Abstract
Wastewaters from the industrial sector and, in particular, those from the textile industry have a diverse composition of organic dyes. These dyes are xenobiotics for living organisms and, falling into natural water, can lead to severe consequences for aquatic flora and fauna. This is the case with the azo-dyes, such as, for example, the amaranth. The adaptive responses of the micro- and metafauna communities in activated sludge towards shock loading of high concentrations of the azo-dyes in wastewater treatment plants (WWTPs) have been poorly studied. The aim of this research was to study the adaptation mechanisms of the communities of micro- and metafauna in activated sludge towards the shock loading of amaranth (200 mg/L)with and without the addition of Pseudomonas aureofaciens AP-9 as a bioaugmentation agent (3.03 × 107 cells/mL). To achieve this, the change in the quantities of key groups of micro- and metafauna was monitored in the course of a model biodegradation process involving real activated sludge from a WWTP and toxic pollutant amaranth at a concentration of 200 mg/L. The results showed that in the case of such shock loading with amaranth, the microfauna communities changed very quickly. In addition, a positive bioaugmentation effect of the added strain of Pseudomonas aureofaciens AP-9 was observed for the micro- and metafauna. The bacteria in a homogenous form provide easily accessible food for the macro-organisms and contribute to their adaptation in the conditions of a toxic shock. This is particularly pronounced in the representatives of free-swimming ciliates and small flagellates.
Introduction
A big challenge in biological wastewater treatment is the simultaneous loading of domestic and industrial wastewater in wastewater treatment plants (WWTPs). Thus, the industrial wastewater introduces high concentrations of xenobiotics in the WWTPs. Such xenobiotics are also the dyes used in the textile industry.
Azo-dyes are synthetic organic compounds that are characterized by the presence of one or more N = N groups bonded to an aromatic residue. During the oxidative processes, some azo-dyes generate intermediate metabolites, such as aromatic amines, which are more toxic than the initial compound [Citation1]. Azo-dyes are used in a vast circle of industrial activities such as the production and the processing of leather, textile, cosmetics, medicines, paper and cellulose [Citation2,Citation3]. They are not synthesized in the nature, and because of that azo-dyes are considered xenobiotics. One of the most serious environmental risks related to their wide use is the entry of high concentrations of azo-dyes in the WWTP, which leads to damage of the activated sludge structure and functions [Citation4]. Azo-dyes are the most widely used dyes and represent around 70% of the ones used in the textile industry. They are part of the xenobiotic compounds that are hardly amenable to biodegradation [Citation5–7]. In the wastewater from the textile industry, the concentrations of azo-dyes may vary from 5 to 1500 mg/L [Citation8]. The high toxicity of these dyes has attracted the attention of researchers seeking approaches for their detoxification or effective biodegradation [Citation9,Citation10]. Another aspect of their negative impact is that after their discharge into the water bodies, they impede the light penetration and the oxygen circulation in the water basins. Therefore, the presence of these pollutants impacts the whole aquatic life. These are the reasons why the elimination of dyes is one of the primary concerns in the treatment of wastewater from the textile industry [Citation11].
The model azo-dye used in our experiment is amaranth (Е123), which is a sulphonic acid-based naphthylazo dye that is usually used for adding a red to violet colour of food, cosmetics and medicines [Citation11,Citation12]. The dye is classified as an endocrine disruptor and has a negative impact on the human and animal health [Citation13,Citation14].
The production and use of amaranth and other synthetic dyes has increased significantly in the last decades. This has entailed concomitant formation of industrial wastewater polluted with these compounds. The elaboration of effective methods for the treatment of wastewater containing dyes is an important challenge for the water treatment technologies. Finding a solution to this problem will lead to improved environment protection from the unfavourable impacts of these pollutants [Citation15]. An important approach in the treatment of wastewater from the textile industry is activated sludge. It is a community composed of three key groups of organisms: bacteria, micro- and metafauna [Citation16–18]. Although in most cases the bacteria in the activated sludge can adapt to a volley loading of xenobiotics, especially azo-dyes, due to their enzymatic apparatus, part of the organisms of the micro- and metafauna are quickly inhibited and die [Citation19]. This damages the activated sludge because, although the fauna is not directly committed to the biodegradation of the pollutants in the wastewater, it has a key significance for the sustainment of the bacteria segment, contributes to the flocculation and to a certain extent stimulates the bacterial activity [Citation20–22].
There are limited studies on the potential of the macro-organisms in the activated sludge of WWTPs to adapt to high concentrations of dyes. Kozuharov et al. reported that the values of the indexes used for micro- and metafauna assessment indicate that the constant exposure to high concentrations of azo-dyes has a negative impact on the macro-organisms in the activated sludge [Citation23]. Some of the representatives of the micro- and metafauna, such as Habrotrocha sp. and Opercularia sp., succeed to adapt and are found in activated sludge treating wastewater from the textile industry [Citation24]. The representatives of the micro- and metafauna are very sensitive organisms and are important indicators of the treatment effectiveness in the synthetic textile industry [Citation25]. The ciliated organisms in the activated sludge, such as Opercularia sp., Epistylis sp., Didinium sp. and Chilodonella sp., are also registered in high quantity as organisms that could withstand and adapt to the highly toxic conditions of wastewater containing dyes [Citation25]. An experiment for the sustainability of free-living amoeba with organic dyes (azo and anthraquinone) found that genus Acanthamoeba sp. was highly represented [Citation26]. Another study indicated that the toxicity of the azo-dyes to the macro-organisms limited the usefulness of Sludge Biotic Index in the treatment of wastewater from the textile industry [Citation27].
An emerging technique to improve the catabolism of specific pollutants, such as xenobiotics, is bioaugmentation through the addition of pure strains and cultures of bacteria to the bioreactors for wastewater treatment [Citation28]. Bioaugmentation is one of the main approaches for the management of the treatment technologies toward the achievement of higher effectiveness [Citation18,Citation29,Citation30]. A growing body of evidence suggests that bioaugmentation with pure cultures does not perform as well in the treatment plants as it does in lab conditions [Citation28]. A similar paradox occurred in the studies of Topalova et al. in which the addition of a pure culture of Pseudomonas aurefaciens AP-9 did not lead to a significant increase in the detoxification of pentachlorophenol [Citation18]. The explanation lies in the communities of the micro- and metafauna. The addition of fresh biomass of pseudomonads provides the fauna with an easily accessible food source containing a high percent of detoxifying enzymes. These enzymes support the survival and the functioning of the microorganisms [Citation31]. This decreases the toxic shock over the fauna and, although the bioaugmentation does not have a significant effect on the biodegradation, it improves the quality of the activated sludge [Citation18].
The aim of this research was to study the adaptation mechanisms of the communities of the micro- and metafauna in activated sludge in the shock loading of amaranth with and without the addition of Pseudomonas aureofaciens AP-9 as a bioaugmentation agent. To achieve this, the change in the quantities of individual groups of ciliates, amoebae, flagellates, rotifers and nematodes was monitored in the course of a model biodegradation process involving real activated sludge from WWTP ‘Kubratovo’ (Bulgaria) and a toxic pollutant amaranth at a concentration of 200 mg/L.
Materials and methods
Experimental design
We used two model bioreactors (): Bioreactor 1 (Variant I) includes 900 mL activated sludge (AS) of WWTP ‘Kubratovo’ + 3% nutrient solution + 200 mg/L Amaranth. Bioreactor 2 (Variant II) includes 900 mL AS of WWTP ‘Kubratovo’ + 3% nutrient solution + 200 mg/L Amaranth + Pseudomonas aureofaciens AP-9. In both bioreactors, we used activated sludge from WWTP ‘Kubratovo’ (Bulgaria) and model wastewater. The volume of the wastewater was 100 mL and the activated sludge was 3 g/L as dry weight (as in the WWTP ‘Kubratovo’). The mode of operation is batch process in anoxy conditions. The added nutrient solution contains readily degradable organic matter to provide sufficient reducing equivalents to break the azo bond. Samples and measurements were taken at the following critical control points: 0 h, 24 h, 48 h, 72 h, 96 h, 12 h, 144 h and 168 h. They were selected to study the different phases of the detoxifying process and the adaptation of the communities of the micro- and metafauna. The early phase of the model process occurs from 0 h to 48 h, when the decolorization of the azo-dye is pending. After this moment the process enters the following phase of initial toxicity.
Model wastewater
The model wastewater had the following composition: Salt solution (NaH2PO4 − 3.5 g/L, K2HPO4 − 5.0 g/L, (NH4)2SO4 − 2.5 g/L, MgSO4.7H2O − 0.3 g/L, FeSO4 − 0.05 mg/L, CuSO4 − 0.01 mg/L, ZnSO4 − 0.005 mg/L, CoCl2 − 0.005 mg/L, MgCl2 − 0.005 mg/L, CaCl2 − 0.005 mg/L, Na2MoO4 − 0.005 mg/L), 3% nutrient solution (NaCl − 5 g/L; peptone − 10 g/L; yeast extract − 5 g/L) and Amaranth (200 mg/L) [Citation32]. The model xenobiotic was supplied by Sigma Aldrich.
Activated sludge
Activated sludge (AS) was obtained from WWTP ‘Kubratovo’ from a bio-basin working in a denitrification mode. It was not additionally treated.
Added bacterial culture
The bacterial cell culture of Pseudomonas aureufaciensAP-9 added in Variant II was selected on the basis of its biodegrading potential in the Laboratory of ‘Biological Water Treatment and Environmental Biotechnology’ at the Faculty of Biology (Sofia University, Bulgaria). It was added to the model bioreactors in the form of an 18-hour culture, cultured on nutrient agar and washed with 0.9% solution of NaCl. It was added as 10% v/v as microbial suspension with a density of 3.03 × 107 cells/mL.
Chemical analysis
Amaranth concentration was determined spectrophotometrically (Utrospec3000, PharmaciaBiotech), λ = 520 nm [Citation33]. The residual amount of amaranth in the water phase was determined in the course of the process.
Hydrobiological analyses
Permanent microscopic slides were prepared for microscopic analysis of the activated sludge. Samples from the AS were well homogenized. Using an automatic pipette, 50 µL of sample from the bio-basins was pipetted onto a glass slide; then each sample was fixed and sealed with a cover glass. To determine the organisms of the micro- and metafauna we used the guides of Sladka and Sladeček [Citation34], Foissner & Berger [Citation35] and Gerardi [Citation36]. To determine the biotic index of the activated sludge according to Madoni [Citation37], we observed the whole surface of the slide with a magnification of 200X. The number of the observed organisms from each taxonomic group in 50 µL of sample was counted and recorded and the flocs structure was determined [Citation36,Citation37]. Based on the quantity of the organisms per litre, the total number of taxa, the dominant key group and the number of small flagellates were determined; using Madoni’s table, the biotic index of the activated sludge was determined [Citation37].
Microbiological analysis
The microbial counts were determined through cultivation on solid growth media according to the conventional microbiological practice [Citation38]. Physiological and taxonomic groups of bacteria were determined, like aerobic heterotrophs (AeH), bacteria from the genus Pseudomonas (Ps). The azo-degraders (Azo-degr.) were grown on Nutrient Agar with 200 mg/L Amaranth [Citation32]. The aerobic heterotrophs were grown on Nutrient Agar and bacteria from genus Pseudomonas were grown on Glutamate Starch Pseudomonas Agar [Citation38].
Data analysis
Data are presented as mean values from 3 experiments with standard deviation (±SD). Statistical analysis was performed using t-test in Sigma Plot, version 12.5. Differences were considered statistically significant at the p < 0.05 level.
Results
Residual amount of amaranth
First, we analyzed the dynamics of the residual amount of amaranth in the water phase. By means of calculations we determined the biodetoxifying effect and the effect of the added pseudomonads in the course of the process.
The residual amount of amaranth decreased and was eliminated at the 48th hour (). The difference in the elimination/decolorization rate became visible as early as 8 h; with the added culture of pseudomonads the amount of amaranth was 132.45 mg/L (Variant II), whereas in the control variant it was 148.60 mg/L (Variant I). At 24 h, in variant II the amount of amaranth was 60.46 mg/L, whereas in variant I it was 72.23 mg/L (p = 0.907). At 32 h again the amaranth elimination was higher in the variant with Pseudomonas aureofaciens AP-9. After 48 h, the amount of amaranth in the water phase remained nearly zero.
Figure 2. Residual amount of amaranth in both studied variants of the experiment, where Variant I is control, and Variant II with microbial augmentation (Pseudomonas aureofaciens AP-9).
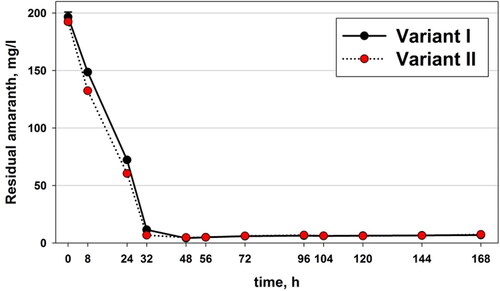
In the next steps of our study, we determined the representatives of the micro- and metafauna to analyze whether they also had indirect relation to the amaranth elimination.
Sludge biotic index
The biotic index of the AS decreased during the studied process in both variants (). At 0 and 24 h, the biotic index reached a peak with a maximal value of 10 in both variants. At 48 h, when total amaranth elimination occurred, the values of the index sharply decreased. In variant I, the index had a value of 8, whereas in variant II it was 4. This two-fold decrease in the index was associated with changes in the microfauna in the activated sludge: part of the key groups disappeared and the dominant groups changed. However, the decrease in the biotic index values was not related to a decrease in the number of the organisms. On the contrary, the number of organisms from the micro- and metafauna at 4h h was higher than in the control variant (). At 72 h the values of the biotic index in both variants both reached a value of 4. In the following hours, the values decrease still further. Only at 168 h the index increased in variant II due to the dominant development of free-swimming ciliates.
Figure 3. Sludge biotic index (SBI) in the two variants of the experiments: Variant I (without P. aureofaciens AP-9) and Variant II (with P. aureofaciens AP-9) [Citation37].
![Figure 3. Sludge biotic index (SBI) in the two variants of the experiments: Variant I (without P. aureofaciens AP-9) and Variant II (with P. aureofaciens AP-9) [Citation37].](/cms/asset/1df186ea-5566-4b4a-bf2e-a36c16df4dea/tbeq_a_2070437_f0003_c.jpg)
Figure 4. Changes in the quantity of the micro- and metafauna and the residual amount of amaranth in Variant I (without P. aureofaciens AP-9) and Variant II (with P. aureofaciens AP-9).
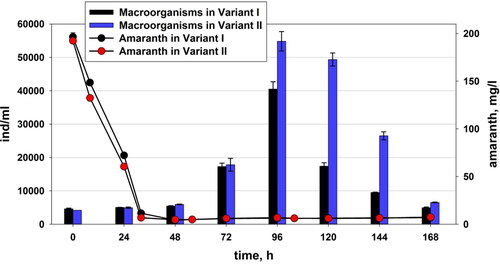
Changes in the number of the micro- and metafauna
The number of organisms from the micro- and metafauna during the first hours − 0 and 24, were more in variant I than in variant II (). At the 48th hour there was a change and the number of the organisms in variant II became larger. This trend continued to the end of the study. There was a very sharp increase in the total number of macro-organisms in the activated sludge at the 96th and 120th hour in both variants. After the 120th hour the number of the representatives of the micro- and metafauna started to decrease significantly in both variants, but their number still remained higher in variant II.
Changes in the as macrostructure
The microscopic analysis registered a decrease in the size of the flocs during the process of amaranth elimination in both variants (). At the 72nd hour of the experiment, there were smaller but thicker flocs. The reduced size of the flocs was due to the toxic shock and led to an increase in the number of the homogenous bacterial cells, which is of vital significance for the adaptation of the micro- and metafauna. These free-swimming bacterial cells are very easily accessible food for the macro-organisms in AS. To a great extent, these bacteria help the macro-organisms to survive the unfavourable conditions created by the shocking influx of the xenobiotic in the system.
Changes in the number of naked and testate amoeba
In both variants, there was a comparatively stable number of naked and testate amoeba (). There was a decrease in both variants at the 72nd and the 96th hour, and after that a sharp increase in the number of both key groups of the micro-fauna. These two groups stayed comparatively unaffected by the shocking amaranth loading. In these unfavourable conditions, they succeeded to adapt, and after the 96th hour their number increased.
Changes in the number of flagellates
Flagellates had a dominant role after the 48th hour in both variants (). This is a clear indicator of damage of the AS due to the toxic shock. There were much larger quantities of small flagellates in the variant with added pseudomonads (Variant II). Their number was especially higher at the 96th hour, when they reached up to 46 300 ind/L and 41 900 ind/L at the 120th hour. At the 168th hour, a decrease in the number of small flagellates was observed in both variants.
Changes in the number of ciliates
In the control variant up to the 48th hour, the crawling and attached ciliates were dominant (). After the 48th hour, the number of these two groups decreased, and the free-swimming ciliates became dominant. In the variant with the added pseudomonads (Variant II), the crawling and attached ciliates were dominant only at the beginning of the experiment. From the 24th hour to the end of the studied process, the free-swimming ciliates were dominant. In Variant II, there was an increase in the number of swimming ciliates, with peak values at the 168th hour. In Variant I, the swimming cilates reached a maximum at the 120th hour. The crawling and attached ciliates, which are positive indicators for the functioning of the AS, almost completely disappeared after the 96th hour.
Figure 8. Changes in the quantity of the ciliates in: (a) Variant I (without P. aureofaciens AP-9) and (b) Variant II (with P. aureofaciens AP-9).
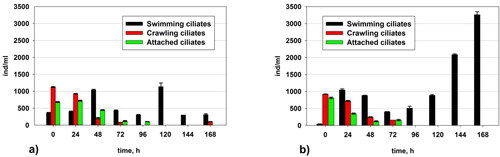
Тhe images in show a free-swimming ciliate that has absorbed the red dye amaranth. The images showed three different organisms. On microscopic examination, red dye was found in the cells of many swimming ciliates.
Changes in the number of suctorians, rotifers and nematodes
presents the data about the representatives of the metafauna. Their number was larger in the control variant but after the 72nd hour they completely disappeared due to the toxic shock of the amaranth loading in the system. In variant I the number of the rotifers was higher at the 48th hour when they reached up to 160 ind./L. In variant II the organisms of the metafauna were found up to the 120th hour through some fluctuations.
Dynamics of the quantity of aerobic heterotrophs, bacteria of genus Pseudomonas and azo-degrading bacteria
shows the number of aerobic heterotrophs, bacteria of genus Pseudomonas and azo-degrading bacteria in comparison with the quantity of macro-organisms (micro- and metafauna). The quantity of fauna representatives increased immediately after registration of higher quantity of bacteria. It is evident that we registered positive succession. The recovery of the fauna was better in Variant II, where additional peseudomonads were added (). At the end of the experiment, the number of pseudomonads decreased in both variants. The number of aerobic heterotrophs decreased from the beginning to the 48th hour (). Then, from 48 to 96 h, there was an increase in the number of heterotrophs. The aerobic heterotrophs were more abundant in the variant without added amaranth from the beginning to the 96th hour. In variant I, the high values of the azo-degrading bacteria were parallel to the development of the small flagellates, as well as the total number of macro-organisms, at the 72nd and the 96th hour (). In the control variant, there was a similar process of adaptation of the micro- and metafauna through homogenous bacterial cells. At the 72nd and the 96th hour in the control variant, due to the destruction of the flocs of the activated sludge, the number of the homogenous cells was increased. This was observed by microscopy.
Figure 11. Dynamics in the quantity of aerobic heterotrophs (а), pseudomonads (b) and azo-degrading bacteria (c) with the quantity of macro-organisms (micro- and metafauna) in Variant I (without P. aureofaciens AP-9) and Variant II (with P. aureofaciens AP-9).
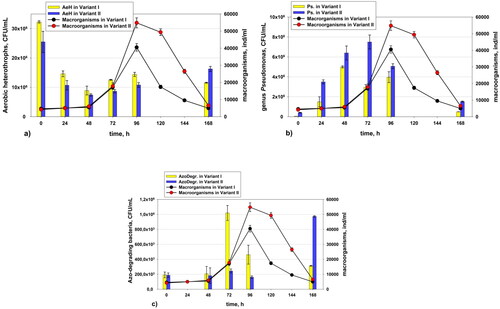
shows the bioaugmentation effect of the added P. aureofaciences AP-9, on the micro- and metafauna organisms in Variant II. The longest positive effect was that for small flagellates from 24 to 144 h. The highest effect occurred for free-swimming ciliates: 600% at the 144th hour and 993.33% at the 168th hour. At the beginning of the biodegradation process, from 0 to 72 h, the added bacteria also had a positive augmentation effect on testate amoebas, crawling and attached ciliates.
Table 1. Effect of added culture of Pseudomonas aureofaciens AP-9 in Variant II on the quantity of micro- and metafauna in activated sludge.
In terms of the total number of macro-organisms in the activated sludge, the added bacteria had a significant effect. There was a positive effect from 48 to 168 h: at the beginning of the process, the supported groups were the testate amoeba, crawling and attached ciliates, whereas in the second phase of the experiment, the small flagellates and free-swimming ciliates were supported.
Discussion
Under shock loading of water treatment processes with toxic pollutants, such as amaranth as an azo dye, activated sludge is generally subject to intoxication reactions. This is due to the accumulation in the flocs and in the representatives of the micro- and metafauna meta-fauna of toxic pollutants. In these intoxication reactions, the eukaryotic organisms in AS are most severely damaged. This is because of the very limited time of adaptation reactions and due to the larger accumulated amounts of toxic pollutants. This leads to a permanent disruption of the entire purification process. One of the mechanisms for protecting activated sludge is the use of microorganisms adapted to biodegradation. They increase the recovery capacity of activated sludge [Citation18].
Pseudomonas aureofaciens AP-9 was used in our experiment, which according to data in the literature has a wide detoxifying biodegradation potential to degrade xenobiotics with aromatic structure, including the azo dye amaranth [Citation18,Citation31]. In the variant with added P. aureofaciens AP-9, its elimination occurred at a higher rate, which was probably due to the added pre-adapted microbial culture. The addition of P. aureofaciens AP-9 did not significantly influence the early and the late elimination of amaranth (). The effect is clearly expressed in terms of the rehabilitation of the micro- and macro-segment of activated sludge. This was evident from the data of the quantitative and qualitative contents of fauna in AS after adding of P. aureofaciens AP-9. These contents will ensure better AS functional properties (better and quick adaptive reaction toward the toxic effect of amaranth as well as a better water purification process). All this was confirmed by the other results in this study. The biotic index of AS decreased throughout the process. This is a typical case in which the azo-dye amaranth had a harmful effect on the organisms of the micro- and metafauna (). A similar effect was described in another study, where the AS of the Kubratovo WWTP was negatively affected by shock loading with mazut [Citation39]. As a result of mazut, there was again a sharp increase in the number of flagellates and free-swimming ciliates cialites. The increased number of these two groups leads to a decrease in the values of the biotic index.
In variant II, the added P. aureofaciens AP-9 had an important role for the number of the micro- and metafauna (). The adaptation of the macro-organisms in conditions of toxic effect depends on the homogenous bacteria that are important food for the fauna. When the fauna accumulated amaranth, the added Pseudomonas benefitted rehabilitation of AS and especially the fauna complex in it, after shock loading with amaranth. This protective role is expressed by the following: 1/supply the fauna with more easily available food; 2/the adapted bacteria contain the detoxifying enzymes in the biomass and that way the fauna has ‘highly quality food’, which has a protective effect and stimulates the detoxification of amaranth in the representatives of the fauna. Thus, the fauna acquires resistance to the toxicant and survives. This effect has already been reported for other xenoboiotic pollutants, e.g. pentachlorophenol, after adding of the same P. aureofaciens AP-9 [Citation18]. In that study, as in the amaranth experiment, homogenous bacterial cells were added to the system, which provided the fauna with easily accessible food in larger quantities and of ‘higher quality’. ‘Higher quality’ is due to the high percentage of detoxification enzymes in pseudomonads [Citation18].
When discussing the results for key groups, especially for the testate amoeba, Dimkov et al. repeatedly prove their stable presence in the activated sludge treating leachate from a municipal landfill where they are constantly put under high concentrations of xenobiotics [Citation40]. This observation can be confirmed in the present study, where the number of amoebae remained relatively constant (). Even with the complete deterioration of conditions, their number increased. In both variants during the whole study the average number of the naked amoeba was more than that of the testate amoeba.
Small heterotrophic flagellates fed on the homogenous bacteria that are in abundance in variant II due to the added pseudomonads (). These bacteria play a protective role for the fauna because, while acting as feed, they provide flagellates with valuable enzymes against the toxicant in the system. Thus, this is a ‘2 in 1’ situation: first, the added bacteria are food for the flagellates and second, they play the role of ‘protectors’ owing to the enzymes synthesized by them. However, although the results indicated that amaranth elimination occurred at the 48th hour, it is difficult to prove whether the full biodegradation of this xenobiotic was completed. Some intermediate metabolites, such as aromatic amines, may remain in the system [Citation32].
As already mentioned, after the 96th hour, free-swimming cilates were dominant in AS (). This is to a great extent related to the fact that the addition of Pseudomonas aureofaciens AP-9 provided the optimal variant of food from homogenous bacterial cells for the free-swimming ciliates. This led not only to an increase in the number of representatives from this group but also to their survival during and after the shock influx of amaranth in the system. The quantity of the free-swimming ciliates in Variant II significantly exceeded that in the control at the end of the study. More evidence about the mechanism that the free-swimming ciliates use to survive and to adapt to the unfavourable conditions resulting from the added xenobiotic could be seen in .
The crawling and attached ciliates almost completely disappeared after the 96th hour (). This could be related to their function as a protector and a distributor of the xenobiotic for the bacteria. Since ciliates are bigger organisms, they accumulate a significant part of the toxicant. Thus, they suffer damage and die. After that, they start to discharge the ingested toxicant step-by-step and play the role of a distributor providing a step-by-step increase in the xenobiotic concentration. In support of this, the images in show a free-swimming ciliate that has ingested the red dye amaranth. The images support the suggestion that not only the crawling and attached ciliates but also the free-swimming ciliates have a protective role for the bacteria in the flocs of the activated sludge. Ciliates survive because they have the perfect food of free homogenous cells of Pseudomonas aureofaciens AP-9. Unlike the crawling and attached ciliates, free-swimming ciliates can move freely among the flocs of the activated sludge, which allows them to use the homogenous pseudomonad cells as food.
With regard to the metafauna organisms, the longest survival in the variant with the added pseudomonads could be explained with the fact that pseudomonads are easily accessible food that also provides a large set of enzymes (). They help microorganisms to survive a short period of time in the conditions of high xenobiotic concentrations. This observation is in agreement with previous studies on the biodegradation of mazut and PCP [Citation18,Citation39]. Metafauna cannot survive for a long time without oxygen. All this makes them a good bioindicator of deteriorating conditions in AS. A direct relation between genus Pseudomonas and small flagellates was especially obvious in the variant with the added culture of pseudomonads. These added bacteria had a clear bioaugmenting effect regarding the adaptation of the micro- and metafauna in the AS during the shock pollution with a xenobiotic [Citation18]. As mentioned above, due to the added homogenous cells in variant II, the representatives of the micro- and metafauna adapted easier. Thus, bioaugmentation does not have a significant effect directly on biodegradation, but improves the quality of AS. This is done by supporting micro- and metafauna [Citation18]. There was a similar dependence in the aerobic heterotrophs, which increased during the mass development of the small flagellates (а). The increased number of homogenous cells served as food and respectively as a protector of the micro- and metafauna in the conditions of toxic shock.
The added bacteria had a positive augmentation effect on testate amoebas, crawling and attached ciliates. These three groups had the highest value in determining the sludge biotic index. This means that at the beginning of the experiment, when there was intensive biodegradation of amaranth, the added bacteria had a positive augmentation effect on the key groups of the microfauna. The mechanism of supporting the process can be found in the direct addition of fresh food - biomass of bacterial cells for macro-organisms, which has a synthesized enzyme complex that helps the survival of protozoa. This was clearly registered in the variant with the added pure culture of Pseudomonas aureofaciens AP-9. At the end of the process, when the conditions of the experimental reactor tended to deteriorate, the added bacteria still supported the small flagellates and free-swimming ciliates, which are an indicator of a lower sludge biotic index. These two groups have the ability to swim freely in the mixed liquor and thus the added pseudomonads become easy food for them. In the already severely deteriorated conditions in the reactor at the end of the process, through the mechanism described above, bacteria of the genus Pseudomonas supported both flagellates and free-swimming ciliates.
Conclusions
The microfauna could adapt more effectively in the presence of added pseudomonads. In the process of adaptation, there was a stage of mass development of small flagellates and subsequent development of free-swimming ciliates, which is an indicator for the quality improvement of the AS. The micro- and metafauna have a very important significance for the activated sludge because they are important for the sustainment and the regulation of the bacterial counts. This unbreakable relation between the bacteria and the micro- and metafauna also determines the effectiveness of the water treatment processes. Microbial augmentation with Pseudomonas aureofaciens AP-9 would be very suitable for small modular treatment plants in the dye industry. Their addition would be important in case of shock loading of high concentrations of azo-dyes (over 150–200 mg/L). They would support the elimination process through their biodegradation activity, as well as with their supporting role in relation to the segments of micro- and metafauna.
Acknowledgments
This article is dedicated to my mother Stefka Yotinova.
Disclosure statement
No potential conflict of interest was reported by the authors.
Data availability statement
The data that support the findings of this study are available from the corresponding author, Ivaylo Yotinov, upon reasonable request.
Additional information
Funding
References
- Çiçek F, Özer D, Özer A, et al. Low cost removal of reactive dyes using wheat bran. J Hazard Mater. 2007;146(1–2):408–416.
- Forgacs E, Cserhati T, Oros G. Removal of synthetic dyes from wastewaters: a review. Environ Int. 2004;30(7):953–971.
- Singh PK, Singh RL. Bio-removal of azo dyes: a review. Int J Appl Sci Biotechnol. 2017;5(2):108–126.
- Salem SS, Mohamed A, El-Gamal M, et al. Biological decolorization and degradation of azo dyes from textile wastewater effluent by Aspergillus niger. Egypt J Chem. 2019;62(10):1799–1813.
- Lee YH, Pavlostathis SG. Decolorization and toxicity of reactive anthraquinone textile dyes under methanogenic conditions. Water Res. 2004;38(7):1838–1852.
- Shi Y, Yang Z, Xing L, et al. Recent advances in the biodegradation of azo dyes. World J Microbiol Biotechnol. 2021;37(8):1–18.
- Sreedharan V, Rao KVB. Biodegradation of textile azo dyes. In Nanoscience and biotechnology for environmental applications. Cham: Springer; 2019. p. 115–139.
- Pierce J. Colour in textile effluents-the origins of the problem. J Soc Dyers Colour. 1994;110(4):131–133.
- Jamee R, Siddique R. Biodegradation of synthetic dyes of textile effluent by microorganisms: an environmentally and economically sustainable approach. Eur J Microbiol Immunol (Bp). 2019;9(4):114–118.
- Pan H, Feng J, He GX, et al. Evaluation of impact of exposure of Sudan azo dyes and their metabolites on human intestinal bacteria. Anaerobe. 2012;18(4):445–453.
- Belouhova M. Management of wastewater treatment by biofilm communities in innovative detoxification technologies [Thesis]. Sofia (Bulgaria): SU st. Kliment Ohridski; 2015. p. 191. p.
- Alshehrei F. Role of microorganisms in biodegradation of food additive azo dyes: a review. Afr J Biotechnol. 2020;19(11):799–805.
- Chung KT. Azo dyes and human health: a review. J Environ Sci Health C Environ Carcinog Ecotoxicol Rev. 2016;34(4):233–261.
- Lau K, McLean WG, Williams DP, et al. Synergistic interactions between commonly used food additives in a developmental neurotoxicity test. Toxicol Sci. 2006;90(1):178–187.
- Kunz A, Peralta-Zamora P, Moraes SGD, et al. Novas tendências no tratamento de efluentes têxteis. Quím Nova. 2002;25(1):78–82.
- Lellis B, Fávaro-Polonio CZ, Pamphile JA, et al. Effects of textile dyes on health and the environment and bioremediation potential of living organisms. Biotechnol Res Innov. 2019;3(2):275–290.
- Spychała M, Sowińska A, Starzyk J, et al. Protozoa and metazoa relations to technological conditions of non-woven textile filters for wastewater treatment. Environ Technol. 2015;36(13–16):1865–1875.
- Topalova Y. 2009. Biological control and wastewater management. Sofia (Bulgaria): Publish-Sai-Set-Eco.
- Kozuharov D, Topalova J, Dimkov R, et al. Biodiversity response of micro- and metafauna in activated sludge towards toxic effect of ONP and PCP. Biotechnol Biotechnol Equip. 1999;13(2):80–86.
- Curds CR. The ecology and role of protozoa in aerobic sewage treatment processes. Annu Rev Microbiol. 1982;36(1):27–28.
- Kozuharov D, Topalova J, Dimkov R, et al. Bioindicative potential of the micro- and metafauna in the activated sludge towards ONP. Biotechnol Biotechnol Equip. 2001;15(1):92–98.
- Martín-Cereceda M, Serrano S, Guinea A. A comparative study of ciliated protozoa communities in activated-sludge plants. FEMS Microbiol Ecol. 1996;21(4):267–276.
- Kozuharov D, Topalova YI, Nakova EI, et al. Structure and functioning of micro and metafauna in activated sludge from waste water treatment plant of textile manufacturing. Acta Zool Bulgar. 2009;61(2):169–176.
- Ogleni N, Damar Arifoglu Y, Ileri R. Microbiological and performance evaluation of sequencing batch reactor for textile wastewater treatment. Water Environ Res. 2012;84(4):346–353.
- Ogleni N, Ovez S, Ogleni O. Wastewater characterization and microbial diversity of a textile industry wastewater treatment system. Fresenius Environ Bull. 2010;19:1911–1916.
- Ramirez E, Robles E, Martinez B, et al. Distribution of free-living amoebae in a treatment system of textile industrial wastewater. Exp Parasitol. 2014;145:S34–S38.
- dos Santos LA, Ferreira V, Pereira MO, et al. Relationship between protozoan and metazoan communities and operation and performance parameters in a textile sewage activated sludge system. Eur J Protistol. 2014;50(4):319–328.
- Herrero M, Stuckey DC. Bioaugmentation and its application in wastewater treatment: a review. Chemosphere. 2015;140:119–128.
- Cycoń M, Mrozik A, Piotrowska-Seget Z. Bioaugmentation as a strategy for the remediation of pesticide-polluted soil: a review. Chemosphere. 2017;172:52–71.
- Tondera K, Chazarenc F, Chagnon PL, et al. Bioaugmentation of treatment wetlands–a review. Sci Total Environ. 2021;775:145820.
- Topalova Y, Kozuharov D, Dimkov R. Adaptive response of trivial activated sludge towards toxic effect of oNP, PCP and combination oNP/PCP. Waste Manage (Oxford). 1999;19(4):247–254.
- Belouhova M, Schneider I, Chakarov S, et al. Microbial community development of biofilm in amaranth decolourization technology analysed by FISH. Biotechnol Biotechnol Equip. 2014;28(4):635–642.
- Grekova-Vasileva M. Bioalgorithms for management of wastewater treatment in textile industry [dissertation]. Sofia (Bulgaria): Sofia University; 2009.
- Sladka A, Sladeček V. A guide of organisms from waste water plants. Vyz Ustav Vodohosp v Praze Prace a Studie. 1985;162:156.
- Foissner W, Berger H. A user-friendly guide to the ciliates (protozoa, ciliophora) commonly used by hydrobiologists as bioindicators in Rivers, Lakes, and waste waters, with notes on their ecology. Freshwater Biol. 1996;35(2):375–482.
- Gerardi MH. 2008. Microscopic examination of the activated sludge process. Hoboken (NJ): John Wiley & Sons, Inc.
- Madoni P. A sludge biotic index (SBI) for the evaluation of the biological performance of activated sludge plants based on the microfauna analysis. Water Res. 1994;28(1):67–75.
- Kuznetsov SI, Dubinina GA. Methods of investigation of aquatic microorganisms. Chapter 19. In: Ecology and classification of North American freshwater invertebrates. 3rd ed. Cambridge (MA): Academic Press; 1989. p. 725–771.
- Topalova Y, Todorova Y, Schneider I, et al. Detoxification potential and rehabilitation of activated sludge after shock loading of sofia’s wastewater treatment plant ‘kubratovo’with mazut. Water Sci Technol. 2018;78(3–4):588–601.
- Dimkov R, Topalova Y, Schneider I. Environmental biotechnology. Sofia (Bulgaria): Publish Sci-Set Eco; 2017.