Abstract
Polymorphisms in the genes of drug-metabolizing enzymes have the potential to contribute to inter-individual differences in drug pharmacokinetics and toxicity. A custom next-generation sequencing (NGS) panel was used in 71 kidney transplanted patients to study the polymorphisms of 11 genes relevant to the metabolism of immunosuppressive drugs. Cyclosporine A and tacrolimus concentrations were determined by a validated liquid chromatography with tandem mass spectrometry (LC-MS/MS) method. More than 1000 polymorphisms were found in the studied 11 genes, and 45% of them were different non-synonymous variants. Eleven missense mutations were observed in the CYP3A7 gene, resulting in increased metabolism of tacrolimus at day 21 post-transplantation (6.7 µg/L vs. 10.3 µg/L; p = 0.048). Two alleles encoding a cytochrome P450 2D6 enzyme with impaired function—CYP2D6*4 (non-functional) and CYP2D6*10 (decreased function), were found in the studied group and both of them were associated with higher levels of tacrolimus at day 14 (10.1 µg/L; p = 0.021 and 9.7 µg/L; p = 0.036, vs. 7.7 µg/L respectively). Altered function of ABC transporters C3 and C2 was also associated with increased TAC concentration. ABCC3 significantly influenced TAC metabolism by itself, but polymorphisms affecting both ABCC3 and ABCC2 resulted in higher changes: 13.6 µg/L vs. 7.9 µg/L, day 14 (p = 0.003) and 20 µg/L versus 9.3 µg/L, day 21 (p = 0.019). All associations were also checked for variants affecting the activity of CYP3A4 and CYP3A5. Despite its small size, the study points out that the pharmacogenetics of calcineurin inhibitors may also be influenced by other genes besides CYP3A4 and CYP3A5.
Introduction
Despite the significant advances in terms of surgical techniques, tissue typing and patient care, most of the progress in organ transplantation is largely attributable to the recognized importance of immunosuppressive therapy [Citation1–3]. Therapeutic drug monitoring (TDM) and pharmacogenetic studies have been inspired by the large inter- and intra-individual variability of immunosuppressive drugs. The focus has been on proteins which are involved in their pharmacodynamics and on their dose-concentration relationships [Citation4]. Single nucleotide polymorphisms (SNPs) in genes associated with drug metabolism seem to influence the post-transplant outcome and to modulate the adverse effects of immunosuppressants.
Since the introduction of Cyclosporine A (CsA) and tacrolimus (TAC) in the clinical practice, they have considerably improved graft survival in solid organ transplantations (SOT). Their bioavailability and metabolism are primarily controlled by efflux pumps (ABCB) and 3A4 and 3A5 members of the cytochrome P450 family. Therefore, part of the inter-individual variability in drugs’ pharmacokinetics is explained by genetics [Citation5].
The four genes CYP3A4, CYP3A5, CYP3A7 and CYP3A43 form one of the most versatile biotransformation systems that facilitate the elimination of drugs—the human CYP3A subfamily [Citation6–9]. As mentioned by Lamba et al. [Citation6], about 30% of cytochrome P450 function in liver comes from CYP3A4 and CYP3A5. Approximately half of the medications that are metabolized by CYP450 are CYP3A substrates. Both enzymes are expressed in the intestine and liver, while CYP3A5 is also the predominant form expressed in extrahepatic tissues [Citation7]. CYP3A5 is highly polymorphic and one of the polymorphisms within intron 3 (rs776746, CYP3A5*3) results in a splicing defect, leading to a truncated protein with no enzyme activity [Citation6, Citation8]. Many studies have focused on CsA metabolism, but no sharp genetic variations have been identified. In contrast to cyclosporine, a strong association between pharmacokinetics of tacrolimus and CYP3A5 genotype (*1/*3) has been clearly demonstrated in patients after renal transplantation [Citation4, Citation7, Citation10, Citation11]. The therapeutic concentration dose was estimated to be twice as much in carriers of at least one CYP3A5*1 or *3 allele compared to non-carriers.
CsA and TAC are also substrates of P-glycoprotein (P-gp), the major efflux transporter encoded by the ABCB1 gene [Citation11, Citation12]. ABCB is one of the 7 subfamilies of the human ATP-binding cassette (ABC) transporter superfamily. These transporters play a pivotal role in many cellular and metabolic processes, transporting a number of divergent endogenous substrates across the plasma and intracellular membranes [Citation13]. Many studies address the influence of ABCB1 SNPs on CsA or TAC pharmacokinetics but the results remain uncertain [Citation5, Citation11, Citation14, Citation15]. However, polymorphisms in ABCB1 may affect the efficacy or toxicity of calcineurin inhibitors (CNIs). The role of ABCC transporters in the metabolism of CNIs has been significantly less studied, with studies focusing on polymorphisms in the ABCC2 gene.
In this study the polymorphisms of 11 genes relevant to the metabolism of CsA and TAC were investigated with the aim to assess their potential to influence the bioavailability of these immunosuppressants [Citation16–19].
Subjects and methods
Ethics statement
The study was approved by the Hospital Ethics Committee and all patients signed informed consent forms.
Patients
The study encompassed 71 patients transplanted in the period 2015–2020 at University Hospital ‘Alexandrovska’ Sofia, Bulgaria. Immediate graft function after operation and lack of early post-operative complications were required as inclusion criteria. Patients were followed in the early post-transplant period, during the first month after transplantation. Demographic and clinical characteristics of the subjects are presented in . Thirty-three patients treated with CsA twice daily, glucocorticoids and azathioprine (older immunosuppressive regime after kidney transplantation) were selected from our database to be compared to patients who started with the current immunosuppressive regime utilizing TAC. Thirty-eight patients were on TAC, mycophenolate mofetil and glucocorticoids (current immunosuppressive regime in our transplant center). Data were collected from the patients’ medical records. Blood samples collected during regular pre-transplantation clinical visits were used for genotyping.
Table 1. Patients’ characteristics.
Drug monitoring
Determination of CsA and TAC, simultaneously with sirolimus and everolimus was performed by a previously validated and published LC-MS/MS method [Citation20]. It utilizes methanol/Zn sulfate solution for cell lysis, extraction with 1-chlorobutane, [D12]CsA (dCsA), ascomycin (Asco) and [13c2d4]RAD001 (cdRAD) used as internal standards, isocratic elution on C18 analytical column, positive-ion electrospray ionization, and selected reaction monitoring for the respective m/z transitions: 1203→425 for CsA, 822→766 for TAC, 1215→437 for dCsA, 809→756 for Asco and 982→915 for cdRAD. The method was validated according to FDA (United States Food and Drug Administration) and EMA (European Medicines Agency) guidance requirements, and ASI/LGS proficiency testing certified, with documented selectivity and matrix effect of 92–113%, accuracy and precision within 8.9%; extraction recoveries averaging 65–76%; linearity range with R2 > 0.995, freeze-thaw stability for three cycles of 24 h, post-preparative stability for 96 h at 10 °C, short-term stability at ambient temperature for 12 h in the dark and for 6 h at daylight; stock solution stability and long-term stability in whole human blood—for 7 days at 4–8 °C, and for 120 days at −20 °C.
TAC concentrations on days 7, 14, 21 and 30 post-transplantation were related to the pharmacogenetic study. The collection of data for CsA depended on the availability of samples for genotyping and therefore, reflected different individual time periods in later post-transplant period without matching to patients on TAC. Therefore, associations of genetic polymorphisms with CsA levels were only mentioned in this paper.
NGS panel
We have designed a custom NGS panel with 11 genes known to be associated with drug response, using AmpliSeq Designer. The panel covered 99.5% of the exon regions and included the following genes: SLCO1B1, CYP3A4, CYP3A5, CYP3A7, CYP2C19, CYP2C9, CYP2C8, CYP2D6, ABCB1, ABCC2 and ABCC3. For the amplification, primer pairs were divided into two panel pools. The length of the amplicons was between 125 and 275 bp, with the majority of amplification products of about 275 bp. The NGS libraries were prepared using IonAmpliSeq Library kit v2.0. Templates were prepared by Ion Chef 540 kit. Sequencing was performed on S5 by IonS5 Sequencing kit and 540 Chip. Variants in genes analyzed were assigned using Ion Reporter.
Statistical analysis
Descriptive statistics approach was used to characterize the groups of patients. Mann–Whitney U test, Chi-squared and Fischer’s exact test were used to evaluate differences between groups. In all tests, differences were considered statistically significant at the p ≤ 0.050 level. Statistical analyses were performed via SPSS 16.0 (IBM Corp., New York, USA) software.
Results
The patients’ demographics and characteristics are presented in . Dose optimization in the first month post transplantation was performed according to the following targets: CsA trough levels within 100–200 µg/L for transplants from cadaver donors and between 150–250 µg/L for transplants from live donors; TAC trough levels within 8–12 µg/L for transplants from cadaver donors and between 10–15 µg/L for transplants from live donors.
Over 1000 polymorphisms were identified in the genes covered by the NGS panel: synonymous (65%), missense (30%), frame-shift (3%), nonsense (>1%), as well as several unknown SNPs (data not shown). Of the unknown gene variants, 5 had a change in the reading frame and 12 had a non-synonymous codon change, leading to amino acid substitution. The ATP-binding proteins showed highest polymorphism level with 547 gene variants found in the studied cohort. The gene variants detected in the CYP450 family were 462.
From the 11 genes included in the panel (described in Subjects and methods section) only CYP2C19, CYP3A7, CYP2D6, ABCC2 and ABCC3 showed statistically significant association with the TAC and CsA levels and the paper focuses on them. The polymorphisms in the CYP3A4 and CYP3A5 genes that were found in the studied group did not show statistically significant association with the CNI’s metabolism. Therefore, because a significant portion of their metabolism passes through these genes, the results described below reflect the potential presence of other mechanisms, pathways, or interactions.
CYP2C19
Most of the patients were carriers of the CYP2C19*1 allele. Patients with CYP2C19*1B allele had significantly higher levels of TAC on day 21 (p = 0.041, OR = 13; 1.1–147.8). This result was unexpected with regard that *1B allele is considered to have a normal function. We further examined the carriers of *1B allele and found that 3 of them (33%) were with higher levels of TAC (mean 24.7 µg/L). One of these 3 patients was a carrier of the nonfunctional CYP2D6*4 allele, and the second was a carrier of CYP2C9*2 allele. CYP2C9 is one of the enzymes that metabolize TAC and *2 allele encodes an isoform with decreased function. Since the role of CYP2C19 in TAC metabolism is often associated with CYP3A5 enzyme, the group was controlled for the presence of CYP3A5*3 and/or CYP3A5*5 alleles. No statistically significant difference was observed.
CYP3A7
A total of 11 missense mutations were identified in comparison to the wild type: 10 CYP3A7*2 alleles (rs2257401; 7 patients on CsA and 3 patients on TAC) and 1 rs765087201 in a patient on TAC (). All of them were associated with significantly lower concentrations of TAC on day 21, compared to the wild type (6.7 µg/L vs. 10.3 µg/L). Interestingly, none of the patients in the TAC group carried a variant with decreased enzyme activity in CYP3A4 and CYP3A5 genes. A lower concentration of CsA was also observed in these non-wild-type carriers—66.7 µg/L versus 95.1 µg/L. This observation was not statistically significant and due to the specificity of our CsA group (mentioned in Subjects and methods section), conclusions should be made with caution. In the CsA group, one patient was a carrier of CYP3A5*3B and CYP3A5*5 alleles, both of which encode a non-functional protein and could explain the result in that particular patient. The other 6 patients were with wild-type CYP3A4/CYP3A5 (codes enzymes with normal function).
Table 2. Influence of the CYP3A7 gene variants on the tacrolimus concentration in the post-Tx period.
CYP2D6
Eighteen different frame-shift and missense polymorphisms with a total of 87 replicates were identified in the CYP2D6 gene. At least one polymorphism was detected in 36 patients (50.7%), which makes the CYP2D6 gene the most polymorphic in the analyzed panel. Of all individual rs polymorphisms only rs3892097 (CYP2D6*4 which encodes a non-functional enzyme) and rs1065852 (CYP2D6*10—the encoded enzyme is with decreased function) were associated with a statistically significant increase in the concentration of TAC on day 14 post-transplantation (). CYP2D6*4 carriers showed mean concentration of 10.1 µg/L compared to 7.7 µg/L for the non-carriers (p = 0.021). CYP2D6*10 carriers showed mean TAC concentration of 9.7 µg/L on day 14 post-transplantation, compared to 7.7 µg/L for the non-carriers (p = 0.036). We also compared the patients’ phenotypes: poor metabolizers (carriers of two alleles with decreased function) and intermediate metabolizers (one allele with decreased function and one with normal) against patients with normal activity of CYP2D6 enzyme (carriers of wild-type alleles, ). Again, the results were statistically significant: higher concentration of TAC on day 14 post-transplantation was observed in poor and intermediate metabolizers, compared to normal metabolizers (9.3 µg/L vs. 7.5 µg/L, p = 0.022). Due to the well-established role of CYP3A4 and CYP3A5 in the metabolism of CNIs, we examined their effect on the results of CYP2D6. All CYP2D6*4 and CYP2D6*10 carriers in the TAC group had wild-type CYP3A4 and CYP3A5 genes (coding enzymes with normal function).
Table 3. Influence of the CYP2D6 gene variants on the tacrolimus concentration in the post-Tx period.
Regarding the CYP2D6 and CsA metabolism, the carriers of alleles encoding enzymes with decreased activity had higher CsA concentrations: 138.8 µg/L versus 72.9 µg/L (p = 0.646). Only one patient in this group was also a carrier of a non-functional CYP3A5 enzyme.
ABCC3
Variants in ABCC3 were also found to correlate with TAC levels: patients with wild-type allele had significantly lower TAC concentrations on day 14 post-transplantation compared to non-wild type carriers (7.7 µg/L vs. 9.7 µg/L, p = 0.022). The same trend was observed on day 21 post-transplantation (8.5 µg/L vs. 14 µg/L, p = 0.041, ).
Table 4. Influence of the ABCC3 gene variants on the tacrolimus concentration in the post-Tx period.
Due to the overlapping function of ABCC3 and ABCC2 transporters, we also studied the combined effect of variants in their genes. As shown in , TAC levels were higher in patients with mutations in both ABCC2 and ABCC3, and that tendency was detected as early as day 7 post-transplantation: 11.3 µg/L, compared to 6.4 µg/L in patients who had wild-type phenotype or mutation in only one of the ABCC2 and ABCC3 genes, p = 0.053 (). That difference reached statistical significance on day 14 and on day 21 post-transplantation (13.6 µg/L vs. 7.9 µg/L, p = 0.003, , and 20.0 µg/L vs. 9.3 µg/L, p = 0.019, ). Once again, none of the patients in the group was a carrier of non-functional (or with decreased activity) CYP3A4 and/or CYP3A5 enzyme.
Figure 1. Effects of ABCC2-ABCC3 phenotypes on tacrolimus concentrations on days 7, 14 and 21 post-transplantation. In two patients, both ABCC2 and ABCC3 genes were with impaired metabolic activity with tacrolimus concentrations significantly higher compared to wild types and intermediate metabolizers (n = 36), as early as day 7 (A, p = 0.053), day 14 (B, p = 0.003) and day 21 (C, p = 0.019) post transplantation.
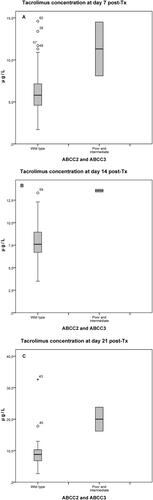
Discussion
Several studies report association of CYP2C19 with TAC bioavailability, but its role is mainly in combination with CYP3A5 polymorphisms [Citation10, Citation21–23]. Our results did not show association between the polymorphisms in CYP2C19. The only observation, decreased levels of TAC in CYP2C19*1 allele carriers, was statistically unstable and was the result of the influence of polymorphisms in other genes, due to the small group we studied. None of the TAC patients carried alleles with impaired CYP3A5 and/or CYP3A4 activity, which did not allow us to assess the combinatorial effect of CYP2C19 and CYP3A5/CYP3A4 poor metabolizers.
An interesting result was observed for CYP3A7, one of the four CYP3A enzymes in humans [Citation8]. While CYP3A7 is the main CYP3A enzyme in fetus liver, its function is gradually replaced by CYP3A4 after birth [Citation24]. Its presence was found to be tissue-specific and dependent on the developmental stage [Citation9]. However, there are data showing that in about 10% of the adults CYP3A7 expression is higher than CYP3A5, which may be relevant for the metabolism of TAC [Citation25]. In our cohort of patients, we found only two variants besides the wild type: 10 patients had the CYP3A7*2 allele (rs2257401) and one had rs765087201. The C-G substitution in CYP3A7*2 carriers leads to a Thr/Arg amino acid substitution, thereby impairing enzyme activity [Citation26, Citation27]. Our results showed that both TAC and CsA are affected, as their concentrations are significantly lower compared to wild-type carriers. Such correlation was also reported by other groups [Citation7, Citation27, Citation28].
Two studies report that CYP3A7 rs2257401 negatively affects the metabolism of CsA [Citation28] and dose-adjusted TAC trough concentrations (C0/D) in adult kidney transplant recipients [Citation27]. Liu et al. [Citation7] reported a two-fold higher concentration of TAC in wild type CYP3A7 (GG) compared to CC/CG carriers (rs2257401) in pediatric patients with nephrotic range proteinuria. Similar to the mechanism for persistent CYP3A7 expression in adults in CYP3A7*1C carriers, Liu et al. [Citation7] and Burk et al. [Citation29] proposed that rs2257401 results in high expression of the CYP3A7 gene, leading to change in enzymatic activity. Our data confirm those observations, as both TAC and CsA concentrations are significantly lower in CC/CG carriers (rs2257401) compared to wild-type carriers.
These findings suggest that CYP3A7 rs2257401 may serve as a significant genetic marker for the administration of CNIs. The CYP3A7*2 allele frequency differs substantially between ethnic groups, being higher in Asian populations and lower in Caucasians [Citation27, Citation30, Citation31]. In our patient cohort, the CYP3A7*2 allele was observed in 14.1% of individuals, making it the second most frequent allele after the wild type CYP3A7*1. This relatively high frequency indicates that a substantial proportion of patients may carry this allele, which may have a significant impact on their immunosuppressive protocols and the outcome of transplantation.
Relatively surprising results were obtained in the analysis of the effect of CYP2D6 on the metabolism of tacrolimus. CYP2D6 metabolizes around 25% of currently prescribed drugs, including various antidepressants, neuroleptics, beta-blockers, opioids, antiemetics and antiarrhythmics [Citation32]. CYP2D6 is also characterized with extensive polymorphism with more than 100 allelic variants described so far [Citation32, Citation33]. Allele frequencies differ substantially between ethnic groups and geographic regions and can entail clinically important differences in drug response. Several of these alleles are non-functional or with decreased activity—CYP2D6*3, *4 and *5 being more frequent in Caucasian populations, and CYP2D6*10 more characteristic for Asian populations [Citation32, Citation33].
In the studied group of patients, we found two alleles encoding a non-functional/decreased activity enzyme, CYP2D6*4 and CYP2D6*10. Both alleles associate with higher levels of TAC. Phenotypes showed even stronger association: the TAC concentration was higher in poor and intermediate metabolizers, compared to patients in whom the function of the CYP2D6 enzyme is not affected. Although the clinical pharmacokinetics of TAC has been reviewed repeatedly, we could not find reported associations between CYP2D6 polymorphism and TAC concentration. TAC is co-medicated with multiple drugs under clinical conditions. It is highly lipophilic and is excreted from the body after extensive metabolism predominantly mediated by CYP3A4 and secondarily by CYP3A5 [Citation34–36]. It is hypothesized that only a small fraction of TAC may be metabolized by other members of the cytochrome P450 superfamily, including CYP2D6 [Citation36].
As TAC concentration seems to be influenced mainly by CYP3A4 and CYP3A5, our data showed that on days 14 and 21 post-transplantation, the blood concentrations of TAC were significantly influenced by polymorphisms in the ABCC3 gene. Members of the ABCC family are major determinants of drug resistance, as they limit the bioavailability of various drugs by influencing their adsorption, distribution and elimination or restricting permeation through blood-tissue barriers, respectively [Citation13, Citation37]. Twelve members of the MRP family ABCC have been described so far. They are located on different chromosomes and share only low sequence identities. The most important transporters of the MRP family in respect to drug metabolism and clinical outcome are the members ABCC2 and ABCC3 [Citation13]. They are expressed in various tissues including liver, kidneys and intestine [Citation13, Citation38, Citation39]. ABCC2 localization is on the apical membrane of the polarized cells, like hepatocytes and enterocytes, mediating excretion, whereas ABCC3 is localized on the basolateral membrane [Citation13, Citation38]. Both transporters are known to have a very broad substrate specificity, including TAC. The transported substrates of ABCC2 and ABCC3 partially overlap and although ABCC3 is not abundantly present in the healthy liver, a strong upregulation of ABCC3 is observed when ABCC2 function is decreased or absent [Citation13, Citation16, Citation38, Citation40, Citation41].
To the best of our knowledge, association between SNPs in ABCC3 and TAC metabolism has not been reported so far. The gene has been relatively poorly studied, with data focusing primarily on its polymorphism and protein function [Citation13, Citation38, Citation42]. Few studies report on the role of ABC transporters in the pharmacokinetics of TAC [Citation16–19]. Ogasawara et al. [Citation16] showed that CYP3A5 expressers (CYP3A5*1 carriers) and MRP2 (ABCC2) high activity group (H2/H2 and H1/H2) decreased the dose-normalized trough concentration of TAC by 2.3-fold (p < 0.001) and 1.5-fold (p = 0.007), respectively. Genvidir et al. [Citation19] reported that ABCC2 c.3972C > T (rs3740066, reported also by Ogasawara et al. [Citation16]) polymorphism affected tacrolimus C/D in Brazilian kidney transplant recipients (n = 148). Renders et al. [Citation18] report that the combined effect of ABCC2 − 24C > T and 1249 G > A rather than individual polymorphisms influence the TAC trough concentration in adult recipients (n = 154). We were not able to evaluate the influence of −24 C > T and c.3972C > T, because those variants were not present in our patient cohort. Nevertheless, the low-activity ABCC2-ABCC3 haplotypes (poor metabolizers) showed an impaired ability to metabolize TAC, compared to normal proteins. The slight difference between previous studies can probably be explained by the lack of data on the ABCC3 transporter, which seems to compensate for the function of ABCC2 in the case of a non-functional protein [Citation13, Citation16, Citation38, Citation40, Citation41]. For the same reason, we can explain the normal levels of patients with an intermediate haplotype—non-functional ABCC2/ABCC3 and normal ABCC3/ABCC2 haplotypes. Of course, the very small number of patients included in our study should also be noted. In any case, our data confirm the role of ABC transporters in the pharmacokinetics of TAC and indicate the need for further and more extensive research in that field.
As the TAC concentration seems to be influenced mainly by CYP3A4 and CYP3A5, we checked all associations in this study for additional mutations in these genes that could explain our results. None of the patients on TAC had a non-functional CYP3A4 and/or CYP3A5 enzyme. Only individual patients in the CsA group had variants leading to impaired function of one of these two enzymes.
There are two limitations of this study: first, the small number of patients included, which affects the strength of conclusions; and second, the data for CsA reflected time periods in later post-transplant period which did not match the time periods of patients on TAC. Therefore, the associations between genetic polymorphisms and CsA levels were only slightly mentioned in the interpretation and assessment of results.
Conclusions
As the role of CYP3A4 and CYP3A5 for the metabolism CNIs is well described and the polymorphisms in their genes are relatively well characterized, this study focused on the assessment of the potential of other genetic factors. Among the 11 genes included in the panel, CYP2C19, CYP3A7, CYP2D6, ABCC2 and ABCC3 showed statistically significant association with TAC and CsA trough levels. Despite the small sample size, our results demonstrate that other genes, apart from CYP3A4 and CYP3A5, may also have a significant effect on the pharmacokinetics of TAC and possibly of CsA. Larger-scale studies of polymorphisms in gene complexes rather than of individual genes will obviously be needed to better characterize the diversity of CNIs metabolic pathways and their clinical significance.
Abbreviations | ||
= | Therapeutic drug monitoring | |
TDM | = | Cyclosporine A |
CsA | = | Tacrolimus |
TAC | = | Solid organ transplantation |
SOT | = | ATP-binding cassette |
ABC | = | Calcineurin inhibitors |
CNIs | = |
Data availability statement
The data that support the findings of this study are available from the corresponding author, upon reasonable request.
Disclosure statement
The authors do not have any conflicts of interest to declare.
Additional information
Funding
References
- Provenzani A, Santeusanio A, Mathis E, et al. Pharmacogenetic considerations for optimizing tacrolimus dosing in liver and kidney transplant patients. World J Gastroenterol. 2013;19(48):9156–9173.
- Penninga L, Wettergren A, Chan AW, et al. Calcineurin inhibitor minimisation versus continuation of calcineurin inhibitor treatment for liver transplant recipients. Cochrane Database Syst Rev. 2012;3:CD008852.
- Ponticelli C. Present and future of immunosuppressive therapy in kidney transplantation. Transplant Proc. 2011;43(6):2439–2440.
- Picard N, Marquet M. The influence of pharmacogenetics and cofactors on clinical outcomes in kidney transplantation. Expert Opin Drug Metab Toxicol. 2011;7(6):731–743.
- Tang JT, Andrews LM, van Gelder T, et al. Pharmacogenetic aspects of the use of tacrolimus in renal transplantation: recent developments and ethnic considerations. Expert Opin Drug Metab Toxicol. 2016;12(5):555–565.
- Lamba J, Hebert JM, Schuetz EG, et al. PharmGKB summary: very important pharmacogene information for CYP3A5. Pharmacogenet Genomics. 2012;22(7):555–558.
- Liu H, Xu Q, Huang W, et al. CYP3A5 and CYP3A7 genetic polymorphisms affect tacrolimus concentration in pediatric patients with nephrotic range proteinuria [published correction appears in Eur J Clin Pharmacol. 2021 Jan 30]. Eur J Clin Pharmacol. 2019;75(11):1533–1540.
- Lam YWF. Pharmacogenomics. In: Lam YWF, Scott SA, editors. Principles of pharmacogenomics: pharmacokinetic, pharmacodynamic, and clinical implications. 2nd ed. London (UK): Academic Press; 2019. p. 1–53.
- Rodriguez-Antona C, Axelson M, Otter C, et al. A novel polymorphic cytochrome P450 formed by splicing of CYP3A7 and the pseudogene CYP3AP1. J Biol Chem. 2005;280(31):28324–28331.
- Passey C, Birnbaum AK, Brundage RC, et al. Dosing equation for tacrolimus using genetic variants and clinical factors. Br J Clin Pharmacol. 2011;72(6):948–957.
- Capron A, Mourad M, De Meyer M, et al. CYP3A5 and ABCB1 polymorphisms influence tacrolimus concentrations in peripheral blood mononuclear cells after renal transplantation. Pharmacogenomics. 2010;11(5):703–714.
- Saeki T, Ueda K, Tanigawara Y, et al. Human P-glycoprotein transports cyclosporin A and FK506. J Biol Chem. 1993;268(9):6077–6080.
- Bruhn O, Cascorbi I. Polymorphisms of the drug transporters ABCB1, ABCG2, ABCC2 and ABCC3 and their impact on drug bioavailability and clinical relevance. Expert Opin Drug Metab Toxicol. 2014;10(10):1337–1354.
- Shuker N, Bouamar R, Weimar W, et al. ATP-binding cassette transporters as pharmacogenetic biomarkers for kidney transplantation. Clin Chim Acta. 2012;413(17–18):1326–1337.
- Vafadari R, Bouamar R, Hesselink DA, et al. Genetic polymorphisms in ABCB1 influence the pharmacodynamics of tacrolimus. Ther Drug Monit. 2013;35(4):459–465.
- Ogasawara K, Chitnis SD, Gohh RY, et al. Multidrug resistance-associated protein 2 (MRP2/ABCC2) haplotypes significantly affect the pharmacokinetics of tacrolimus in kidney transplant recipients. Clin Pharmacokinet. 2013;52(9):751–762.
- Zhao W, Elie V, Roussey G, et al. Population pharmacokinetics and pharmacogenetics of tacrolimus in de novo pediatric kidney transplant recipients. Clin Pharmacol Ther. 2009;86(6):609–618.
- Renders L, Frisman M, Ufer M, et al. CYP3A5 genotype markedly influences the pharmacokinetics of tacrolimus and sirolimus in kidney transplant recipients. Clin Pharmacol Ther. 2007;81(2):228–234.
- Genvigir FDV, Nishikawa AM, Felipe CR, et al. Influence of ABCC2, CYP2C8, and CYP2J2 polymorphisms on tacrolimus and mycophenolate sodium-based treatment in Brazilian kidney transplant recipients. Pharmacotherapy. 2017;37(5):535–545.
- Filipov J, Zlatkov B, Paskalev E, et al. Relationship between vitamin D status and immunosuppressive therapy in kidney transplant recipients. Biotechnol Biotechnol Equip. 2015;29(2):331–335.
- Imamura CK, Furihata K, Okamoto S, et al. Impact of cytochrome P450 2C19 polymorphisms on the pharmacokinetics of tacrolimus when coadministered with voriconazole. J Clin Pharmacol. 2016;56(4):408–413.
- Suetsugu K, Mori Y, Yamamoto N, et al. Impact of CYP3A5, POR, and CYP2C19 polymorphisms on trough concentration to dose ratio of tacrolimus in allogeneic hematopoietic stem cell transplantation. IJMS. 2019;20(10):2413.
- Hosohata K, Masuda S, Katsura T, et al. Impact of intestinal CYP2C19 genotypes on the interaction between tacrolimus and omeprazole, but not lansoprazole, in adult living-donor liver transplant patients. Drug Metab Dispos. 2009;37(4):821–826.
- Stevens JC, Hines RN, Gu C, et al. Developmental expression of the major human hepatic CYP3A enzymes. J Pharmacol Exp Ther. 2003;307(2):573–582.
- Sim SC, Edwards RJ, Boobis AR, et al. CYP3A7 protein expression is high in a fraction of adult human livers and partially associated with the CYP3A7*1C allele. Pharmacogenet Genom. 2005;15(9):625–631.
- CYP3A7 gene. Pharmavar – pharmacogene variation consortium web site. https://www.pharmvar.org/genes. Accessed May 04, 2020.
- Sohn M, Kim MG, Han N, et al. Whole exome sequencing for the identification of CYP3A7 variants associated with tacrolimus concentrations in kidney transplant patients [published correction appears in Sci Rep. 2020 Feb 7;10(1):2486]. Sci Rep. 2018;8(1):18064.
- Sun B, Guo Y, Gao J, et al. Influence of CYP3A and ABCB1 polymorphisms on cyclosporine concentrations in renal transplant recipients. Pharmacogenomics. 2017;18(16):1503–1513.
- Burk O, Tegude H, Koch I, et al. Molecular mechanisms of polymorphic CYP3A7 expression in adult human liver and intestine. J Biol Chem. 2002;277(27):24280–24288.
- Rodríguez-Antona C, Jande M, Rane A, et al. Identification and phenotype characterization of two CYP3A haplotypes causing different enzymatic capacity in fetal livers. Clin Pharmacol Ther. 2005;77(4):259–270.
- Crettol S, Venetz JP, Fontana M, et al. CYP3A7, CYP3A5, CYP3A4, and ABCB1 genetic polymorphisms, cyclosporine concentration, and dose requirement in transplant recipients. Ther Drug Monit. 2008;30(6):689–699.
- Petrović J, Pešić V, Lauschke VM. Frequencies of clinically important CYP2C19 and CYP2D6 alleles are graded across Europe. Eur J Hum Genet. 2020;28(1):88–94.
- Bertilsson L, Dahl ML, Dalén P, et al. Molecular genetics of CYP2D6: clinical relevance with focus on psychotropic drugs. Br J Clin Pharmacol. 2002;53(2):111–122.
- Iwasaki K. Metabolism of tacrolimus (FK506) and recent topics in clinical pharmacokinetics. Drug Metab Pharmacokinet. 2007;22(5):328–335.
- Luo X, Zhu LJ, Cai NF, et al. Prediction of tacrolimus metabolism and dosage requirements based on CYP3A4 phenotype and CYP3A5(*)3 genotype in Chinese renal transplant recipients. Acta Pharmacol Sin. 2016;37(4):555–560.
- Shiraga T, Niwa T, Teramura Y, et al. Oxidative metabolism of tacrolimus and its metabolite by human cytochrome P450 3A subfamily. Drug Metab Pharmacokinet. 1999;14(4):277–285..
- Cascorbi I, Haenisch S. Pharmacogenetics of ATP-binding cassette transporters and clinical implications. Methods Mol Biol. 2010;596:95–121.
- van der Schoor LW, Verkade HJ, Kuipers F, et al. New insights in the biology of ABC transporters ABCC2 and ABCC3: impact on drug disposition. Expert Opin Drug Metab Toxicol. 2015;11(2):273–293.
- Nishimura M, Naito S. Tissue-specific mRNA expression profiles of human ATP-binding cassette and solute carrier transporter superfamilies. Drug Metab Pharmacokinet. 2005;20(6):452–477.
- König J, Rost D, Cui Y, et al. Characterization of the human multidrug resistance protein isoform MRP3 localized to the basolateral hepatocyte membrane. Hepatology. 1999;29(4):1156–1163.
- Donner MG, Keppler D. Up-regulation of basolateral multidrug resistance protein 3 (Mrp3) in cholestatic rat liver. Hepatology. 2001;34(2):351–359.
- Mohammad IS, He W, Yin L. Understanding of human ATP binding cassette superfamily and novel multidrug resistance modulators to overcome MDR. Biomed Pharmacother. 2018;100:335–348.