Abstract
The degradation of organic pollutants in plants involves uptake and diffusion through the roots, trunk or leaves, transformation, accumulation and/or volatilization of soil- and aqueous-phase contaminants. 2,4,6-trinitrotoluene (TNT), the most widely used explosive, is toxic to a number of photosynthetic organisms, poses hazards to human health and pollutes the environment because it is recalcitrant to degradation. In this study, we investigated the nitroreductase gene from Citrobacter freundii in transgenic Arabidopsis thaliana plants. Transgenic plants showed a promising ability to tolerate, take up and detoxify TNT. Our results suggest that transgenic plants show higher potential for removing and transformation of TNT. The expression of nitroreductase in Arabidopsis could be useful for phytoremediation and could be explored for the effective cleanup of TNT-contaminated sites.
Introduction
TNT (2,4,6-trinitrotoluene) is one of the most widely used military and civil explosives, which has a huge negative impact on the environmental ecosystems [Citation1]. TNT, RDX and HMX have been listed as priority pollutants by the United States Environmental Protection Agency [Citation2]. Explosives in soil and water can cause toxic liver disease, anemia and other damage, serious poisoning and even death after entering the human body [Citation3, Citation4]. Therefore, TNT degradation has attracted the attention of many environmental protection agencies and several agencies have made the removal of TNT a high priority [Citation5–7].
The operation cost of phytoremediation technology is much lower than that of traditional methods. It has the advantages of beauty, safety, easy operation and in-situ disposal of contaminated soil [Citation8–10]. Although plants can detoxify TNT, they usually lack the catabolic pathway of complete degradation/mineralization of TNT compared with microbial [Citation11]. The purpose of genetic modification (GM) technology is to degrade TNT by expressing microbial TNT detoxification gene in plants [Citation12]. Phytoremediation is an approach that could be used to clean-up explosive pollution and could be a solution to the high phytotoxicity of TNT [Citation13].
In a review, Khan et al. [Citation14] have demonstrated the microbial degradation intermediates of TNT and its implications in bioremediation. Gunning et al. [Citation15] have reported that Arabidopsis thaliana plants overexpressing GST-U24 and GST-U25 exhibit significantly enhanced ability to withstand and detoxify TNT. Moreover they also published another paper about the structure of GSTU25 and they identified key residues involved in the formation of 2-glutathionyl-4,6-dinitrotoluene (2-GDNT) [Citation16]. Johnston’s research showed that A. thaliana plants with a mutation in the MDHAR6 gene could grow more tenaciously with residual TNT. The plants have longer root systems and greater mass than normal plants. In other words, they are more resistant to environments with higher residual concentrations of TNT [Citation17]. Zhang et al. [Citation18] reported that transgenic grass was used for phytoremediation of explosive and environmental pollutants. Meanwhile, they first reported the detoxification of TNT with transplastomic plants: they expressed nfsI in the plastids of tobacco and examined the response of the plants to TNT [Citation19]. A study has shown that western wheatgrass (Pascopyrum smithii) transformed with xplA, xplB and nfsI were more resistant to TNT toxicity, and detoxifed more TNT than wild-type plants [Citation13]. Yang et al. showed that the exposure to TNT, RDX and HMX could lead to imbalance of plant photosynthetic characteristics and antioxidant enzymes; it could change the basic metabolism of plants and has significant ecotoxicity [Citation3].
The aim of this study was to introduce the nfsb gene from Citrobacter freundii into plants and test whether transgenic plants can eliminate TNT more effectively. The results showed that NFSBI transgenic plants displayed an enhanced growth phenotype, higher nitroreductase activity and higher TNT degradation efficiency. The results will be useful for future studies on the possible phytoremediation.
Materials and methods
Plant material and growth conditions
The A. thaliana (A. thaliana ecotype Columbia) was used in this study. All the transgenic lines and wild-type of Arabidopsis were maintained at 22 °C under a 16 h light/8 h dark regime at 6000–8000 lx, unless stated otherwise.
Design and chemical synthesis of the CfNFSBI gene
We synthesized a nitroreductase gene, CfNFSBI, on the basis of the encoding amino acid of the wild-type gene from Citrobacter freundii ATCC8090 (GenBank Accession No: WP_003831162) through a PCR-based two-step DNA synthesis (PTDS) strategy [Citation20, Citation21]. Polymerase chain reaction (PCR) was carried out in a total volume of 50 μL containing 30 pmol of each outer primer and 1.5 pmol of each inner primer for 25 cycles of 94 °C for 30 s, 54 °C for 30 s and 72 °C for 40 s followed by 72 °C for 10 min to ensure complete extension for all PCR reactions.
Generation of transgenic plants with the synthesized CfNFSBI gene
The synthesized CfNFSBI gene was transformed into A. thaliana (ecotype Columbia) plants with the hygromycin B phosphotransferase gene as the genetic selection marker. The CfNFSBI gene cassette containing the double CaMV 35S promoter and Nos-Terminator in its downstream sequence was flanked by two tobacco scaffold attachment regions. A. thaliana was transformed by the floral dip method [Citation22]. Plants were grown on Murashige and Skoog medium for 15 d, and transferred into the pots filled with a 9:3:1 mixture of vermiculite/peat moss/perlite in a controlled environmental chamber at 22 °C under a 16 h/8 h (light/dark) photoperiod at a daylight intensity of 100 E m2− s−1. Ten transgenic plants (T1) were identified by PCR from 15 putative transgenic plants regenerated on half-strength MS agar plates containing hygromycin B gene. Both transgenic plants and wild-type plants were identical in phenotype in soil or on half-strength MS agar plates. This indicates that the insertion of the CfNFSBI gene in these plants produced no visible morphological changes.
Total RNA extraction and reverse transcription-PCR analysis
Total RNA was extracted from transgenic and wild-type seedlings with the multisource total RNA miniprep kit (Axygen, Union City, CA, USA) according to the manufacturer’s instructions and treated with DNase I (Promega, Madison, WI, USA) for removing genomic DNA. The first strand of cDNA was synthesized using 5 μg of total RNA as a template with the Reverse Transcription System (Promega) in a 20 μL reaction volume. The fragment of CfNFSBI gene was amplified by PCR using a forward primer 5′-AAGGAACTGAAAGATGACAAC-3′ and a reverse primer 5′-CGTCTTCCACTGGAGACTACT-3′ according to the sequence of the CfNFSBI gene. A 250 bp fragment of the A. thaliana actin gene (AtAc2, accession number NM112764) was amplified by two primers (AtAc2Z1: 5′-GCA CCC TGT TCT TCT TAC CGA G-3′; AtAc2F1: 5′-AGT AAG GTC ACG TCC AGC AAG G-3′) served as an internal standard gene. All RT-PCR reactions were carried out in 25 cycles lasting 30 s at 94 °C, 30 s at 54 °C, and 30 s at 72 °C and with a final extension at 72 °C for 10 min (Applied Biosystems 2720 PCR instrument). The PCR products were separated in a 2% agarose gel and quantified with a Model Gel Doc 1000 (Bio-Rad, USA). The DNA intensity ratio of the CfNFSBI gene to AtAc2 was analyzed with ShineTech Gel Analyser (Shanghai Shine Science of Technology Co., Ltd, China) to evaluate the expression pattern of the CfNFSBI gene.
The effect of TNT on the A. thaliana growth and photosynthesis
For plant growth assay, seeds of wild-type A. thaliana and three T2 homozygous CfNFSBI transgenic lines (BH216-3, 5 and 6) were grown vertically on MS medium containing 0, 25, and 50 μmol L−1 TNT predissolved in acetone for 2 weeks. The root lengths of all seedlings were measured. Meanwhile, transgenic lines and wild-type seeds were planted to MS medium with different concentrations TNT (0, 50, 100 and 150 μmol L−1) for horizontal growth. The survival rate was determined and photographed after 2 weeks.
On the other hand, other wild-type and transgenic seeds were surface-sterilized and germinated in MS medium and grown for 10 days, then the seedlings were transferred to sterile flasks containing 15 ml MS liquid medium for 48 h in order to recover and then transferred aseptically to 15 ml conical flasks containing 0, 50, 100 and 150 μmol L−1 TNT in sterile MS liquid medium. Flasks were incubated in the light at 23 °C with rotary shaking. The wet weight of seedlings was then determined after a further 2 weeks of growth. To test the effect of TNT on photosynthesis, 4-week-old plants grown in soil were soaked with 0, 250, 500 and 750 μmol L−1 TNT for a week. Three-five leaves in the Columbia ecotype were used for photosynthesis analysis. Chlorophyll and Fv/Fm was determined as our previous report [Citation23].
Preparation of protein extract and nitroreductase activity
Wild-type and transgenic lines were ground in liquid nitrogen in a mortar with pestle and homogenized with pre-cooled 20 mmol L−1 Tris-HCl (pH 7.0) and incubated on ice for 30 min. The homogenate was centrifuged at 12,000 rpm (Eppendorf 5418 centrifuge, Shanghai aiyan Biotechnology Co., Ltd, China) (4 °C) and the supernatant was used as crude extract. Total protein content was determined by using the Bradford assay [Citation24]. The nitroreductase activity was measured by the method of Villanueva [Citation25].
Rate constant of TNT disappearance in plants
Samples of the seedlings in MS liquid medium described above were taken every 24 h for 144 h. The details of reverse-phase high-performance liquid chromatography (HPLC) performed in this study is consistent with our previously published paper [Citation26]. We calculated the kinetics of TNT disappearance described by Pavlostathis et al. [Citation27].
Data analysis
Data are mean values from at least three independent experiments performed in triplicates. Statistical analysis was performed by two-way analysis of variance (ANOVA) with Tukey’s multiple comparisons test. Differences were considered statistically significant at the p ≤ 0.05 level.
Results
Stable expression of the CfNFSBI gene and enzyme activity in transgenic plant
The expression levels of the CfNFSBI gene were identified from transgenic plants by RT-PCR. The specific DNA band of 250 bp was detected from three lines, whereas no such signals were detected in wild-type plants (). With the AtAc2 gene as a reference, relatively higher transcript levels were detected in BH216-5 and BH216-6 compared with that in BH216-3. The quantity RT-PCR analysis of RNA also revealed that CfNFSBI was expressed in all of the three transgenic lines, with the different transcript levels in transgenic plants, the highest for BH216-6, then for BH216-5 and the lowest for BH216-3 (). Nitroreductase activities of wild-type and transgenic lines in crude extracts were determined. The amount of the enzyme activities in all three transgenic lines showed significantly higher nitroreductase activities than wild-type plants. Moreover, the amount of the enzyme activities in all three transgenic lines were correlated to the transcript levels of the CfNFSBI gene ().
Figure 1. PCR analysis of the CfNFSBI gene fragment and enhanced nitroreductase activity. (a) RT-PCR analysis of the CfNFSBI gene fragment, WT serves as wild-type plant; wild-type and BH216 lines (BH216-3,-5,-6) with actin gene as a reference. (b) Relative transcript levels analyses of different CfNFSBI transgenic Arabidopsis lines. (c) Nitroreductase activity of wild-type and transgenic plants.
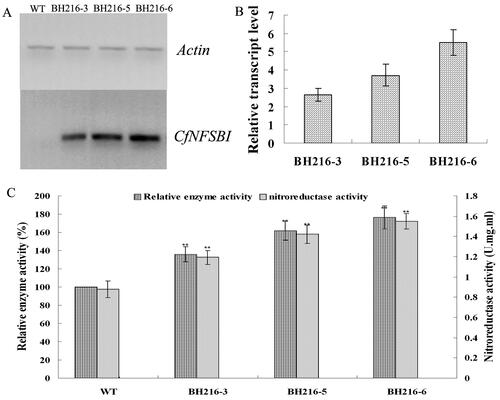
Morphological responses of transgenic lines to TNT
To test the effect of TNT on transgenic lines, firstly, we investigated the seedlings growth in vertical petri dishes. TNT phytotoxic effects were estimated by plants color, leaf loss and lack of new growth. The symptom of TNT phytotoxic on wild-type and transgenic plants seriously as TNT concentration increased. All seedlings containing TNT showed inhibition of root elongation compared with MS solid medium (). However, the decrease of root length in the transgenic plants was significantly lower than that in wild-type plants (). Especially for BH216-6, the root lengths were about 3-fold longer than those in wild-type plants at 25 and 50 μmol L−1 TNT.
Figure 2. Morphological responses of wild-type and transgenic plants under different concentrations of TNT. (a) Wild-type (WT) and BH216 plants germinated and grown vertically for 2 weeks on half-strength MS agar plates containing 0, 25 and 50 μmol L−1 TNT. (b) Root length of 2-week-old WT and BH216 plants grown on half-strength MS agar plates containing 0, 25 and 50 μmol L−1 TNT. The data represent mean values ± SD (n = 10).
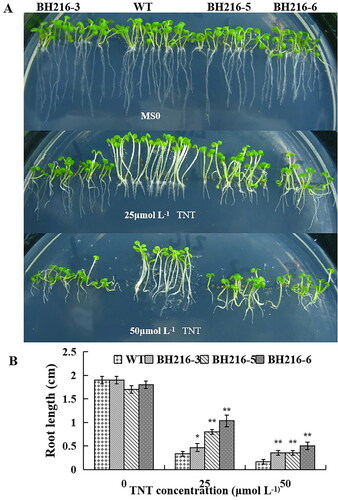
We next investigated the responses of wild-type and transgenic seedlings to TNT stress in horizontal petri dishes. The survival rate was determined after seedlings growth for 2 weeks (). The seeds of transgenic and wild-type plants grew well with normal morphology on plates without TNT. Many plants including wild-type and transgenic lines at 50 μmol L−1 TNT concentration remained green and healthy after 2 weeks (). All seedlings exhibited stress symptoms including inhibition of germination, leaf loss and color loss at higher concentrations of TNT (100 and 150 μmol L−1). However, the wild-type seedlings were suppressed to a greater extent, for example, the seedlings turned yellow or brown, the leaves grew smaller and there was lack of new growth. Almost all of the wild-type seedlings were killed at 150 μmol L−1 TNT and the survival rate was zero. On the contrary, many seedlings of the transgenic lines were green and more than 40% seedlings were survived ().
Enhanced tolerance of transgenic lines to TNT
An experiment was conducted to assess the toxic effect of TNT on wild-type and transgenic seedlings. The wild-type and BH216-6 seedlings were transferred to the liquid MS medium for 48 h before the addition of TNT. Without TNT, the seedlings growth appeared healthy (). The relative fresh weight for the wild-type was larger than BH216-6 line plants. At 50 μmol L−1 TNT concentration, the transgenic BH216-6 plants showed no phytotoxic effects and the increased growth biomass was 2 times than that of the wild-type plants (). However, all wild-type plants at the higher concentrations of TNT (100 and 150 μmol L−1) showed chlorosis after incubation, with the leaves being yellow, the roots shorter and the biomass less. But the majority of leaves were green for BH216-6 plants and the degree of damage degree was lower than that in wild-type plants.
Figure 4. Growth of wild-type and transgenic line in liquid medium. (a) Thirty seedlings grown in MS solid medium for 2 weeks were transferred to MS liquid medium in flasks containing 0, 50, 100 and 150 μmol L−1 TNT. (b) Increased fresh weights of seedlings in the individual flasks in the above treatment. The data represent mean values ± SD (n = 3).
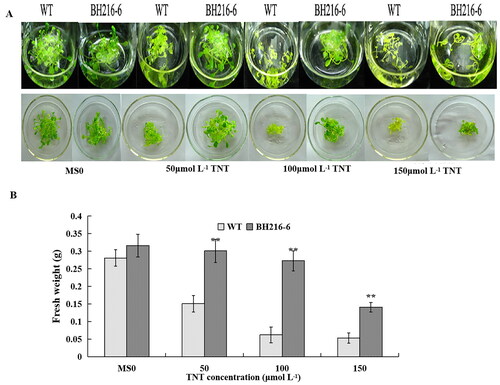
Moreover, transgenic plants were resistant to chlorophyll degradation and photosynthesis inactivation. After soaking 4-week-old plants with different concentrations of TNT (0, 250, 500, 750 μmol L−1) for a week, there was significant difference in either the chlorophyll contents or the Fv/Fm parameter among the wild-type and transgenic lines. The total chlorophyll contents decreased in the wild-type and all of the transgenic lines, but the chlorophyll reduction in wild-type plants was significantly more pronounced than the reduction in the transgenic plants at all the TNT treatment (). The Fv/Fm value decreased as a consequence of accumulated photooxidative damage to PSII. It was higher in all of the transgenic plants than in the wild-type plants (). This is consistent with the view that plants overexpressing the CfNFSBI gene are more resistant to photo inhibition than wild-type plants.
Figure 5. Effect of TNT treatment on photosynthetic activities. (a) Chlorophyll (Chl) changes in wild-type and transformed plants. (b) Fv/Fm changes in wild-type and transformed plants. Wild-type and transformed plants grown in soil for 4-week-old and then soaked with 0, 250, 500 and 750 μmol L−1 TNT for a week. The data represent mean values ± SD (n = 3).
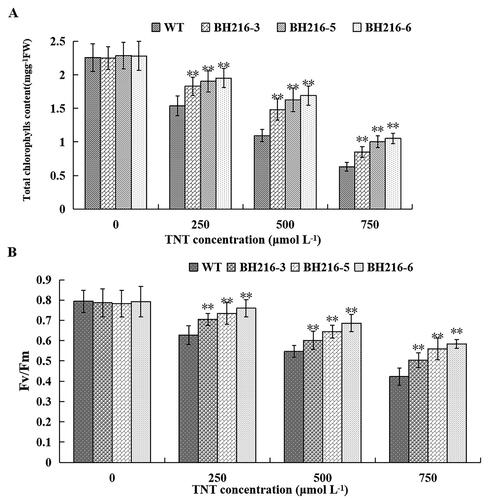
Rate constant of TNT disappearance in plant and extent of TNT removal
The concentration of TNT was measured by HPLC, the method was the same as that in our previous paper [Citation23]. Both wild-type and transgenic plants were able to remove TNT from the liquid medium. However, almost all of the TNT was removed within 96, 120 and 144 h at 50, 100 and 150 μmol L−1 TNT for all the transgenic plants except for BH216-3 (). At 50 μmol L−1, the wild-type plants removed 71% of TNT within 96 h; however, 57% to 61% TNT was removed within the first 24 h. In incubations with 100 and 150 μmol L−1 TNT, wild-type plants removed only 32% and 27% TNT within 24 h, the transgenic plants removed about 55% and 50% TNT within 24 h. At 100 and 150 μmol L−1, no TNT could be detected in the transgenic plants within 120 and 144 h except for BH216-3, for which 14.90 μmol L−1 TNT remained at 144 h at 150 μmol L−1. However, wild-type plants did not have this characteristic during the incubation period.
Discussion
In our previous study [Citation28], a system of phytoremediation by Arabidopsis plants was developed on the basis of overexpression of triphenylmethane reductase (TMR) from Citrobacter sp. The results showed that the TMR transgenic Arabidopsis plants showed significantly enhanced tolerances to crystal violet (CV) and malachite green (MG). In this study, we successfully introduced CfNFSBSI genes of the NAD(P)H-flavin nitroreductase from Citrobacter freundii into Arabidopsis plants. In vitro assays also showed that the crude protein produced by the transgenic plants can catalyze TNT degradation.
The root elongation (), survival rate of seedlings (), leaf color and size and fresh weight () were inhibited after TNT treatment for all plants, however, the transgenic plants showed higher tolerance to TNT than wild-type plants. In any potential phytoremediation application, normal root formation is essential [Citation29]. There was no notable difference in the root length between wild-type and transgenic plants at MS medium (). At 25 μmol L−1 TNT, the wild-type seedlings exhibited TNT phytotoxic effects in shorter root length and extensive root hair growth, whereas the transgenic lines showed negligible phytotoxic effects with longer root length. TNT and its transformation products are transported from the plant roots to the plant stem and leaves where they are further transformed to more polar metabolites [Citation27]. In 1997, Vanderford et al. demonstrated that dissolved TNT most likely enters the roots and then passes through the vascular elements to the stem and then into the leaf [Citation30]. TNT transformation products are usually deposited in vacuoles and cell walls of plants. In agreement with this, our experiment also showed that the root elongation was the most inhibited indicator of TNT phytotoxic effects in wild-type and transgenic plants. Based on the results of this experiment, the transgenic plants showed higher TNT tolerance than the wild-type plants.
In our experiment, the kinetics of TNT removal was determined at 50, 100 and 150 μmol L−1 TNT in wild-type and transgenic plants. All plant samples showed a decrease in TNT concentration over the incubation period. Almost all of the TNT were removed within 96, 120 and 144 h at 50, 100 and 150 μmol L−1 TNT for all the transgenic plants (except for BH216-3 at 144 h). On the contrary, the wild-type plants removed a limited amount of TNT from both the liquid medium and the plant tissues. Therefore, the lower TNT removal rates associated with the higher initial TNT concentrations may be attributed to phytotoxic effects of TNT and/or transformation products.
Conclusions
This study demonstrated that transgenic plants that overexpressed nitroreductase gene from the Citrobacter freundii have an intrinsic capacity to remove TNT and reduce its phytotoxic effects. Further studies on the nitroreductases system participating in the degradation of TNT from both the metabolic and structural levels can be applied for discovering more catalysts with desired activities.
Data availability
The data supporting the findings of this study are available from the authors upon reasonable request.
Disclosure statement
All authors declare that they have no conflict of interest; they are entitled to the authorship and have approved the final version of the manuscript.
Additional information
Funding
References
- Liao HY, Chien CC, Tang P, et al. The integrated analysis of transcriptome and proteome for exploring the biodegradation mechanism of 2, 4, 6-trinitrotoluene by Citrobacter sp. J Hazard Mater. 2018;349:79–90.
- Arora PK. Bacilli-mediated degradation of xenobiotic compounds and heavy metals. Front Bioeng Biotechnol. 2020;8:570307–570335.
- Yang X, Zhang Y, Lai JL, et al. Analysis of the biodegradation and phytotoxicity mechanism of TNT, RDX, HMX in alfalfa (Medicago sativa). Chemosphere. 2021;281:130842.
- Alothman ZA, Bahkali AH, Elgorban AM, et al. Bioremediation of explosive TNT by Trichoderma viride. Molecules. 2020;25(6):1393–1406.
- Esteve-Núñez A, Caballero A, Ramos JL. Biological degradation of 2,4,6-trinitrotoluene. Microbiol Mol Biol Rev. 2001;65(3):335–352.
- Ramos JL, Caballero A, Duque E, et al. Physiological evidence for respiration of TNT by Pseudomonas sp. JLR11. In: Ramos JL, editor. Pseudomonas. 3rd ed. London: Kluwer Academic/Plenum Publisher; 2004. p. 229–240.
- Islam MN, Jung HY, Park JH. Subcritical water treatment of explosive and heavy metals co-contaminated soil: removal of the explosive, and immobilization and risk assessment of heavy metals. J Environ Manage. 2015;163:262–269.
- Vanek T, Nepovim A, Podlipna R, et al. Phytoremediation of selected explosives. Water Air Soil Pollut: Focus. 2003;3:259–267.
- Vanek T, Gerth A, Vavrikova Z, et al. Phytoremediation of explosives. In: Marmiroli N, Samotokin B, Marmiroli M, editors. Advanced science and technology for biological decontamination of sites affected by chemical and radiological nuclear agents, NATO science series, series IV: earth and environmental sciences. Vol. 75. Dordrecht: Springer; 2007. p. 209–225.
- Panz K, Miksch K. Phytoremediation of explosives (TNT, RDX, HMX) by wild-type and transgenic plants. J Environ Manage. 2012;113:85–92.
- You SH, Zhu B, Han HJ, et al. Phytoremediation of 2,4,6-trinitrotoluene by arabidopsis plants expressing a NAD(P)H-avin nitroreductase from Enterobactercloacae. Plant Biotechnol Rep. 2015;9(6):417–430.
- Rylott EL. Endophyte consortia for xenobiotic phytoremediation: the root to success? Plant Soil. 2014;385(1–2):389–394.
- Zhang L, Rylott EL, Bruce NC, et al. Genetic modification of Western wheatgrass (Pascopyrum smithii) for the phytoremediation of RDX and TNT. Planta. 2019;249(4):1007–1015.
- Khan MI, Lee J, Park J. A toxicological review on potential microbial degradation intermediates of 2,4,6-trinitrotoluene, and its implications in bioremediation. KSCE J Civ Eng. 2013;17(6):1223–1231.
- Gunning V, Tzafestas K, Sparrow H, et al. Arabidopsis glutathione transferases U24 and U25 exhibit a range of detoxification activities with the environmental pollutant and explosive, 2,4,6-trinitrotoluene. Plant Physiol. 2014;165(2):854–865.
- Tzafestas K, Ahmad L, Dani MP, et al. Structure-guided mechanisms behind the metabolism of 2,4,6-trinitrotoluene by glutathione transferases U25 and U24 that lead to alternate product distribution. Front Plant Sci. 2018; 9:01846.
- Johnston EJ, Rylott EL, Beynon E, et al. Monodehydroascorbate reductase mediates TNT toxicity in plants. Science. 2015;349(6252):1072–1075.
- Zhang L, Routsong R, Nguyen Q, et al. Expression in grasses of multiple transgenes for degradation of munitions compounds on live-fire training ranges. Plant Biotechnol J. 2017;15(5):624–633.
- Zhang L, Rylott EL, Bruce NC, et al. Phytodetoxification of TNT by transplastomic tobacco (Nicotiana tabacum) expressing a bacterial nitroreductase. Plant Mol Biol. 2017;95(1–2):99–109.
- Peng RH, Xiong AS, Yao QH. A direct and efficient PAGE-mediated overlap extension PCR method for gene multiple-site mutagenesis. Appl Microbiol Biotechnol. 2006;73(1):234–240.
- Xiong AS, Yao QH, Peng RH, et al. PCR-based accurate synthesis of long DNA sequences. Nat Protoc. 2006;1(2):791–797.
- Zhang X, Henriques R, Lin SS, et al. Agrobacterium-mediated transformation of Arabidopsis thaliana using the floral dip method. Nat Protoc. 2006;1(2):641–646.
- Zhu B, Han HJ, Fu XY, et al. Degradation of trinitrotoluene by transgenic nitroreductase in arabidopsis plants. Plant Soil Environ. 2018;64(8):379–385.
- Bradford MM. A rapid and sensitive method for the quantization of microgram quantities of protein utilizing the principle of protein-dye binding. Anal Biochem. 1976;72(1–2):248–254.
- Villanueva JR. Nitro-reductase from a Nocardia sp. Antonie Van Leeuwenhoek. 1964;30:17–32.
- Zhu B, Peng RH, Fu XY, et al. Enhanced transformation of TNT by arabidopsis plants expressing an old yellow enzyme. PLoS One. 2012; 7(7):e39861.
- Pavlostathis SG, Comstock KK, Jacobson ME, et al. Transformation of 2,4,6-trinitrotoluene by the aquatic plant Myriophyllum spicatum. Environ Toxicol Chem. 1998;17(11):2266–2273.
- Fu XY, Zhao W, Xiong AS, et al. Phytoremediation of triphenylmethane dyes by overexpressing a Citrobacter sp. triphenylmethane reductase in transgenic Arabidopsis. Appl Microbiol Biotechnol. 2013;97(4):1799–1806.
- Hannink N, Rosser S, French C, et al. Phytodetoxification of TNT by transgenic plants expressing a bacterial nitroreductase. Nat Biotechnol. 2001;19(12):1168–1172.
- Vanderford M, Shanks JV, Hughes JB. Phytotransformation of trinitrotoluene (TNT) and distribution of metabolic products in Myriophyllum aquaticum. Biotechnol Lett. 1997;19(3):277–280.