Abstract
The emergence of superbugs and resistant pathogens poses a challenge in scientific and medical research as they threaten public health worldwide. Many herbal natural products currently used in therapies have been suggested to exert antimicrobial, antiviral and even virucidal activities against a vast majority of impervious pathogens. Rhazya stricta, a folk medicinal desert plant from Saudi Arabia was recently revealed to exhibit bactericidal activity against multidrug-resistant (MDR) microorganisms. The pandemic caused by the severe acute respiratory syndrome coronavirus-2 (SARS-CoV-2) is a threat to public health worldwide. Hence, we examined the antiviral activity of R. stricta against the virus. The R. stricta water extract was prepared at the traditional dose. The antagonistic effects of this extract against pathogens have been proven in previous studies, and those against SARS-CoV-2 were shown in the present study. Therefore, we explored the effects of the plant extracts and fractions against the virus for future drug development. All plant extracts showed antiviral effects against SARS-CoV-2 in the Vero E6 cell lines. Non-alkaloids showed the strongest effect against the virus, followed by weak base alkaloids and finally strong base alkaloids. A cytotoxicity assay was performed to explore the safest dose with the strongest antiviral effects. The non-alkaloid extract derived from R. stricta leaves is a promising antiviral candidate for the development of potential drugs with appropriate activity against COVID-19 and other life-threatening diseases.
Introduction
Viral infections play a significant role in human diseases [Citation1]. Thus, identifying novel antiviral drugs is critically important, and natural products are an excellent source for such discoveries. Natural products are recognised as safe for human health, affordable and more importantly, capable of interfering at different stages of the viral life cycles. They can target host-specific interactions or simply have virucidal properties which unaffordable by synthetic medications. A mini-review [Citation1] described natural antiviral products as affecting a wide range of viral pathogens, such as Coronavirus (CoV), Coxsackievirus (CV), Dengue virus (DENV), Hepatitis B virus (HBV), Herpes simplex virus (HSV), Human immunodeficiency virus (HIV) and Influenza virus.
Currently, there is a growing interest in testing for phytochemicals as natural antiviral compounds. One of the most recent reviews on the subject [Citation2] mentioned new phytochemicals interfering with HSV-1 and HSV-2 infections. These phytochemicals were isolated from algae, fungi and plants. In their concluding remarks, the authors mentioned that many phytochemicals derived from these organisms were potent antiviral agents in cell culture assays and in vivo assays, as far as HSV is concerned. Furthermore, these compounds exert their effects through virucidal activity and the inhibition of viral entry into the target cell or even by interfering with the viral protein expression and viral nucleic acid replication processes, which are unique properties of these phytochemicals and are not exhibited by the drugs currently used in traditional practice. An excellent property of these drugs is that they prevent recurrent infection by the viral particles [Citation3]. Finally, the authors suggest that the advantages of these phytochemicals act synergistically to disable virus replication with no chance for the virus to mutate into a new resistant strain [Citation4, Citation5].
Many other familiar herbs widely used in folk medicine have been subjected to scientific studies. For example, oregano from the mint family was studied by [Citation6] as the oregano oil and its primary active molecule, carvacrol, showed antiviral activities against Murine Norovirus (MNV), a human norovirus surrogate. Furthermore, the study indicated that the oil and carvacrol exhibited potential sanitising properties for natural food to control Human Norovirus. Finally, an interesting investigation by [Citation7], who used Human Coronavirus NL63 (HCoV-NL63) to testing the antiviral effect of the leaf extract of a Chinese herb, Strobilanthes cusia (Nees), found that this extract exhibited a strong virucidal activity.
The coronavirus disease 2019 (COVID-19) pandemic started in late December 2019, in Wuhan, China. The etiological agent is a novel coronavirus, severe acute respiratory syndrome coronavirus-2 (SARS-CoV-2), named for the similarity of its symptoms to those induced by the severe acute respiratory syndrome coronavirus, SARS-CoV, according to the World Health Organisation (WHO) [Citation8]. Coronaviruses (CoVs) are a large group of viruses belonging to the family Coronaviridae that is highly transmitted to animals and humans, causing respiratory diseases and other severe illnesses. These viruses are enveloped, positive-sense single-stranded RNA viruses with genomes ranging in size from 26 to 32 kb, and use the same receptor as SARS-CoV (angiotensin-converting enzyme 2; ACE2). Severe acute respiratory syndrome (SARS) occurred in 2002, Middle East respiratory syndrome (MERS) occurred in 2012, and COVID-19 occurred in 2019, all of which are associated with severe and potentially fatal respiratory tract infections (Citation9–15]. Therefore, the testing of herbal products that have never been tested for antiviral activities has become necessary with the current outbreak of SARS-CoV-2. The candidate examined in this study was Rhazya stricta.
R. stricta is a desert shrub of the Apocynaceae family that is used in folk medicine. The plant was recorded for the first time in Saudi Arabia’s western region [Citation16, Citation17]. It has been used for many years in several countries to treat microbial infections, inflammation, cancer and diabetes [Citation18]. Many of these medicinal properties have been validated experimentally by several investigations, the most recent of which was the one by [Citation19], which demonstrated for the first time the bactericidal effect of this plant on multidrug-resistant (MDR) bacteria. In addition, via collaborations with some international institutions in the USA and UK, the whole genome of the R. stricta has been extensively studied, which may lead to breakthroughs in natural product genomics and phytodynamic therapy (PDT) in the future. "R. stricta is a plant that is used in indigenous medicine for its anticancer and antimicrobial properties. However, it is difficult for the general population to identify genuine samples of this plant when purchasing it in local markets" [Citation20]. Our previous studies proved the antimicrobial activities of the plant against microorganisms such as fungi [Citation20, Citation19] and superbugs [Citation19, Citation21, Citation23], and the different plant extracts and fractions showed promising results against MDR Staphylococcus aureus (MRSA).
To date, no therapeutics have been proven to be effective for the treatment of severe illness caused by SARS-CoV-2. Therefore, reliable knowledge of the structure of SARS-CoV-2 and the plant phytochemicals will help us find curative treatments for COVID-19.
Material and methods
Plant collection and processing
Fresh aerial parts of the R. stricta plant were collected from its natural habitat in the desert of the Al Gholah region near Asfan Road, Jeddah Province, Saudi Arabia. The voucher specimen was deposited in the Department of Biological Sciences Herbarium at King Abdulaziz University (Number 1150/M/75, collected by N. Baeshen, M. Baeshen and J. Sabir). The plant samples were taken directly to the laboratory in an ice box. Leaves were cut and washed under running water to remove the dust and left to dry in the laboratory at room temperature. One week later, the dry leaves were ground to a fine powder and stored at room temperature for further extraction and fractionation. For the preparation of the traditional water extract, 6 g of the plant powder was added to 1 L of sterilised distilled water and kept in a shaker overnight. The next day, the extract was filtered through a 0.22-µm sterile membrane and kept in a refrigerator for one week [Citation19]. Crude ethanolic extracts were prepared as described by [Citation21–23] to obtain the fractions of strong base alkaloids, weak base alkaloids and non-alkaloids. Each fraction was dissolved in 10% DMSO before the experiment to achieve the required concentration.
Viruses and cells
Vero E6 cells, a normal continuous kidney-epithelial cell line from African green monkey (ATTC code, VERO C1008), were kindly provided by St. Jude Research Hospital. The cells were maintained and subcultured in a complete growth medium, which was Dulbecco’s modified Eagle’s medium (DMEM) with 4.5 g/L glucose, L-glutamine and sodium pyruvate (DMEM LONZA BE12-604F) supplemented with 10% fetal bovine serum (Gibco™ 10270106) and 1% antibiotic- antimycotic (Gibco™ 15240062).
The cells were prepared in 96-well plates and 6-well plates for cytotoxicity, viral titration and reduction assays using infection medium (DMEM supplemented with 1% antibiotic- antimycotic and 0.3% bovine serum albumin (Invitrogen, Carlsbad CA, USA).
The SARS-CoV-2 virus used in this study was an Egyptian isolate, human Coronavirus 2019 (hCoV-19)/Egypt/NRC-03/2020 (hCoV-19_NRC-03, accession number in the GISAID database: EPI_ISL_430820), isolated from an oropharyngeal swab specimen from a 34-year-old woman from El-Minya Governorate [Citation25, Citation26].
The virus was propagated for two passages in the cell line and subjected to sequencing; the sequence is published in the GISAID database (EPI_ISL_430820). In addition, the propagated virus was subjected to titration by a plaque assay, and the TCID50 was determined to quantify the infectious particles.
All experiments with the live SARS-CoV-2 virus were performed at a certified biosafety level 3 facility after proper permissions were obtained from the relevant authorities.
Determination of half-maximal cytotoxic concentration(CC50)
Each obtained extract was dissolved in dimethyl sulfoxide (DMSO, Sigma-Aldrich) and stored at −80 °C. To assess the half maximal cytotoxic concentration (CC50), the extract stock solutions were diluted further with DMEM to obtain working solutions. The cytotoxicity was tested in Vero E6 cells by using the crystal violet assay as described by [Citation27] with minor modifications. Briefly, the cells were seeded in 96 well-plates (100 µL/well at a density of 3 × 105 cells/mL) and incubated for 24 h at 37 °C in 5% CO2. After 24 h, the cells were treated with various concentrations of extracts in triplicates. In addition, to select the safest DMSO concentration, the cell cultures were tested with different concentrations of DMSO. The assay revealed 10% DMSO to be safe. As previously described by [Citation28], at 72 h post-treatment, the supernatant was discarded, and cell monolayers were fixed with 10% formaldehyde for 1 h at room temperature (RT). The fixed monolayers were then dried well and stained with 50 µL of 0.1% crystal violet for 20 min on a bench rocker at RT. The monolayers were then washed and dried overnight, and the crystal violet dye in each well was then dissolved in 200 µL of methanol for 20 min on a bench rocker at RT. The absorbance of the crystal violet solutions was measured at RT and λmax 570 nm (as a reference wavelength) using a multiwell plate reader. The CC50 value was calculated using non-linear regression analysis in GraphPad Prism software (version 5.01) by plotting log concentrations of the plant extracts versus the normalised response (variable slope).
Determination of half-maximal inhibitory concentration 50 (IC50)
Cells were distributed in 96-well tissue culture plates, with 2.4 × 104 VeroE6 cells in each well, and incubated overnight in a humidified 37 °C incubator with 5% CO2. The cell monolayers were then washed once with 1x PBS. An aliquot of the SARS-CoV-2 "NRC-03-nhCoV" virus [Citation26] containing 100 TCID50 (the 50% Tissue Culture Infectious Dose Assay) of the virus was incubated with serial dilutions of the tested compound and kept at 37 °C for 1 h. The VeroE6 cell monolayers were treated with the virus/compound mixtures and coincubated at 37 °C in a total volume of 200 µL per well. Untreated cells that were infected with the virus represented the "virus control", whereas cells that were not treated and not infected were the "cell control". Following incubation at 37 °C in a 5% CO2 incubator for 72 h, the cells were fixed with 100 μL of 10% paraformaldehyde for 20 min and stained with 0.5% crystal violet in distilled water for 15 min at RT. The crystal violet dye was then dissolved using 100 μL of absolute methanol per well, and the optical density of the colored solution was measured at 570 nm using an AnthosZenyth200rt plate reader (AnthosLabtec Instruments, Heerhugowaard, Netherlands). The half maximal inhibitory concentration (IC50) of the compounds was required to reduce the virus-induced cytopathic effect (CPE) by 50% relative to the virus control. The IC50 value was calculated using non-linear regression analysis in GraphPad Prism software (version 5.01) by plotting log concentrations of the plant fractions versus the normalised response (variable slope).
Results
Determination of the cytotoxic activity of R. stricta plant extracts using VeroE6 cells
To search for a new and promising anti- SARS-CoV-2 candidate molecule from medicinal plants, we chose various extracts of R. stricta. Initially, we prepared a water extract of the plant to test our hypothesis. As shown in , we found that the water extract had significant anti- SARS-CoV-2 activity. Encouraged by this discovery, we prepared several other plant extracts, including non-alkaloidal, strong base alkaloids and weak base alkaloidal fractions, as described in the Materials and Methods section. First, we investigated the toxicity of the different plant fractions in Vero E6 cells, which are widely used for viral infection, isolation and propagation [Citation29]. The cells were treated with various concentrations of the extracts, and the cell viability was measured using the crystal violet assay (). The CC50 value of the plant extracts and fractions were measured: strong alkaloid fraction (CC50: 0.12 mg/mL), water extract (CC50: 2.0 mg/mL), non-alkaloidal fraction (CC50: 0.18 mg/mL) and the weak base alkaloids (CC50: 0.0464 mg/mL) (). These results suggest a variation in the degree of the cell cytotoxicity of the various plant extracts.
Figure 1. Half-maximal cytotoxic concentration (CC50) and half-maximal inhibitory concentration (IC50) of various R. stricta plant extracts against Vero E6 cells and SARS-CoV-2-infected Vero E6 cells, respectively. The graphs on the left (a, c, e and g) show the CC50 of various plant extracts. Vero E6 cells were treated with various concentrations of the extracts. Cell viability was examined by using a crystal violet assay. Absorbance was measured at λmax 570 nm. The graphs on the right (b, d, f, and h) show the IC50 against SARS-CoV-2 infected Vero E6 cells. The CC50 and IC50 values were calculated using non-linear regression analysis of GraphPad Prism.
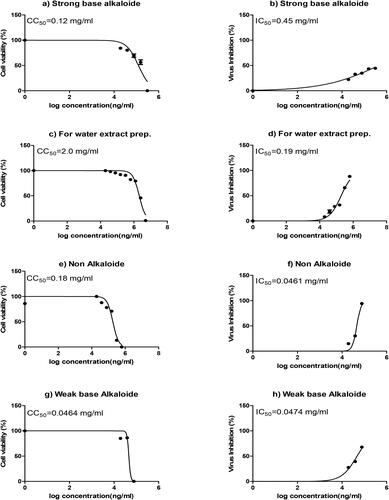
Determination of the IC50 of the R. stricta plant extracts against SARS-CoV-2 infected vero E6 cells
After measuring the cytotoxic effect of the plant extracts, we measured the IC50 values of the various plant extracts on SARS-CoV-2-infected Vero E6 cells. We performed a dose-response analysis. Vero E6 cells were infected with SARS-CoV-2 at 100 TCID50 for 1 h and subsequently treated with different plant extracts at various concentrations. Our results showed that the anti-SARS-CoV-2 effect of the different plant extracts was dose dependent (). The IC50 values of the various plant extracts were as follows: 0.45 mg/mL for the strong base alkaloid fraction, 0.19 mg/mL for the water extract, 0.0461 mg/mL for the non-alkaloidal fraction, and 0.0474 mg/mL for the weak base alkaloid fraction (). These results indicated the dose-dependent inhibitory effect of various plant extracts on SARS-CoV-2-infected Vero E6 cells. In vitro antiviral assays showed that the different plant extracts and fractions had varying antiviral effects against SARS-CoV-2. This may be due to the presence of different bioactive molecules in these extracts. Our previous studies [Citation20, Citation21] on the antimicrobial effects of the R. stricta water extract showed promising results against microorganisms in general, and particularly MDR bacteria [Citation19], which encouraged us to test the effect of the plant’s organic fractions against SARS-CoV-2. The results of the present study are promising, and the need for future research into the effect of the different fractions of the plant extract is essential for the development of antiviral and/or virucidal drugs against SARS-CoV-2. Moreover, including other examples of potential medicinal herbs that have anti-human coronavirus (anti-HcoV) therapeutic activities and/or applications in the treatment of COVID-19 symptoms, as shown in [Citation30]. In contrast to our results, small plant molecules such as polyphenols are found to inhibit coronavirus infections.
Discussion
The emergence of deadly pathogens and their resistant forms is a major public health concern. The recent emergence of SARS-CoV-2 and its various mutant forms has posed a serious challenge to human health worldwide that has never been experienced before. Despite the significant gain in knowledge, data for the virological, clinical and epidemiological aspects of the infection is still generally lacking. Identifying new antiviral agents is of particular interest, as the treatment options are currently limited. In this challenging time, medicinal plants and traditional medicine have proven to be a blessing for the scientific community due to their good track record and acceptance among the general public. Plant extracts can exert an inhibitory effect against SARS-CoV-2 infection at both pre-entry and post-infection phases [Citation30, Citation33]. In the present study, we used an extract of the traditional medicinal plant R. stricta, which is known to have various therapeutic effects against many diseases and infections [Citation19, Citation32]. Therefore, we used different extracts of this plant against SARS-CoV-2 in cell lines as described below
Our results showed that the non-alkaloidal fraction had the highest antiviral activity against SARS-CoV-2, with an IC50 of 0.0461 mg/mL and a CC50 of0.0461 mg/mL, followed by weak base alkaloids (IC50: 0.0474 mg/mL; CC50: 0.0464 mg/mL). Finally, strong base alkaloids showed the weakest effect (IC50: 0.45 mg/mL; CC50: 0.12 mg/mL). These results are consistent with the results of our previous studies [Citation22, Citation23], where the organic fractions showed stronger antimicrobial effects against resistant microorganisms and supported the strong effects of the non-alkaloidal fraction of the plant. We believe that the existence of several types of chemical compounds in these extracts is responsible for antiviral activity. This activity might be attributed to a single compound or a combination of two or more compounds, which needs to be addressed in a future work. The synergistic effect of different chemical constituents in the different plant extracts may be responsible for the different cell toxicity and antiviral activities. Fine-tuning of these molecules by various chemical modifications will be a useful strategy to enhance the antiviral activities of the native chemical molecules in these extracts. We also believe that these plant extracts can also be used along with other antiviral medicines or compounds in combinatorial therapy.
This would be supported by the design of an anti-SARS-CoV2 treatment that exhibits the potential antiviral or virucidal activity of plant extracts and specific compounds in in vitro and in vivo experiments. Therefore, further investigations will be supported afterwards by preclinical studies. However, novel screening strategies for target activities are extremely important [Citation33]. Even though some plant-specific compounds and their extracts, such as lignans, saponins, alkaloids, kaempferol and flavonoids, play various roles in inhibiting the viral entry, destroying the nucleocapsid and genetic material, and inhibiting the replication of viruses (SARS strains included), certain essential factors affect the virulence and pathogenesis of COVID-19. These plant phytochemicals will help us to find a treatment to combat these coronavirus infections [Citation34]. In addition, an important protein present on the virus called the spike (S) protein, is the final determinant of viral entry into cells, leading to deregulation of multiple pathways as described in [Citation35].
This study, however, had several limitations since there was little or no prior research on a specific treatment for COVID-19. Consequently, this led us to establish a new line of research to identify potential therapeutic candidates. On the other hand, future research is needed to address and determine the possible bioactive compounds.
Conclusions
We have identified R. stricta plant extracts as promising candidates for use against SARS-CoV-2. Our present work also highlights the importance of using and applying traditional medicinal plants against deadly human diseases and emerging pathogens. However, we believe that using R. stricta extracts against SARS-CoV-2 and its variants will be promising as long as we can adjust its toxicity to be minimal in humans. In summary, further research is needed to understand the mechanism of action of different plant extracts of R. stricta. Isolation of bioactive molecules from these extracts will be an active area of future research. We would also like to develop and apply a high-level screen for antiviral candidates from plant extracts coupled with imaging techniques and other tools that demonstrate the mechanism of neutralising the virus via the binding of plant molecules with the viral S proteins, such as ACE2 (angiotensin-converting enzyme 2), which mediates viral entry into the host. In addition, the inhibitory effect against SARS-CoV-2 replication and infectivity will be illustrated with cytotoxicity profiling or viral distraction.
Since R. stricta is easily available in various parts of the world, the use of its extracts offers new therapeutic opportunities in the battle against SARS-CoV-2 and in attempts to find a new potential anti-HCoV drug by combining these approaches.
Author contributions statements
N.A.B., N.N.B., A.A.J. and M.N.B. designed the project and wrote the manuscript. M.N.B., A.O.A. and Y.Q.A. collected the plant material and performed plant experiments, plant extraction and fractionation. T.A.B., R.A., S.H.M., N.MA.S. and M.A.A. performed antiviral activity experiments and results analysis. A.K. and B.A. worked on providing project supplies and correspondence. M.B., S.Z. and K.A. provided and achieved cell lines experiments. A. M. finalized and edited the manuscript. All authors reviewed the manuscript.
Acknowledgement
The authors acknowledge with thanks the Ministry of Health for technical support.
Data availability statement
The authors confirm that the data supporting the findings of this study are available within the article.
Disclosure statement
No potential conflict of interest was reported by the authors.
Funding
This work was funded by the Ministry of Health under grant No. 535 and date: 20/4/2020.
Additional information
Funding
References
- Lin L, Hsu W, Lin C. Antiviral natural products and herbal medicines. J Tradit Complement Med. 2014;4(1):24–35.
- Álvarez DM, Castillo E, Duarte LF, et al. Current antivirals and novel botanical molecules interfering with herpes simplex virus infection. Front Microbiol. 2020;11:139.
- Ibáñez FJ, Farías MA, Retamal-Díaz A, et al. Pharmacological induction of heme oxygenase-1 impairs nuclear accumulation of herpes simplex virus capsids upon infection. Front Microbiol. 2017;8:2108.
- Chugh NA, Bali S, Koul A. Integration of botanicals in contemporary medicine: road blocks, checkpoints and go-ahead signals. Integr Med Res. 2018;7(2):109–125.
- Yuan H, Ma Q, Ye L, et al. The traditional medicine and modern medicine from natural products. Molecules. 2016;21(5):559.
- Gilling DH, Kitajima M, Torrey JR, et al. Antiviral efficacy and mechanisms of action of oregano essential oil and its primary component carvacrol against murine norovirus. J Appl Microbiol. 2014;116(5):1149–1163.
- Tsai Y, Lee C, Yen H, et al. Antiviral action of tryptanthrin isolated from Strobilanthes cusia leaf against human coronavirus NL63. Biomolecules. 2020;10(3):366.
- World Health Organisation (WHO). 2020. Retrieved from https://www.who.int/emergencies/diseases/novel-coronavirus-2019.
- Gorbalenya, A. E., Baker, S. C., Baric, R., Groot, R. J. D., Drosten, C., Gulyaeva, A. A., ... & Ziebuhr, J. Severe acute respiratory syndrome-related coronavirus: The species and its viruses–a statement of the Coronavirus Study Group. bioRxiv, 7. Preprint
- Gorbalenya, A. E., Baker, S. C., Baric, R. S., De Groot, R. J., Drosten, C., Gulyaeva, A. A., ... & Ziebuhr, J. The species Severe acute respiratory syndrome-related coronavirus: classifying 2019-nCoV and naming it SARS-CoV-2. Nat Microbiol. 2020b; 5:536–544. https://Go.Nature.Com/3cW9qJR
- Chang L, Yan Y, Wang L. Coronavirus disease 2019: coronaviruses and blood safety. Transfus Med Rev. 2020;34(2):75–80.
- Guo Y, Cao Q, Hong Z, et al. The origin, transmission and clinical therapies on coronavirus disease 2019 (COVID-19) outbreak–an update on the status. Military Med Res. 2020;7(1):1–10.
- Jiang F, Deng L, Zhang L, et al. Review of the clinical characteristics of coronavirus disease 2019 (COVID-19). J Gen Intern Med. 2020;35(5):1545–1549.
- Li G, Fan Y, Lai Y, et al. Coronavirus infections and immune responses. J Med Virol. 2020;92(4):424–432.
- Tang X, Wu C, Li X, et al. On the origin and continuing evolution of SARS-CoV-2. Natl Sci Rev. 2020;7(6):1012–1023.
- Batanouny KH, Baeshin NA. Studies on the flora of Arabia II.the Medina-Badr Road. Saudi Arabia. Bull Faculty Sci KAU Jeddah. 1982;6:1–26.
- Batanouny KH, Baeshin NA. Plant communities along the medina-badr road across the Hejaz mountains, Saudi Arabia. Vegetatio. 1983;53(1):33–43.
- Khan SA, Baeshen MN, Ramadan HA, et al. ITS2: an ideal DNA barcode for the arid medicinal plant rhazya stricta. Pharmaceut Med. 2019;33(1):53–61.
- Baeshen MN, Al-Attas SG, Ahmed MM, et al. The effect of Rhazya stricta aqueous leaves extract on MRSA genotypes in Jeddah province. Biotechnol Biotechnol Equip. 2016;30(2):368–374.
- Baeshin NA, Twaty N, Al-Hebshi A. Evaluating the genotoxicity of Rhazya stricta leaves extract by the Saccharomyces cerevisiae auxotrophic mutants test. Egypt J Nat Toxins. 2005;2:87–100.
- Baeshin NA, Qari SH, Sabir J, et al. Biochemical and molecular evaluation of genetic effects of Rhazya stricta (decne) l leaves extract on Aspergillus terreus. Saudi J Biol Sci. 2008;15(1):25–33.
- Khan R, Baeshen MN, Saini KS, et al. Antibacterial activity of Rhazya stricta non-alkaloid extract against methicillin-resistant Staphylococcus aureus. Biol Syst Open Access. 2016a;5(157):2.
- Khan R, Baeshen MN, Saini KS, et al. Antibacterial activities of Rhazya stricta leaf extracts against multidrug-resistant human pathogens. Biotechnol Biotechnol Equip. 2016b;30(5):1016–1025.
- Elkady AI, Hussein RAEH, Abu-Zinadah OA. Effects of crude extracts from medicinal herbs Rhazya stricta and Zingiber officinale on growth and proliferation of human brain cancer cell line in vitro. Biomed Res Int. 2014;2014:260210.
- Gomaa MR, Kandeil A, Mostafa A, et al. Prevalence of severe acute respiratory syndrome coronavirus 2 neutralizing antibodies in egyptian convalescent plasma Donors. Front Microbiol. 2020;11:596851..
- Kandeil A, Mostafa A, El-Shesheny R, et al. Coding-complete genome sequences of two SARS-Cov-2 isolates from Egypt. Microbiol Resour Announc. 2020;9(22):489.
- Feoktistova M, Geserick P, Leverkus M. Crystal violet assay for determining viability of cultured cells. Cold Spring Harb Protoc. 2016;2016(4):pdb.prot087379.
- Mahmoud A, Mostafa A, Al-Karmalawy AA, et al. Telaprevir is a potential drug for repurposing against SARS-CoV-2: computational and in vitro studies. Heliyon. 2021;7(9):e07962. 10.1016/j.heliyon.2021.e07962
- Matsuyama S, Nao N, Shirato K, et al. Enhanced isolation of SARS-CoV-2 by TMPRSS2-expressing cells. Proc Natl Acad Sci U S A. 2020;117(13):7001–7003.
- Ghoran SH, El-Shazly M, Sekeroglu N, et al. Natural products from medicinal plants with anti-human coronavirus activities. Molecules. 2021;26(6):1754.
- Kanjanasirirat P, Suksatu A, Manopwisedjaroen S, et al. High-content screening of Thai medicinal plants reveals Boesenbergia rotunda extract and its component Panduratin A as anti-SARS-CoV-2 agents. Sci Rep. 2020;10(1):1–12.
- Baeshen MN, Khan R, Bora RS, Baeshen NA. Therapeutic potential of the folkloric medicinal plant Rhazya stricta. Biol Syst Open Access. 2015;5:151.
- Matveeva T, Khafizova G, Sokornova S. In search of herbal anti-SARS-Cov2 compounds. Front Plant Sci. 2020;11:589998.
- Anand AV, Balamuralikrishnan B, Kaviya M, et al. Medicinal plants, phytochemicals, and herbs to combat viral pathogens including SARS-CoV-2. Molecules. 2021;26(6):1775.
- Singh RS, Singh A, Kaur H, et al. Promising traditional Indian medicinal plants for the management of novel coronavirus disease: a systematic review. Phytother Res. 2021;35(8):4456–4484.
- Coronaviridae Study Group of the International Committee on Taxonomy of Viruses. The species severe acute respiratory syndrome-related coronavirus: classifying 2019-nCoV and naming it SARS-CoV-2. Nat Microbiol. 2020;5:536–544. https://Go.Nature.Com/3cW9qJR.