Abstract
Angelica dahurica is an ancient herbaceous plant and a famous traditional Chinese medicine. A. dahurica polysaccharides (AdPs) are macromolecular bioactive carbohydrates that have many functions. The animal gut contains a remarkable abundance of microbes that are closely related and play a major role in maintaining the overall health. The intestinal flora is also known as the ‘second genome’ of the human body. To study the functional interactions between AdPs and gut microbiota, we extracted AdPs using water extraction. After a series of treatments, including degreasing, alcohol precipitation, protein decolorization and purification by cellulose column, 200 mg/kg of purified AdPs were administered daily to 5 weeks old Kunming (KM) mice for 21 days. Microbial composition was evaluated in fecal samples using high-throughput sequencing. Our results revealed that AdPs affected the composition and structure of gut microbiota and can potentially regulate the intestinal flora as prebiotics.
Introduction
The dried root of Angelica dahuricae (Bai Zhi) plant is used as an ancient traditional Chinese medicine [Citation1]. Previous studies have shown that A. dahurica has many pharmacological effects, such as analgesic, anti-inflammatory, antibacterial, anti-tumorigenic and antioxidant, as well as regulating the central nervous system and reducing blood glucose [Citation2]. Due to its aromatic properties, A. dahurica is also used in cosmetics, health care, spices and as food additives [Citation3].
The active components of A. dahurica are coumarin, volatile oils, polysaccharides, starch and essential oils. Studies have shown that a polysaccharide from A. dahurica exhibits strong antioxidant activity and could be used as a potential novel natural antioxidant in food and medicine [Citation4]. A. dahurica polysaccharides (AdPs), through toll-like receptor 4 (TLR4) and its downstream signalling, can activate dendritic cells that play an important role in initiating antitumor immune responses [Citation5].
In the present study, we aimed to understand the functional interactions between AdPs and gut microbiota and evaluate whether AdPs affect the probiotics or pathogens present in the body. AdPs were extracted using water extraction and subjected to a series of treatments, including degreasing, alcohol precipitation, protein decolorization, and purification by cellulose column. Subsequently, 5-week-old Kunming (KM) mice were gavaged orally with pure AdPs (200 mg/kg) every morning for 21 d. The change in the animals’ weight was monitored regularly while providing sufficient food and water. Microbial composition was evaluated in mouse fecal samples using high-throughput sequencing (HTS). The results showed that AdPs affected gut microbiota in mice and provided insights into the potential of A. dahurica polysaccharides to be used as a prebiotic.
Materials and methods
Purification of A. dahurica polysaccharides (AdPs)
Chinese traditional herbaceous A. dahurica was acquired from Luzhou City, Sichuan Province, China. Polysaccharides were purified using the standard method for polysaccharide purification, which is a convenient, practical and efficient method. Crude polysaccharides were extracted under the parameters of time (4 h) and temperature (100 °C). Then, the extracted polysaccharide aqueous solution was alcohol precipitated and decolorized. Finally, purified AdPs were obtained using ion exchange cellulose column elution, followed by dialysis and freeze-drying. The total sugar content of AdPs was determined using the sulphuric acid-phenol method with a glucose standard as a control [Citation6]. After the reaction, the absorbance was detected using a UV-visible spectrophotometer at 490 nm, and the total sugar content was calculated using a standard curve. Purified AdPs were prepared for the next experiment.
Animal experiment
Eight male KM mice with body weights (BW) of 20 ± 2 g were obtained from the Animal Experiment Center of Southwest Medical University (Luzhou City, Sichuan Province, China). The mice were kept in an environment with controlled humidity (60%) and temperature (23 °C), with 12 h of light and 12 h of darkness. They were able to freely obtain food and water. After 3 d of growth and adaptation to the environment, the mice were randomly divided into Control (ConT) and AdPs groups. In the morning, the ConT group (1) was orally gavaged once daily with distilled water, and the AdPs group (2) was orally gavaged once daily with 100 mg/kg of AdPs. All animal care and procedures strictly followed the rules approved by the Animal Care and Use Committee of Southwest Medical University. At the end of the experiment, the male KM mice were fixed on the console and their anuses gently wiped with sterile cotton swabs to initiate a stress response and cause the mice to defecate. Mice feces were collected and placed in sterilised test tubes. Samples were transferred into liquid nitrogen and then, stored in a freezer (–80° C) for future research and analysis.
16S Ribosomal RNA (rRNA) gene and bioinformatics analysis
A genomic DNA isolation kit was used to extract DNA from mouse feces [Citation7]. The V3-V4 hypervariable region of the 16S rRNA gene was amplified from genomic DNA through polymerase chain reaction (PCR) [Citation8] and subjected to high-throughput sequencing (HTS) which was performed at Majorbio Company (Shanghai, China). Before analysis, the sequences were demultiplexed and quality-filtered using QIIME2 platform. After quality control, sequences were analysed using QIIME2 (v2020.2), with 100% similarity classified as an amplicon sequence variant (ASV). 16S rRNA genes were assigned to different classification categories using the ribosomal database item classifier algorithm and the Silva (SSU123) database, and differences between the two groups were tested using the Wilcoxon rank-sum test. The component differences between the two groups were analysed using principal coordinate analysis (PCoA) and R packages. Linear discriminant analysis Effect Size (LEfSe) was used to analyse the reasons for the differences in bacterial composition.
Statistical analysis
Data of all analyses were expressed as mean ± standard deviation (SD). We evaluated and calculated the significance of differences between groups using the statistical software GraphPad Prism (7.04). p < 0.05 was considered statistically significant.
Results
Effect of AdPs on gut microbiota composition
The relative abundance of each phylum was calculated using community barplot analyses. Firmicutes, Bacteroides, Deferribacterota and Campilobacterota were the main bacterial phyla occurring in the mouse gut microbiota. Compared with the ConT group, the Firmicutes in the AdPs-supplemented group increased; however, the abundance of Bacteroides tended to decrease after the supplementation with AdPs. However, Deferribacterota and Campilobacterota showed no obvious changes between the ConT and AdPs group. Community barplot analyses showed that Firmicutes and Bacteroides were fundamental in the mouse gut microbiota, and the experimental results showed that AdPs modulated the composition of gut microbiota in mice (). Therefore, AdPs had a distinctive influence on the composition of the mouse intestinal microbiota.
Figure 1. (A) Effects of A. dahurica polysaccharides (AdPs) on the relative abundance of gut microbiota at the phylum level. (B) Heatmap of fecal microbiota at the genus level.
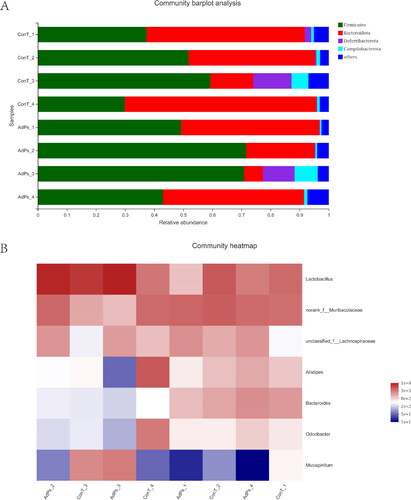
In order to determine the differences in the gut community at the genus level after intragastric administration of AdPs in mice, heatmap analysis was performed. The heatmap clearly exhibited the correlation among the predominant genera (). In contrast to the ConT group, the genus level of the mice gut microbiota was apparently altered by AdPs, and the most abundant bacteria were the representatives of genera Lactobacillus, Muribaculaceae and Lachnospiraceae. Based on the phyla levels, the roles of Bacteroidetes and Firmicutes in the metabolism of AdPs seemed to be complex.
Differences in gut microbiota composition
Modification of the gut microbial community in mice was quantitatively determined at the phylum level by 16S ribosomal RNA (rRNA) HTS analysis (). The ConT and AdPs groups were mainly composed of Bacteroides and Firmicutes, and their total relative abundance accounted for at least 90% of the total bacterial community. Compared with ConT group, the abundance of Firmicutes in the AdPs group increased (p < 0.05); however, the abundance of Bacteroides decreased, thereby increasing the ratio of Firmicutes to Bacteroides.
Modification of the gut microbial community in mice was quantitatively determined at the genus level by 16S rRNA HTS analysis (). The results showed that the AdPs and ConT groups comprised five main types of bacterial genera. Compared to the ConT group, the relative abundance of Lactobacillus and Norank-f-Muribaculaceae in the AdPs group increased, while the number of unclassified-f-Lachnospiraceae, Alistipes and Bacteroides Norank-f-Muribaculaceae decreased.
Effect of AdPs on gut microbiota structure
The effect of AdPs on mouse intestinal flora was studied by HTS analysis. Principal Co-ordinates Analysis (PCoA) was used to classify the microbiota between the ConT and AdPs groups. The results clearly showed the classification of a unique microbiota structure (), and the AdPs and ConT samples were clustered based on their respective groups. The first two components accounted for 41.63% and 22.91%, respectively, indicating that the structure of microbiota in the AdPs and ConT groups showed significant differences (p = 0.038).
LEfSe analysis
LEfSe analysis is used to find the species characteristics that can best explain the differences between two or more groups of samples and the impact of these characteristics. In our study, LEfSe analysis was performed to evaluate statistically distinct genera between the two groups. According to the influence of bacterial genera in each group, the higher the linear discriminant analysis (LDA) score, the more significant it is in the comparison and vice versa.
LEfSe analysis of microbial flora in the intestines of ConT and AdPs groups showed that there were 25 species that contributed to the difference of relative abundance among the two groups. The LEfSe results of gut microbiota composition was shown in . The predominant bacteria that caused the variation in gut microbiota between the ConT and AdPs groups were Clostridia, Lachnospirales and Lachnospiraceae. Additionally, Bacillus, Bacillales, and Bacillaceae played an important role in regulating the gut microbiota composition in the ConT group.
Discussion
Polysaccharides are one of the four basic groups of substances of life. As a macromolecular substance, it is widely present in nature [Citation9]. Plant polysaccharides, especially Chinese herbal polysaccharides, exhibit a wide range of pharmacological effects and have unique properties, such as antioxidant [Citation10], anti-diabetic [Citation11], anti-inflammatory [Citation3], anti-cancer [Citation12] and bone protective [Citation13]. Plant polysaccharides exhibit minimal toxicity in the human body, and can improve glucose metabolism and prevent diseases by changing the composition of the intestinal microbiota [Citation14]. Many studies have shown that most macromolecular polysaccharides can regulate various gut microbiota [Citation15], which is considered to be an important part of maintaining human health because of its ability to interfere with the mechanism of disease response [Citation16]. In the present study, the analysis of gut microbiota data showed that A. dahurica polysaccharides (AdPs) regulated gut microbiota composition. The intestinal microbiota of mice included the following different bacterial phyla: Firmicutes, Bacteroidetes, Deferribacterota and Campilobacterota (). Bacteroidetes and Firmicutes were the most prevalent phyla, accounting for almost 90% of the total bacteria in the intestine [Citation17]. Interestingly, the ratio of Firmicutes to Bacteroidetes decreased significantly after the administration of AdPs. The change of Firmicutes to Bacteroidetes indicated that polysaccharides may be conducive to weight loss [Citation18] and this ratio is a well-known hallmark of obesity [Citation19]. In addition, Firmicutes include a variety of gram-positive bacteria in the intestine and are the main producers of butyric acid, which is the main degradation product of non-digestible polysaccharides [Citation20]. Consistent with these findings, our results showed that the ratio of Firmicutes to Bacteroidetes in the Control (ConT) group was higher than that in the AdPs group (). Lactobacillus causes weight loss and ameliorates metabolic disorders. As a potential prebiotic, polysaccharides can be used to produce metabolites. The mechanism of action of Lactobacillus is closely related to obesity [Citation21]. These results collectively suggest that AdPs play a major role in changing the gut microbiota composition and enhancing the gut barrier function through their prebiotic ability, which is in accordance with the results of previous studies. Administration of AdPs promotes the growth of probiotics and significantly alters the composition of intestinal microbiota in mice.
Although our study is not exhaustive, the results show that the diversity of gut microbiota does not change, but AdPs altered the structure and proportion of gut microbiota. Therefore, AdPs have the potential to be used as prebiotics in the body as they are the main metabolites of gut microbiota and are significantly different between healthy and unhealthy individuals [Citation22]. Further experiments on determination of short chain fatty acids (SCFAs) would confirm our findings that AdPs have the function of probiotics. However, we speculate that as AdPs are pure heteropolysaccharides, this could be the reason for the unchanged intestinal microbial diversity. In a next study, we will purify the polysaccharides into a single component and further investigate the potential physiological effects of the gut microbiota altered by AdPs.
Conclusion
In conclusion, our study evaluated the gut microbial composition in mice after the administration of AdPs. Based on HTS analysis, Firmicutes, Bacteroidetes, Deferribacterota, and Campilobacterota were found to be the main bacterial phyla of the mouse gut microbiota. Notably, AdPs could change the composition and structure of gut microbiota, but the diversity between the AdPs and ConT groups did not change significantly. Taken together, these results provide insights into the potential of AdPs as a prebiotic in future studies.
Authors’ contributions
Conceived and designed the experiments: Can Song; performed the experiments: Qi Fang, Yu Luo and Yong Lai; contributed reagents/materials/analysis tools: Yu Luo and Hong Niu; wrote the paper: Yong Lai, Yu Luo and Can Song; created the figures: Can Song and Rui Wang. All authors reviewed the manuscript.
Ethical approval
All applicable international, national, and/or institutional guidelines for the care and use of animals were followed.
Disclosure statement
No potential conflict of interest was reported by the authors.
Data availability
The raw 16S rRNA sequences supporting the results of this study are available in the GenBank Database under the accession number PRJNA770123.
Additional information
Funding
References
- Guo J, Hu Z, Yan F, et al. Angelica dahurica promoted angiogenesis and accelerated wound healing in db/db mice via the HIF-1α/PDGF-β signalling pathway. Free Radic Biol Med. 2020;160:447–457.
- Wang R, Liu J, Yang DY, et al. Research progress in chemical constituents and pharmacological action of Angelica dahurica. Inform Trad Chinese Med. 2020;37(2):123–128.
- Yang WQ, Song YL, Zhu ZX, et al. Anti-inflammatory dimeric furanocoumarins from the roots of Angelica dahurica. Fitoterapia. 2015;105:187–193.
- Xu SF, Ye YP, Li XY, et al. Chemical composition and antioxidant activities of different polysaccharides from the roots of Angelica dahurica. Chem Biodivers. 2011;8(6):1121–1131.
- Kim HS, Shin BR, Lee HK, et al. Dendritic cell activation by polysaccharide isolated from Angelica dahurica. Food Chem Toxicol. 2013;55:241–247.
- Xu Y, Wu YJ, Sun PL, et al. Chemically modified polysaccharides: synthesis, characterization, structure activity relationships of action. Int J Biol Macromol. 2019;132:970–977.
- Zhu X, Xiang S, Feng X, et al. Impact of cyanocobalamin and methylcobalamin on inflammatory bowel disease and the intestinal microbiota composition. J Agric Food Chem. 2019;67(3):916–926.
- Guo XR, Lei H, Zhang KL, et al. Diversification of animal gut microbes and NRPS gene clusters in some carnivores, herbivores and omnivores. Biotechnol Biotechnol Equip. 2020;34(1):1280–1287.
- Sun SS, Wang K, Ma K, et al. An insoluble polysaccharide from the sclerotium of Poria cocos improves hyperglycemia, hyperlipidemia and hepatic steatosis in ob/ob mice via modulation of gut microbiota. Chin J Nat Med. 2019;17(1):3–14.
- Zheng YM, Shen JZ, Wang Y, et al. Anti-oxidant and anti-cancer activities of Angelica dahurica extract via induction of apoptosis in Colon cancer cells. Phytomedicine. 2016;23(11):1267–1274.
- Lee MY, Seo CS, Lee JA, et al. Anti-asthmatic effects of Angelica dahurica against ovalbumin-induced airway inflammation via upregulation of heme oxygenase-1. Food Chem Toxicol. 2011;49(4):829–837.
- Luo KW, Sun JG, Chan JY, et al. Anticancer effects of imperatorin isolated from Angelica dahurica: induction of apoptosis in HepG2 cells through both death-receptor- and mitochondria-mediated pathways. Chemotherapy. 2011; 57(6):449–459.
- Wang C, Sun J, Li H, et al. In vivo anti-inflammatory activities of the essential oil from Radix angelicae dahuricae. J Nat Med. 2016;70(3):563–570.
- Fang QY, Hu JL, Nie QX, et al. Effects of polysaccharides on glycometabolism based on gut microbiota alteration. Trend Food Sci Technol. 2019;92:65–70.
- Li X, Guo R, Wu X, et al. Dynamic digestion of tamarind seed polysaccharide: Indigestibility in gastrointestinal simulations and gut microbiota changes in vitro. Carbohydr Polym. 2020;239:116–194.
- Xie SZ, Liu B, Ye HY, et al. Dendrobium huoshanense polysaccharide regionally regulates intestinal mucosal barrier function and intestinal microbiota in mice. Carbohydr Polym. 2019;206:149–162.
- Wang M, Chen Y, Wang Y, et al. The effect of probiotics and polysaccharides on the gut microbiota composition and function of weaned rats. Food Funct. 2018;9(3):1864–1877.
- Chen G, Xie M, Wan P, et al. Digestion under saliva, simulated gastric and small intestinal conditions and fermentation in vitro by human intestinal microbiota of polysaccharides from fuzhuan brick tea. Food Chem. 2018;244:331–339.
- Ganesan K, Chung SK, Vanamala J, et al. Causal relationship between diet-induced gut microbiota changes and diabetes: a novel strategy to transplant Faecalibacterium prausnitzii in preventing diabetes. IJMS. 2018;19(12):3720.
- Crovesy L, Masterson D, Rosado EL. Profile of the gut microbiota of adults with obesity: a systematic review. Eur J Clin Nutr. 2020;74(9):1251–1262.
- Ottosson F, Brunkwall L, Ericson U, et al. Connection between BMI-related plasma metabolite profile and gut microbiota. J Clin Endocrinol Metab. 2018;103(4):1491–1501.
- Ahmadi S, Nagpal R, Wang S, et al. Prebiotics from acorn and sago prevent high-fat-diet-induced insulin resistance via microbiome-gut-brain axis modulation. J Nutr Biochem. 2019;67:1–13.