complete deduced amino acid sequences of CalS genes from cucumber and Arabidopsis were used to construct a phylogenetic tree. The sequences were subjected to multiple alignment in CLUSTAL W and analyzed by the neighbor-joining method in MEGA v6.0 [Citation30].
Gene structure and transmembrane domain (TMD) analysis
Information on CsCalS genes, including locus tags and accession numbers, were acquired from the Cucurbit Genomics databases and NCBI (National Center for Biotechnology Information). The exon-intron structure was analyzed using the GSDS2.0 website (http://gsds.cbi.pku.edu.cn/). Protein sequence analysis was performed using the SMART website (http://smart.embl-heidelberg.de/), and the biochemical properties were predicted based on the amino acid sequence with the ProtParam online software (http://web.expasy.org/protparam/). Functional domain analysis was carried out using the Prosite database (https://prosite.expasy.org/). TMDs were analyzed using TMHMM (http://www.cbs.dtu.dk/services/TMHMM/).
Preparation and inoculation of fungal pathogen spore suspension from cucumber downy and powdery mildews
Fresh sporangia were collected and brushed into Petri dishes filled with sterile distilled water. After three rounds of filtration, a spore suspension was prepared. Suspended droplets were placed on a haemocytometer, and the concentration of spores in the suspension was determined under a 40× microscope. The concentrations of downy mildew and powdery mildew spores were 5 × 105 spores/mL and 1 × 105 spores/mL, respectively.
Six cucumber seedlings (at the six-leaf stage) with the same growth status were chosen as test plants, with four leaves per plant selected for inoculation and numbering. The front and back sides of each leaf were spray-inoculated with 2 mL of cucumber downy mildew/powdery mildew spore suspension at 17:00. Water was used as a control. The inoculated cucumber leaves were quickly covered with a self-sealing bag. Samples of treated and control plants were taken 6 and 24 h after inoculation. All samples were rinsed and immediately frozen in liquid nitrogen before storage at −80 °C.
RNA extraction and cDNA synthesis
Total RNA was extracted from the cucumber samples using a Column Plant RNAout kit (Tiandz, Beijing, China; http://www.tiandz.com). The extracted total RNA was treated with DNAase to remove residual DNA. Approximately 3 μg of purified RNA was used as a template for single-stranded cDNA synthesis using PowerScript reverse transcriptase (Invitrogen, Carlsbad, CA, USA).
Real-time quantitative polymerase chain reaction (qRT-PCR)
qRT-PCR was performed on an ABI 7500 system (Bio-Rad, United States) using the SYBR Green detection protocol (Takara). TUA was used as an internal control. Primers used for the qRT-PCR analysis are given in . The mean expression level of relevant genes was calculated using the 2−ΔΔCt method [Citation31].
Table 1. Information of CsCalS genes.
Construction of a β-glucuronidase (GUS) vector and GUS staining
To generate a CsCalS4 promoter::GUS construct, a 1.8-kb fragment of the CsCalS4 promoter (−1860 to −1 bp from the ATG start codon) was amplified from genomic DNA using primers PCsCalS4F and PCsCalS4F () and then inserted between the PstI and BamHI sites of a pCAMBIA1391 vector.
For GUS staining, the transgenic Arabidopsis samples were incubated in GUS staining solution overnight at 37 °C using the method described by Jefferson et al. [Citation32]. After staining, the samples were washed with 80% ethanol and observed.
Localization of CsCalS4::GFP in epidermal cells of onion (Allium cepa)
To study the subcellular localization of the CsCalS4 protein, the 1254-bp coding sequence of CsCalS4 (4504–5757 bp from the ATG start codon, including eight C-terminal TMDs) was amplified from cDNA using primers GCalS4F and GCalS4R (). The resulting PCR product was then fused to the upstream region of green fluorescent protein (GFP) between the SmaI (5′-end)/BamHI (3′-end) sites of a pEZS-NL vector (http://deepgreen.stanford.edu).
Using a Bio-Rad PDS-1000/He particle delivery system, strips of onion bulb epidermis were bombarded with gold particles containing the CsCalS4::GFP fusion plasmid. The epidermal strips were placed on Murashige-Skoog medium and incubated in darkness at 22 °C for 24 h. GFP fluorescence was then observed under a confocal laser-scanning microscope (Nikon C1, Tokyo, Japan) at a 488-nm excitation wavelength.
Results
Identification and phylogenetic analysis of the cucumber CalS family
Ten candidate CsCalS genes were identified and named CsCalS1-10 (). The CsCalS sequences were based on BLAST searches against NCBI and Cucurbit Genomics databases (http://cucurbitgenomics.org/). The deduced CsCalS polypeptides ranged from 1767 to 1951 amino acid residues. Eight of the deduced cucumber polypeptides (CsCalS1-8) had an average size of 1930 amino acid residues, whereas two polypeptides (CsCalS9-10) averaged 1768 residues, which was approximately 160 amino acids shorter than the first eight polypeptides (). Their isoelectric points (pI) were from 8.36 to 9.12, indicating that they were alkaline proteins.
According to gene structural modelling, most CsCalS genes have 40–50 exons; the exceptions are CsCalS9 and CsCalS10, with only one exon each (). To analyze the structural characteristics of CsCalS genes, we examined their conserved regions and aligned their putative protein sequences. According to our results, CsCalS proteins have pfam14288 (FKS1_dom1;1,3-beta-glucan synthase subunit FKS1, domain-1) and pfam02364 (Glucan_synthase; 1,3-beta-glucan synthase component) domains. In addition, we found that CsCalS1-6, but not CsCalS7-10, have a pfam04652 (Vta1) domain.
Figure 1. Gene sequence and transmembrane analysis of CsCalS. (A) Exon-intron structure of the 10 CsCalS genes. (B) Transmembrane domains of CsCalS proteins. The positions of the N-terminal and C-terminal domains of each protein are indicated by blue or pink lines. The domains were predicted using the TMHMM server.

All CsCalSs appeared to be membrane proteins with 13–18 TMDs (). To confirm the presence of the TMDs, the amino acid sequences were submitted to the TMHMM v.2.07 server. All CsCalS proteins contain multiple TMDs clustered into two regions (N-terminal and C-terminal), leaving a large hydrophilic central loop. The N-terminal TMDs are outside the membrane in CsCalS1, CsCalS2, CsCalS5 and CsCalS9, and inside the membrane in CsCalS3, CsCalS4, CsCalS6, CsCalS7, CsCalS8 and CsCalS10. The central loop contains a putative catalytic domain that can be further subdivided into two domains: a UDP-glucose binding domain and a glycosyltransferase domain ( and ).
A phylogenetic tree based on the 10 CsCalS sequences and 12 AtCalS sequences was constructed using MEGA v6.0. In the tree shown in , the cucumber sequences cluster into four main subfamilies. The first subfamily contains four cucumber sequences (CsCalS3, CsCalS4, CsCalS5 and CsCalS6) and four sequences from Arabidopsis (AtCalS3, AtCalS4, AtCalS5 and AtCalS52). The second subfamily contains two sequences each from cucumber (CsCalS9 and CsCalS10) and Arabidopsis (AtCalS11 and AtCalS12), and the third subfamily comprises two cucumber (CsCalS7 and CsCalS8) and two Arabidopsis (AtCalS9 and AtCalS10) sequences as well. The fourth subfamily includes two sequences from cucumber (CsCalS1 and CsCalS2) and four from Arabidopsis (AtCalS1, AtCalS2, AtCalS7 and AtCalS8).
Figure 2. Phylogenetic tree of CsCalS and AtCalS proteins. The unrooted tree was constructed using Poisson-corrected distances in MEGA v6.0. Numbers at nodes are bootstrap support percentages based on 1,000 replicates. Accession numbers of cucumber and Arabidopsis CalS sequences are given in and , respectively.
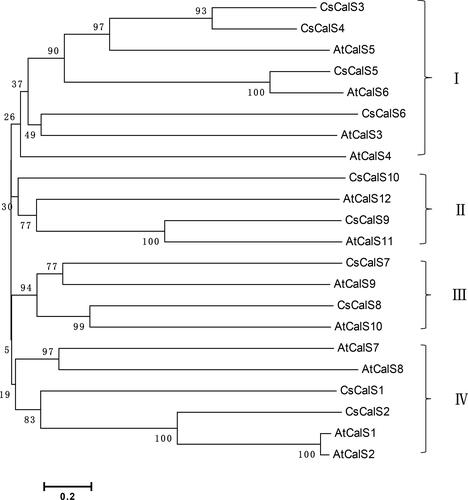
The sequence identities/similarities of CsCalS genes and encoded proteins ranged from 46–92% to 38–92%, respectively (). The closest homologs were CsCalS3 and CsCalS4, which exhibited 92% similarity.
Table 2. Identity/similarity matrix for CsCalS genes and proteins.
Expression patterns of CsCalS genes in cucumber
The CsCalS expression patterns in various tissues and organs, including roots, stems, leaves, male flowers, female flowers and fruits, were analyzed by qRT-PCR (). Although the 10 CsCalS genes were expressed in most selected organs, their expression levels were similar or varied considerably. For example, CsCalS5, CsCalS6, CsCalS7 and CsCalS8 have similar expression characteristics: they were mainly expressed in stems, male and female flowers. The highest expression of CsCalS5, CsCalS6 and CsCalS7 was found in female flowers, whilst CsCalS8 was found in male flowers. CsCalS1 and CsCalS10 were more highly expressed in the stem and female flowers than in other tissues. CsCalS9 was highly expressed in male and female flowers. Notably, CsCalS4 was mainly expressed in male flowers, where the expression level was nearly 20 times higher than in other parts, whereas there was hardly expression of CsCalS3 in male flowers. CsCalS2 was detected at similar expression levels in all selected organs, thus suggesting a role as a housekeeping gene. Genes with different expression patterns may have different functions. These results indicate that CsCalS genes are involved in various physiological and developmental processes.
Figure 3. Expression profiles of CsCalS genes in different organs/tissues based on quantitative real-time PCR analysis. Total RNA was isolated from roots (R), stems (S), leaves (L), fruits (F), female flowers (FF) and male flowers (MF). The TUA gene was used as an internal control. Data are presented as means ± SD (n = 3). The sequences of the PCR primers, which were designed to avoid amplification of conserved regions, are shown in .
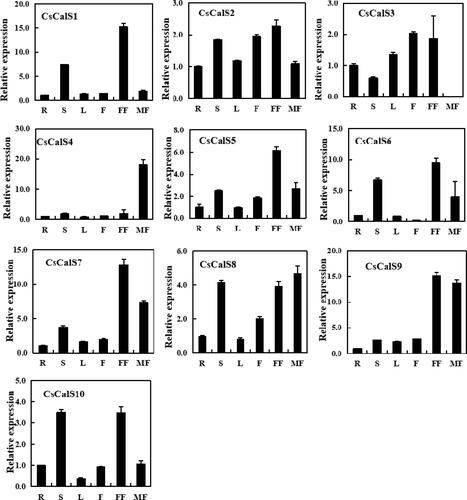
Expression patterns of CsCalS genes in response to biotic stress
Downy mildew and powdery mildew are the two main diseases severely affecting cucumber production. Callose plays an essential role in the plant defense responses to invading pathogens. To determine whether CsCalS is involved in response to pathogen stress, wild-type cucumber plants were treated with cucumber downy mildew and powdery mildew pathomycetes Pseudoperonospora cubensis and Sphaerotheca fuliginea, respectively. Water was used as a control. CsCalS gene expressions were then examined in the leaves (
Figure 4. Effect of biotic stress on the expression profiles of CsCalS genes. (A) Control treatment with water. (B) Inoculation with Pseudoperonospora cubensis. (C) Inoculation with Sphaerotheca fuliginea. Total RNA was isolated from leaves, and the TUA gene was used as an internal control. The data are presented as means ± SD (n = 4).
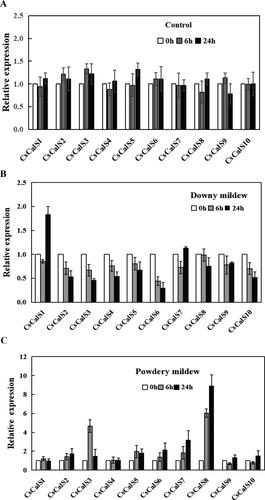
Under the control condition, the change in genes expression with time was not obvious (
Cucumber powdery mildew modulated by Sphaerotheca fuliginea generally caused the transcript levels of CsCalS genes to increase compared with the control as the infection time increased; the exceptions were CsCalS1, CsCalS4, CsCalS9 and CsCalS10, whose expression levels were changed slightly. In addition, the transcript levels of CsCalS3 and CsCalS8 were approximately five to six times higher than that of the control after 6 h of incubation, and CsCalS8 expression continued to increase to a level nearly 10 times higher than the control after 24 h of incubation (
Amino acid sequence structural analysis and subcellular localization of CsCalS4
According to our analysis of CsCalS gene expression patterns in cucumber, CsCalS4 is mainly expressed in male flowers. We therefore further focussed on the expression characteristics and localization of CsCalS4.
The amino acid sequence analyses indicated that CsCalS4 possesses an FKS1 domain, Vta-like domains and a large hydrophilic domain thought to provide glycosyl transferase catalytic activity at the C-terminus (
Figure 5. Amino acid sequence structure and subcellular localization of CsCalS4. (A) Predicted protein sequential element of CsCalS4 analysis. (B) The predicted topology of CsCalS4 in the cell membrane. Vertical blue bars indicate the transmembrane helices of CsCalS4. The length of the peptide chain in each non-membrane-spanning segment is indicated by the number of amino acid residues. (C) Subcellular localization of CsCalS4-GFP. This includes a diagram of the CsCalS4::GFP construct, subcellular localization of the green fluorescent protein (GFP) alone and the CsCalS4-GFP fusion protein in onion epidermal cells. Bars = 50 µm. Green indicates fluorescence from GFP.
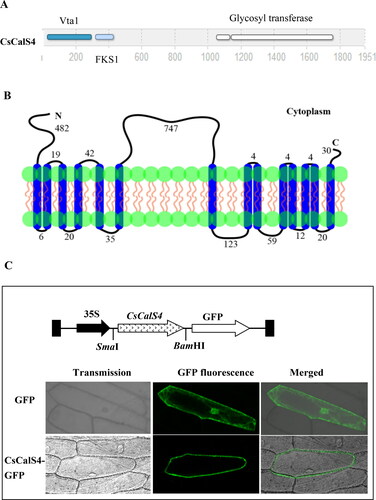
In order to detect the subcellular localization of CsCalS4, the 1254-bp CsCalS4 ORF was fused with the GFP reporter gene to produce the CsCalS4-GFP fusion protein. Transient expression experiments in onion epidermal cells showed that CsCalS4-GFP was mainly located in the plasma membrane, whilst the transformed onion epidermal cells containing only GFP showed fluorescence throughout the whole structure (
CsCalS4 expression during flower development and histochemical localization
To examine the spatial and temporal expression of CsCalS4 during flower development, we further examined the transcript levels of CsCalS4 in petals, sepals and anthers of male cucumber flowers. We observed that CsCalS4 was mainly expressed in anthers and only rarely in sepals and petals (
Figure 6. CsCalS4 expression during flower development in cucumber and histochemical localization in Arabidopsis. (A) Relative expression of CsCalS4 in sepals, petals, and anthers of stage-13 male flowers. (B) Relative expression of CsCalS4 at various stages of male flower development. The TUA gene was used as an internal control. Error bars indicate the SE from three technical replicates of three biological replicates. (C) Histochemical localization of CsCalS4 in Arabidopsis. This included diagram of the CsCalS4-promoter::GUS construct and localization of CsCalS4-promoter-GUS signals in an Arabidopsis inflorescence.
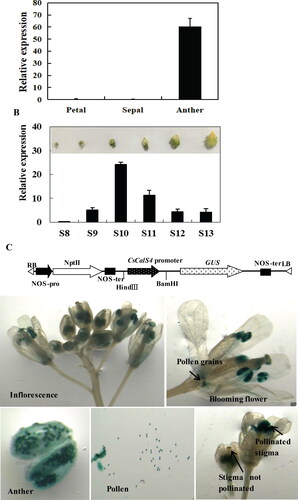
To confirm the CsCalS4 expression patterns and obtain detailed tissue-level expression profiles during plant development, we generated transgenic Arabidopsis plants expressing the GUS gene under the control of the CsCalS4 promoter. GUS activity was primarily limited to the anthers, and observation of anthers under magnification revealed that the staining was restricted to the pollen grains. GUS staining was also observed in the stigmas of pollinated flowers but not in unpollinated ones (
Discussion
The CalS gene family is widely distributed in plants and participates in many significant physiological processes, but members of this family have only been identified and characterized at the genome level in a few species. In this study, we explored 10 CsCalS genes in cucumber. These values are consistent with the 2000-amino acid size of most proteins encoded by CalS genes, which are larger than those of most plant genes. The 10 genes are distributed on the same or different chromosomes, and the number of exons and introns varies greatly amongst them (
Our phylogenetic analysis of Arabidopsis and cucumber CalS proteins divided the family into four main subfamilies (
Callose plays an important role in plant defense response. In plants invaded by a pathogenic fungus, callose may be deposited between the plasma membrane and cell wall or around stomata or may affect the plasmodesmata transport following pathogen attack [Citation39,Citation40]. In Arabidopsis, AtCalS1, AtCalS5, AtCalS9, AtCalS10 and AtCalS12 participate in the defense response to pathogen infection [Citation26]. AtCalS12 is closely associated with callose synthesis after pathogen invasion; the expression of AtCalS12 is induced by downy mildew (Hyaloperonospora), and its mutants exhibited reduced callose synthesis at the site of wounding and pathogen invasion [Citation26,Citation41,Citation42]. As another example of the important role of callose synthase in the resistance to fungal pathogens, increased callose deposition can enhance the resistance of plants to powdery mildew (Golovinomyces cichoracearum) [Citation43]. Downy mildew and powdery mildew respectively caused by Pseudoperonospora cubensis and Sphaerotheca fuliginea are the main destructive cucumber diseases in agricultural areas with warm and humid climate conditions. In the present study, CsCalS1 was up-regulated by P. cubensis, and CsCalS3 and CsCalS8 were up-regulated by S. fuliginea. These results suggest that these CsCalS genes may be involved in defense responses against downy mildew and powdery mildew disease attack. These findings will help better understand the role of CsCalS in fungal disease resistance and in functional analysis of the CsCalS gene family.
Callose participates in the process of plant sexual reproduction. Callose not only acts as mechanical isolation and chemical barrier to the sex cells (such as macrospore mother cells and microspore mother cells) that are about to divide, but also is necessary for the formation of the outer wall of pollen and the maintenance of pollen fertility and vitality during pollen development [Citation22,Citation44]. Callose walls formed during microspore formation can be divided into two types: the peripheral wall surrounding the four microspores and the interstitial wall separating the four microspores in the tetrad stage [Citation45]. In this study, we found CsCalS4, which is a plasma membrane protein (
Different members of the gene family may have different functions, may participate in different stages of plant development, or may have similar functions, resulting in functional redundancy. In Arabidopsis, various AtCalS genes are also expressed in pollen grains and have been reported to affect pollen development. The interstitial wall biosynthesis depends on the combined function of AtCalS11 and AtCalS12 [Citation18]. AtCalS9 and AtCalS10 play a role in the microspore entry into mitosis. Impaired AtCalS9 function resulted in dissymmetry of microspore division, irregular deposition of callose, and phagocytosis of germ cells by the cytoplasm of vegetative cells [Citation16,Citation20,Citation21]. AtCalS10 is necessary for microspore asymmetric mitosis [Citation19,Citation20]. In addition, inserted mutant plants of AtCalS7 also produced abnormal pollen grains and aborted embryos [Citation13]. In this study, except CsCalS4, all CsCalS7, CsCalS78 and CsCalS9 were widely expressed in male flowers (
Conclusions
In the present study, we performed a genome-wide analysis of the characteristics, transmembrane domain, phylogenetic relationships, expression levels of 10 CsCalS genes in different tissues of cucumber plants and under biotic stress and the location of CsCalS4. The results indicated that CsCalS2, similar to AtCalS7, may play an important role in phloem development and transport in cucumber. CsCalS1 and CsCalS8 were up-regulated by Pseudoperonospora cubensis and Sphaerotheca fuliginea, respectively, suggested that these CsCalS genes may be involved in the defense responses against downy mildew and powdery mildew disease attack. CsCalS4, encodes a plasma membrane protein, expressed mainly in pollen grains at St10 and St11 of cucumber male flowers. This indicated that CsCalS4 participated in the crucial pollen formation and maturation stages in cucumber male flowers. In addition, CsCalS7, CsCalS8 and CsCalS9 were also expressed in male flowers. They may also perform unique or redundant functions during male flower development. Our research results provide new and effective information for further understanding of the function of the CsCalS gene family in cucumber development and response to multiple biotic and abiotic stresses.
Data sharing
All data that support the findings reported in this study are available from the corresponding author upon reasonable request.
Supplemental Material
Download PDF (132.1 KB)Acknowledgment
We thank Liwen Bianji (Edanz) (www.liwenbianji.cn/), for editing the English text of a draft of this manuscript.
Disclosure statement
No potential conflict of interest was reported by the authors.
Funding
This work was supported by the National Natural Science Foundation of China under Grant No. 31801881.
References
- Chen XY, Kim JY. Callose synthesis in higher plants. Plant Signal Behav. 2009;4(6):489–492.
- Wang XQ, Wu Z, Wang LQ, et al. Cytological and molecular characteristics of pollen abortion in lily with dysplastic tapetum. Hortic Plant J. 2019;5(6):281–294.
- Nedukha OM. Callose: localization, functions, and synthesis in plant cells. Cytol Genet. 2015;49(1):49–57.
- Hong Z, Delauney AJ, Verma DP. A cell plate-specific callose synthase and its interaction with phragmoplastin. Plant Cell. 2001;13(4):755–768.
- Li J, Burton RA, Harvey AJ, et al. Biochemical evidence linking a putative callose synthase gene with (1 -> 3)-beta-D-glucan biosynthesis in barley. Plant Mol Biol. 2003;53(1–2):213–225.
- Liu F, Zou Z, Fernando WGD. Characterization of callose deposition and analysis of the callose synthase gene family of Brassica napus in response to Leptosphaeria maculans. Int J Mol Sci. 2018;19(12):3769.
- Sun M, Voorrips RE, Greet SB, et al. Reduced phloem uptake of Myzus persicae on an aphid resistant pepper accession. BMC Plant Biol. 2018;18(1):138–151.
- Niu Q, Zhang P, Su S, Jiang B, et al. Characterization and expression analyses of callose synthase enzyme (cals) family genes in maize (Zea mays L.). Biochem Genet. 2021;5:1–19.
- Richmond TA, Somerville CR. The cellulose synthase superfamily. Plant Physiol. 2000;124(2):495–498.
- Verma DPS, Hong Z. Purification of an elicitor-induced glucan synthase (callose synthase) from suspension cultures of French bean (Phaseolus vulgaris L.) purification and immunolocation of a probable Mr-65 000 subunit of the enzyme. Plant Mol Biol. 2001;47(6):693–701.
- Slewinski TL, Baker RF, Stubert A, et al. Tie-dyed2 encodes a callose synthase that functions in vein development and affects symplastic trafficking within the phloem of maize leaves. Plant Physiol. 2012;160(3):1540–1550.
- Shi X, Sun X, Zhang Z, et al. Glucan synthase-like 5 (GSL5) plays an essential role in male fertility by regulating callose metabolism during microsporogenesis in rice. Plant Cell Physiol. 2015;56(3):497–509.
- Xie B, Wang X, Zhu M, et al. CalS7 encodes a callose synthase responsible for callose deposition in the phloem. Plant J. 2011;65(1):1–14.
- Barratt DH, Kölling K, Graf A, et al. Callose synthase GSL7 is necessary for normal phloem transport and inflorescence growth in Arabidopsis. Plant Physiol. 2011;155(1):328–341.
- Hong Z, Zhang Z, Olson JM, et al. A novel UDP-glucose transferase is part of the callose synthase complex and interacts with phragmoplastin at the forming cell plate. Plant Cell. 2001;13(4):769–779.
- Chen XY, Liu L, Lee EK, et al. The Arabidopsis callose synthase gene GSL8 is required for cytokinesis and cell patterning. Plant Physiol. 2009;150(1):105–113.
- Thiele K, Wanner G, Kindzierski V, et al. The timely deposition of callose is essential for cytokinesis in Arabidopsis. Plant J. 2009;58(1):13–26.
- Enns LC, Kanaoka MM, Torii KU, et al. Two callose synthases, GSL1 and GSL5, play an essential and redundant role in plant and pollen development and in fertility. Plant Mol Biol. 2005;58(3):333–349.
- Nishikawa SI, Zinkl GM, Swanson RJ, et al. Callose (beta-1,3 glucan) is essential for Arabidopsis pollen wall patterning, but not tube growth. BMC Plant Biol. 2005;5:22.
- Töller A, Brownfield L, Neu C, et al. Dual function of Arabidopsis glucan synthase-like genes GSL8 and GSL10 in male gametophyte development and plant growth. Plant J. 2008;54(5):911–923.
- Huang L, Chen X, Yeonggil R, et al. Arabidopsis glucan synthase-like 10 functions in male gametogenesis. J Plant Physiol. 2009;166(4):344–352.
- Dong X, Hong Z, Sivaramakrishnan M, et al. Callose synthase (CalS5) is required for exine formation during microgametogenesis and for pollen viability in Arabidopsis. Plant J. 2005;42(3):315–328.
- Xie B, Wang X, Hong Z. Precocious pollen germination in Arabidopsis plants with altered callose deposition during microsporogenesis. Planta. 2010;231(4):809–823.
- Jacobs AK, Lipka V, Burton RA, et al. An Arabidopsis callose synthase, GSL5, is required for wound and papillary callose formation. Plant Cell. 2003;15(11):2503–2513.
- Nishimura MT, Stein M, Hou B, et al. Loss of a callose synthase results in salicylic acid-dependent disease resistance. Science. 2003;301(5635):969–972.
- Dong X, Hong Z, Chatterjee J, et al. Expression of callose synthase genes and its connection with Npr1 signaling pathway during pathogen infection. Planta. 2008;229(1):87–98.
- Sun LL, Sui XL, Lucas WJ, Li YX, et al. Down-regulation of the sucrose transporter CsSUT1 causes male sterility by altering carbohydrate supply. Plant Physiol. 2019;180(2):986–997.
- Holsters M, Silva B, Van Vliet F, et al. The functional organization of the nopaline A. tumefaciens plasmid pTiC58. Plasmid. 1980;3(2):212–230.
- Clough SJ, Bent AF. Floral dip: a simplified method for Agrobacterium-mediated transformation of Arabidopsis thaliana. Plant J. 1998;16(6):735–743.
- Tamura K, Stecher G, Peterson DS, et al. MEGA6: Molecular evolutionary genetics analysis version 6.0. Mol Biol Evol. 2013;30(12):2725–2729.
- Livak KJ, Schmittgen TD. Analysis of relative gene expression data using real-time quantitative PCR and the 2(-Delta Delta C(T)) Method. Methods. 2001;25(4):402–408.
- Jefferson RA, Kavanagh TA, Bevan MW. GUS fusions: beta glucuronidase as a sensitive and versatile gene fusion marker in higher plants. Embo J. 1987;6(13):3901–3907.
- Kelly R, Register E, Hsu M, et al. Isolation of a gene involved in 1,3-beta-glucan synthesis in Aspergillus nidulans and purification of the corresponding protein. J Bacteriol. 1996;178(15):4381–4391.
- Xie B, Hong Z. Unplugging the callose plug from sieve pores. Plant Signal Behav. 2011;6(4):491–493.
- Schneider R, Hanak T, Persson S, et al. Cellulose and callose synthesis and organization in focus, what’s new? Curr Opin Plant Biol. 2016;34:9–16.
- Wu SW, Kumar R, Iswanto ABB, et al. Callose balancing at plasmodesmata. J Exp Bot. 2018;69(22):5325–5339.
- Wang HY, Sui XL, Guo JJ, et al. Antisense suppression of cucumber (Cucumis sativus L.) sucrose synthase 3 (CsSUS3) reduces hypoxic stress tolerance. Plant Cell Environ. 2014;37(3):795–810.
- Fan JW, Wang HY, Li X, et al. Down-regulating cucumber sucrose synthase 4 (CsSUS4) suppresses the growth and development of flowers and fruits. Plant Cell Physiol. 2019;60(4):752–764.
- Gindro K, Pezet R, Viret O. Histological study of the responses of two Vitis vinifera cultivars (resistant and susceptible) to plasmopara infections. Plant Physiol Biochem. 2003;41(9):846–853.
- Iga T, Michael S, Tu GD, et al. A phytophthora effector protein promotes symplastic cell-to-cell trafficking by physical interaction with plasmodesmata-localised callose synthases. New Phytol. 2020;227(5):1467–1478.
- Vogel J, Somerville S. Isolation and characterization of powdery mildew-resistant Arabidopsis mutants. Proc Natl Acad Sci U S A. 2000;97(4):1897–1902.
- Østergaard L, Petersen M, Mattsson O, et al. An Arabidopsis callose synthase. Plant Mol Biol. 2002;49(6):559–566.
- Dorothea E, Annemarie GC, Jasmin K, et al. Interaction of the Arabidopsis GTPase RabA4c with its effector PMR4 results in complete penetration resistance to powdery mildew. Plant Cell. 2014;26(7):3185–3200.
- Motomura K, Takeuchi H, Notaguchi M, et al. Persistent directional growth capability in Arabidopsis thaliana pollen tubes after nuclear elimination from the apex. Nat Commun. 2021;12(1):2331.
- McCormick S. Control of male gametophyte development. Plant Cell. 2004;16(Suppl 1):S142–S153.