Abstract
This study is focused on determination of the most appropriate synthetic approach for obtaining series of theophylline-7-acetyl semi- and thiosemicarbazide hybrids by using the molecular hybridization strategy with and without a catalyst. The target derivatives were further investigated for their in vitro neurotoxic and neuroprotective effects on cellular and subcellular level, along with their possible MAO-B inhibitory activities. The results identified 2-(1,3-dimethyl-2,6-dioxo-2,3,6,7-tetrahydro-1H-purin-7-yl)-N-[(methylcarbamo-thioyl)amino] acetamide (5) as the most promising structure for further in vivo evaluations with the lowest neurotoxicity and the highest neuroprotection and MAO B inhibitory activity of 28%.
Graphical Abstract
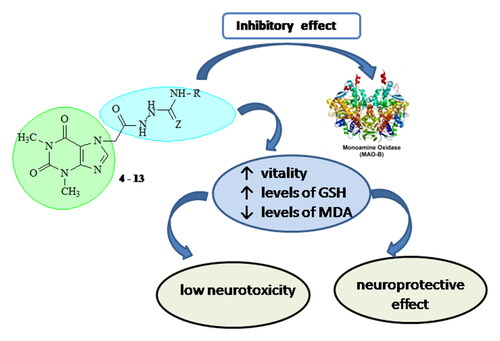
The results identified 2-(1,3-dimethyl-2,6-dioxo-2,3,6,7-tetrahydro-1H-purin-7-yl)-N-[(methylcarbamothio-yl)amino]acetamide (5) as the most prospective structure for further in vivo evaluations with the lowest neurotoxicity and the highest neuroprotection and MAO B inhibitory activity of 28%.
Introduction
The design of new biologically active substances has been largely oriented toward the development of hybrid molecules through the combination of different pharmacophores in one structure by linking the pharmacophoric fragments directly or with spacer agents [Citation1]. This strategy is gaining increasing attention, together with the assessment of hybrid molecules as a diverse range of drug candidates, pharmacological agents and as potent drugs [Citation2–4].
Semicarbazides and thiosemicarbazides are classes of compounds that belong to the urea and thiourea derivatives. Semicarbazides and thiosemicarbazides show high reactivity, which makes them valuable intermediates in the synthesis of important five-membered heterocycles [Citation5]. Some five-membered heterocycles, such as triazoles, thiadiazoles, oxadiazoles and thiazolidinones [Citation6], exhibit various pharmacological activities, including antifungal, anticancer, antibacterial, antimalarial, anticonvulsant, antitubercular, anti-proliferative, anti-inflammatory, antimicrobial, analgesic, antioxidant, antiviral and antipyretic properties [Citation7-–Citation13]. The conjugated N-N-S system of thiosemicarbazide is responsible for the manifestation of a strong inhibitory effect on lipoprotein levels and a significant strong blocking effect on DPPH radicals [Citation7]. Because they are small molecules, thiosemicarbazides can cross the blood–brain barrier and therefore be used as potential drugs in the treatment of neurodegenerative diseases. Some derivatives of this group are known to have some common characteristics such as H-bond acceptors, H-bond donors, hydrophobic substructures, positive ionizable atoms and aromatic rings that interact with MAO enzymes [Citation14,Citation15]. Thiosemicarbazide derivatives are of great interest not only as carriers of pharmacological activity, but also as a starting material for the preparation of various thiosemicarbazones, metal chelating complexes and anticorrosive agents [Citation5,Citation16–18]. Some recent studies have shown that semicarbazones, thiosemicarbazones and hydrazones together, are good MAO-B inhibitors in humans and rats with reversible mechanism of action and additional properties useful for CNS penetration and activity as multi target disease ligands [Citation19].
Based on the pharmacological relevance of methylxanthines and semi- and thiosemicarbazides, herein, we report a synthetic pathway to obtain theophylline-7-acetyl semi- and thiosemicarbazide hybrids by using the molecular hybridization strategy, as well as investigation on their in vitro neuroprotective and MAO-B inhibitory activities.
Materials and methods
Chemistry
All chemicals and solvents were purchased from Merck AG (Merck, Darmstadt, Germany). The chromatographic system for TLC control and purity elucidation is based on an aliminium sheets Silica gel F254 (Merck, Darmstadt, Germany), using the following mobile phases: Phase 1: Acetone/CHCl3/ethanol (3/3/4) and Phase 2: 25% NH4OH/Acetone/CHCl3/n-butanol (1/3/3/4), with detection at UV 254 nm. Yields were calculated for purified products. A Buchi 535 capillary apparatus (Switzerland) was used to determine the melting points. Infrared spectra were acquired by a Nicolet iS10 FT-IR Spectrometer using an ATR technique with a Smart iTR adapter. A Bruker Spectrospin WM250 spectrometer (Faenlanden, Switzerland) was used to acquire 1H-NMR and 13C-NMR spectra at 250 and 75 MHz, respectively. TMS as internal standard and DMSO-d6 were used as an internal standard and solvent, respectively, all OH and NH protons were D2O exchangeable. The coupling constants (J) are expressed in Hertz (Hz). A 6410 Agilent LCMS triple quadruple mass spectrometer (LCMS) with an electrospray ionization (ESI) interface was used to obtain the mass spectra. Microanalyses determined for C, N and H were within ± 0.4% of theoretical values and were performed on a Euro EA 3000-Single, EUROVECTOR SpA analyser. All names were generated by using the structure-to-name algorithm of the ChemBioDraw Ultra software, Version 11.0, CambridgeSoft.
Synthesis
Synthesis of methyl 2-(1,3-dimethyl-2,6-dioxo-2,3,6,7-tetrahydro-1H-purin-7-yl)acetate (2)
Theophylline (0.05 mol, 9 g) was dissolved in 140 mL of anhydrous methanol under stirring and heating. An equimolar amount of sodium methoxide was added to the solution, heated under reflux for 15 min and the solvent was removed in vacuo. The sodium salt obtained was dried in a vacuum desiccator over CaCl2 and after grinding, was transferred to a reaction flask with 100 mL of toluene. To the resulting suspension were added 2 and 3 drops of Aliquat 336 and methyl chloroacetate (0.1 mol, 8.8 mL). The reaction mixture was refluxed with vigorous stirring for 2 h (TLC in Systems 1 and 2). After cooling, it was filtered, evaporated to dryness and recrystallized from water/ethanol mixture.
Synthesis of2-(1,3-dimethyl-2,6-dioxo-2,3,6,7-tetrahydro-1H-purin-7-yl)acetohydrazide (3)
Hydrazine hydrate (0.05 mol, 2.5 mL) was added to a solution of methyl theophylline-7-acetate (2, 0.03 mol) in 50 mL of hot methanol. After 15 min, a voluminous precipitate is formed. The crude product was filtered after cooling. It is chromatographically pure and does not require recrystallization.
General procedure for syntheses of semicarbazides and thiosemicarbazides of 2-(1,3-dimethyl-2,6-dioxo-2,3,6,7-tetrahydro-1H-purin-7-yl)acetohydrazide (Citation4–13)
To a solution of 3 (0.004 mol) in 20 mL of DMF the corresponding isocyanate or isothiocyanate (0.005 mol) was added. The reaction mixture was heated to 50 °C on an electromagnetic stirrer for one hour, and then stirred at room temperature for a few more hours until the starting hydrazide was exhausted (TLC control, system 1 or 2). To the reaction mixture were added 60 mL of water, after standing in the refrigerator for several hours, the separated crystalline precipitate was filtered off and, if necessary, recrystallized from a suitable solvent. See the Supplemental data file.
Biological evaluation
Ethics statement
The vivarium (certificate of registration of farm No. 0072/01.08.2007) was inspected by the Bulgarian Drug Agency in order to check the husbandry conditions (No. A-11-1081/03.11.2011). All procedures were carried out in strict compliance with the requirements of the Institutional Committee for Animal Welfare and the principles set out in the European Convention for the Protection of Vertebrate Animals Used for Experimental and Other Scientific Purposes (ETS 123) (Council of Europe, 1991), throughout the experiment.
Animals
The experiments were performed on 6 male Wistar rats (body weight 200–250 g) which were housed in 2 organic glass cages with a 12/12 h light/dark cycle and standard laboratory conditions (ambient temperature 20° C ± 2° C and humidity 72% ± 4%) with free access to water and standard pelleted rat food 53-3 produced according to the ISO 9001:2008. The experimental animals were acquired from the National Breeding Center, Sofia, Bulgaria. Seven days of acclimatization were allowed before the commencement of the study, and a veterinary physician monitored the health of the animals regularly.
Isolation and incubation of rat brain synaptosomes and mitochondria
The synaptosomes and mitochondria were prepared by multiple, subcellular fractionation using a Percoll gradient [Citation20,Citation21]. Two types of buffers were prepared: Buffer A: HEPES 5 mmol/L and Sucrose 0.32 mol/L; Buffer B: NaCl 290 mmol/L, MgCl2.2H2O 0.95 mmol/L, KCl 10 mmol/L, CaCl2.2H2O 2.4 mmol/L, NaH2PO4 2.1 mmol/L, HEPES 44 mM, d-Glucose 13 mmol/L. Brain homogenates were prepared with buffer A and centrifuged at 1000g for 10 min at 4 °C. The removed supernatants were then centrifuged again under the above conditions. Supernatants were taken and subjected to three rounds of centrifugation at 10,000g for 20 min at 4 °C for purification of the synaptosomes.
Isolation of synaptosomes and mitochondria were performed with the help of a colloidal silicon solution (Percoll): (1). Preparation of 90% stock solution of Percoll. (2). Preparation of Percoll solutions with two concentrations 16% and 10%. 4 mL of Percoll 16% and 10% were added in the tubes. (3). 90% Percoll (7.5% Percoll) was added to the sediment from the last centrifugation. The tubes were centrifuged for 20 min at 15,000g at 4° C, resulting in the formation of three layers. The lower layer contained mitochondria; the upper layer, lipids; and the middle layer (between 16% and 10% Percoll), synaptosomes. The middle and the lower layers of each tube were harvested and buffer B with glucose was added. The mixture was centrifuged at 10,000 g for 20 min at 4 °C. After centrifugation, the sediment with the synaptosomes and mitochondria were mixed with buffer B with glucose.
Synaptosomal viability
After the incubation, the synaptosomes were centrifuged three times at 15,000 g for 1 min. MTT-test was performed to determine the synaptosomal vitality by the method described in the literature [Citation22].
Determination of reduced glutathione (GSH) in brain synaptosomes
The level of reduced glutathione was determined by measuring the non-protein SH-groups after precipitation of the proteins with trichloro acetic acid. After the incubation, synaptosomes were centrifuged at 400g for 3 min. The sediment was treated with 5% trichloro acetic acid and left for 10 min on ice. Samples were centrifuged at 8000g for 10 min (2 °C). The supernatant was removed to determine the level of GSH and was stored at −20 °C. Immediately before the measurement, the samples were neutralized with 5 N NaOH.
The presence of thiols in the supernatant was determined using Elmman reagent. The resulting yellow color was measured spectrophotometrically at λ = 412 nm [Citation23].
Model of 6-OHDA-induced neurotoxicity in synaptosomes
This in vitro model resembles the neurodegenerative processes occurring in PD. Dopamine metabolism and oxidation lead to the formation of reactive oxygen species (ROS) and reactive quinones. They induce dopamine neurotoxicity and neurodegeneration [Citation24]. The synaptosomes were incubated with 150 μmol/L 6-OHDA for 1 h [Citation25].
Tert-butyl hydroperoxide (t-BuOOH)-induced oxidative stress in isolated brain mitochondria
The mitochondria were incubated with 75 µmol/L t-BuOOH [Citation26].
Lipid peroxidation assay in brain mitochondria
We applied 0.3 mL 0.2% TBA and 0.25 mL sulfuric acid (0.05 mol/L) for incubation of mitochondrial suspensions (0.5 mg protein/mL) [Citation27]. Then, 30 min after that, the tubes were maintained in a bath filled with boiling water. Finally, the tubes were transferred to an ice bath and butanol (0.4 mL) was poured into each tube. In the next stage, centrifugation of the tubes was carried out at 3 500 g for 10 min. Following that, assessment of the total quantity of MDA in each sample was carried out using a spectrophotometer (UV–Vis Split) to measure the supernatant absorbance at 532 nm.
Measurement of GSH content in brain mitochondria [Citation27]
DTNB was applied by the spectrophotometric technique to determine the GSH contents in isolated brain mitochondria. Then, 0.04% DTNB was mixed with the mitochondrial suspensions (0.5 mg protein/mL) in 0.1 mol/L of phosphate buffers (pH 7.4). The development of the yellow color was read at 412 nm (spectrophotometer UV–Vis Split).
Preparation of brain microsomes using ultracentrifugation
The brain was homogenized in 9 vol of 0.1 mol/L Tris containing: 0.1 mmol/L dithiothreitol, 0.1 mmol/L phenylmethylsulfonyl fluoride, 0.2 mmol/L EDTA, 1.15% potassium chloride and 20% (v/v) glycerol at pH 7.4 [Citation28]. The homogenate was centrifuged at 17,000g for 30 min. The pellet was suspended in 4 vol of the homogenization buffer and centrifuged at 17,000g for a further period of 30 min. The supernatants from both centrifugations were combined (post-mitochondrial supernatant) and centrifuged at 100,000g for 1 h. The pellet was resuspended in the above buffer and centrifuged at 100,000g for 1 h. The resulting pellet was frozen in the buffer with glycerol.
FeSO4/ascorbic acid-induced lipid peroxidation in isolated brain microsomes
The microsomes were pre-incubated with the analyzed compounds for 15 min at 37 °C. The reaction was started with a solution of iron sulphate 20 μmol/L and ascorbic acid 0.5 mmol/L. The reaction was stopped with a mixture of TCA 25% and TBA 0.67% at 20 min. After starting the reaction, the LPO and the MDA quantity were assessed [Citation29].
MDA assay in brain microsomes
The quantity of the lipid peroxidation product MDA was assessed using the method described in the literature [Citation29]. The absorbance was measured at 535 nm, and the amount of MDA was calculated using a molar extinction coefficient of 1.56 × 105 L mol−1 cm−1.
Measurement of monoamine oxidase B activity
Monoamine oxidase activity assay of recombinant human MAO-B was performed using a fluorimetric method by Amplex Ultra Redreagent [Citation30]. Tyramine hydrochloride was used as substrate.
Statistical analysis
The MAO-B activity was normalized as percentage of the untreated control set as 100% and the results were expressed as mean values and standard deviation (±SD). Statistical analysis was performed by one-way analysis of variance (ANOVA) with post hoc multiple comparisons procedure (Dunnet’s test) to assess the statistical differences in case of normal distribution. Differences were considered statistically significant at the levels of p < 0.05, p < 0.01 and p < 0.001.
Statistical analysis of the results obtained from brain microsomes, synaptosomes and mitochondria were performed using statistical programme “MEDCALC”. The results are expressed as mean values with standard error of the means (± SEM) for six experiments. The significance of the data was assessed using the non-parametric Mann–Whitney test. Differences were considered statistically sig nificant at the levels of p < 0.05, p < 0.01 and p < 0.001.
Results
Chemistry
To obtain the hydrazides of (theophyllin-7-yl)-acetic acid and their semi- and thiosemicarbazide derivatives, we applied a synthetic approach based on the gradual construction of the side chain (Scheme 1).
Scheme 1. Synthesis of semi- and thiosemicarbazides of 7-theophylline acetic acid [Citation4–13].
![Scheme 1. Synthesis of semi- and thiosemicarbazides of 7-theophylline acetic acid [Citation4–13].](/cms/asset/ab2da0a5-67d9-46ea-b71c-ca556ffca36a/tbeq_a_2098819_sch0001_b.jpg)
The sodium salt of theophylline, prepared with sodium methoxide in anhydrous methanol [Citation30,Citation31], does not require further purification and was not isolated, but was directly used in the next step of reaction with methyl chloroacetate.
The reaction can take place in absolute ethanol, with protection from atmospheric moisture. The yield of the isolated product was about 55% at a reaction time of 10 h. The significant amount of the losses is due to the hydrolysis of the ester, occurring during the isolation.
To increase the yield of the products, we performed the synthesis in a two-phase system of the type solid (sodium salt of theophylline)/liquid (benzene, toluene or methylene chloride) in the presence of phase-transfer catalysts (PTC) at a molar ratio of reactants 1/2. As catalysts we used quaternary ammonium salts Aliquat 336, TOAB (tetraoctadecylammonium bromide), as well as crown ethers 18-Crown-6 and Dibenzo-18-Crown-6.
The best results were obtained using quaternary ammonium salts in a medium of toluene and benzene, and in addition to the type of catalyst, the reaction temperature is also important. The advantage of the applied approach is the reduction in the reaction time and the higher final yield for the isolated product compared to the starting theophylline. Hydrazide derivative 3 is prepared by hydrazinolysis of ester 2 with hydrazine hydrate at a yield of 98% in ethanol for 20 min.
The preparation of the target compounds 4–13 is accomplished by reacting the hydrazide 3 with a suitable isocyanate or isothiocyanate according to Scheme 1. The type of the heteroatom Z and the substituent R are shown in .
Table 1. ID, structures and yields of newly synthesized compounds.
The results of this synthesis are summarized in .
Table 2. Yields of the isolated product of 2 under conditions of phase-transfer catalysis.
The reaction was carried out at a molar ratio of reactants of 1:1 to 1.25 in dimethylformamide medium at a temperature of 40–50 °C or water/hydrochloric acid at reflux. The reaction times were determined by TLC – monitoring of depletion of the starting hydrazide. The yields and reaction times in the corresponding medium for compounds 4−13 are presented in .
Neurotoxicity evaluation
Effects of the studied compounds on synaptosomal viability in isolated rat brain synaptosomes
Synaptosomal viability was determined by the MTT assay [Citation31], with test compounds administered at a concentration of 100 μmol/L and the results were compared with untreated control cells. A Percoll gradient was used to isolate synaptosomes as described in [Citation19], and two approaches were used sequentially to determine the toxicity of the newly obtained semi- and thiosemicarbazides at the subcellular level: assessment of synaptosomal viability by MTT test and determination of reduced glutathione levels.
On isolated brain synaptosomes, most of the tested compounds [Citation4–13], hydrazide [Citation3] and theophylline [Citation1] did not show a statistically significant neurotoxic effect. Only compounds 8, 10 and 13 had toxicity, compared to untreated synaptosomes used as control ().
Figure 1. Effect of compounds 1, 3, 4 - 13 administered alone at a concentration of 100 µmol/L on synaptosomal viability. * р<0.05 compared to control (untreated synaptosomes).
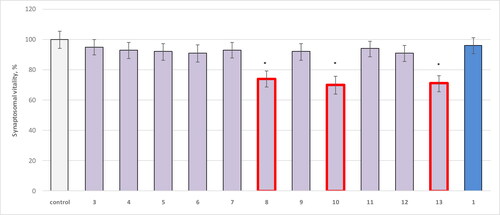
The results showed that 8 decreased the synaptosomal viability statistically significantly by 26%, 10 by 30%, and 13 by 29% compared to the control.
Effects on reduced glutathione (GSH) levels in isolated rat synaptosomes
According to this parameter on isolated brain synaptosomes, most of the tested compounds 4-13, hydrazide (Citation3) and theophylline (Citation1) did not show a statistically significant neurotoxic effect. Only compounds 8, 10 and 13 had toxicity compared to control (untreated synaptosomes) ().
Figure 2. Effect of compounds 1, 3, 4–13 administered alone at a concentration of 100 µM on GSH levels in isolated synaptosomes. **р<0.01 relative to control (untreated synaptosomes).
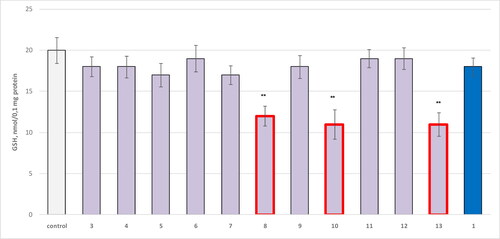
The results showed that 8 reduced the GSH levels, statistically significantly, by 40%; 10 by 45% and 13 by 45% compared to the control.
Effects on isolated brain microsomes
The majority of the test compounds and theophylline (administered as a control) did not show a statistically significant neurotoxic effect. Only compounds 8, 10 and 13 were toxic compared to controls (untreated microsomes) ().
Figure 3. Effect of compounds 1, 3 and 4–13, applied alone at a concentration of 100 µM, on the production of MDA in isolated microsomes. **р<0.01 relative to control (untreated microsomes).
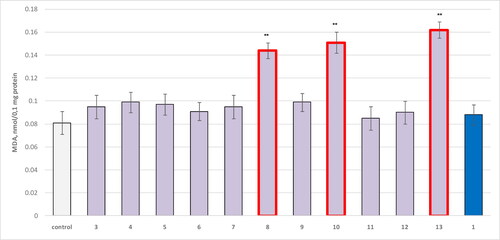
The results showed that three compounds increased MDA production statistically significantly compared to controls: 8 by 78%; 10 by 86% and 13 by 100%.
Effects on isolated brain mitochondria
Compounds 1, 3 and 4–13 were subjected to an in vitro evaluation of their effects on isolated rat brain mitochondria using two parameters: GSH levels and MDA production. The obtained results are presented in and , respectively.
Figure 4. Effect of compounds 1, 3, 4–13 administered alone at a concentration of 100 µmol/L on GSH levels in isolated mitochondria. **р<0.01 compared to control (untreated mitochondria).
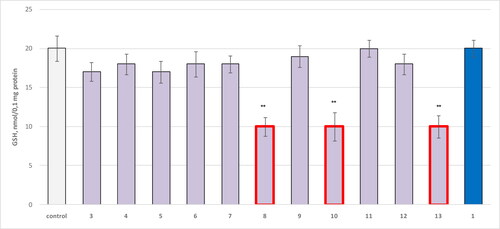
Figure 5. Effect of compounds 1, 3 and 4–13, applied alone at a concentration of 100 µmol/L, on the production of MDA in isolated mitochondria. **р<0.01 compared to control (untreated mitochondria).
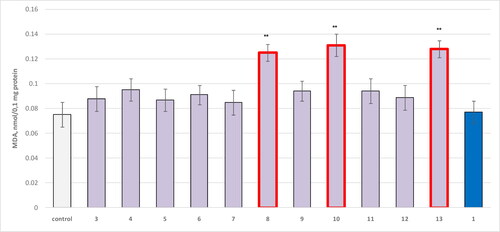
The evaluation of GSH levels in isolated rat mitochondria confirmed the high neurotoxicity of structures 8, 10 and 13, with all three being associated with statistically significant reduction in GSH levels by 50% ().
Again, there was a slight increase in the neurotoxicity determined by MDA production for compound 13, in comparison to 8, where 13 increased the MDA levels by 71%, while 8 by 67%. The most toxic structure based on this parameter was compound 10, increasing the MDA production by 75% ().
Evaluation of the neuroprotective activity of newly obtained derivatives
The protective activity of the newly synthesized compounds was studied in various models of oxidative damage at the subcellular level in brain synaptosomes, microsomes and mitochondria.
Protective effects on synaptosomal viability
In the 6-OHDA-induced oxidative stress model, only compound 5 and theophylline 1 showed a statistically significant neuroprotective effect compared to the toxic agent (). As seen, 6-OHDA showed a visible neurotoxic effect by reducing synaptosomal viability by 55% compared to controls (untreated synaptosomes).
Figure 6. Effect of compounds 1, 3, 4–13 (100 µmol/L), in a model of 6-OHDA-induced oxidative stress, on synaptosomal viability in isolated synaptosomes. ***p < 0.001 compared to control (untreated synaptosomes); ++p < 0.01 compared to 6-OHDA.
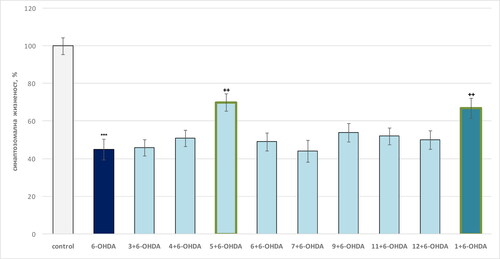
On this background, compound 5 retained synaptosomal viability by 56% relative to the toxic agent, and pure theophylline by 49% relative to 6-OHDA ().
Protective effects on reduced glutathione (GSH) levels in isolated rat synaptosomes
In the 6-OHDA-induced oxidative stress model, the only compound 5 and theophylline 1 showed a statistically significant neuroprotective effect compared to the toxic agent: 5 retained the GSH level by 60% relative to the levels of GSH after treatment with 6-OHDA. Pure theophylline preserved the GSH levels to 50% relative to 6-OHDA, while 6-OHDA administered alone exhibited a pronounced neurotoxic effect by reducing the GSH levels by 50% relative to controls (untreated synaptosomes) ().
Protective effects in an iron/ascorbate (Fe2+/AA) model of non-enzyme-induced lipid peroxidation in isolated rat microsomes
In this model of non-enzyme-induced lipid peroxidation of the test compounds, the only compound 5 and theophylline showed a statistically significant neuroprotective effect against the toxic agent. On its own, iron ascorbate (Fe/AA) had a pronounced pro-oxidant effect by increasing MDA production by 415% compared to the control (untreated microsomes), whereas 5 reduced the MDA production by 50%, relative to the toxic agent. Theophylline reduced MDA by 47% compared to Fe2+/AA ().
Protective effects in a model of tert-butyl hydroperoxide (t-BuOOH)-induced oxidative stress in isolated rat brain mitochondria
The in vitro protective effects of the test compounds in the t-BuOOH-induced oxidative stress model in isolated rat brain mitochondria were investigated by considering two parameters: the GSH levels and MDA production. The results from the performed evaluations are presented in and .
Figure 9. Effect of compounds 1, 3 and 4–13 (100 µmol/L), in a model of t-BuOOH-induced oxidative stress, on MDA production in isolated brain mitochondria. ***p < 0.001 compared to control (untreated mitochondria); ++p < 0.01 compared to t-BuOOH.
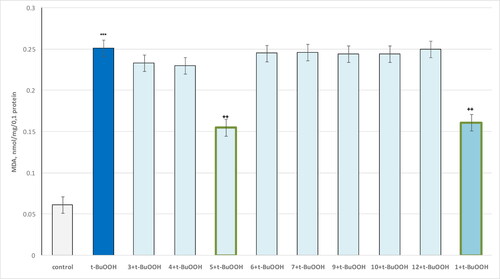
Figure 10. Influence of substances 1, 3 and 4–13 (100 µmol/L), in a model of t-BuOOH-induced oxidative stress, on the GSH level in isolated brain mitochondria. ***p < 0.001 compared to control (untreated mitochondria); ++p < 0.01, compared to t-BuOOH.
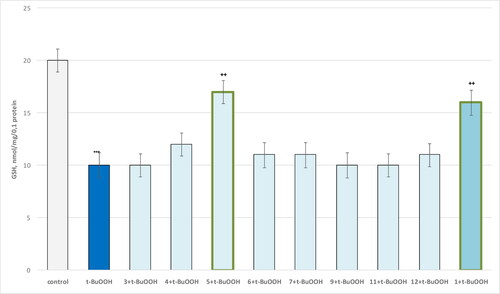
The results show that the only compound 5 and theophylline 1 show a statistically significant neuroprotective effect against the toxic agent. t-BuOOH administered alone showed a pronounced neurotoxic effect by increasing MDA production by 311% and reducing GSH levels by 50% compared to the control (untreated mitochondria). In this neurotoxicity model, compound 5 reduced MDA production by 38% and maintained GSH levels by 70% relative to the toxic agent. Pure theophylline reduced MDA production and maintained GSH levels by 36% and 60%, respectively, relative to pure t-BuOOH ( and , respectively).
Evaluation of the influence of the newly obtained products on the activity of the human recombinant MAO-B enzyme (hMAOB)
For the purposes of the investigation, the test substances were administered at a concentration of 1 µmol/L, the effects were compared with the control (pure hMAOB) and with the effects of selegiline (R-deprenyl) – a classic MAO-B inhibitor, which is used in the treatment of Parkinson’s disease (PD).
When used alone, only compound 5 and theophylline showed a statistically significant inhibitory effect on hMAOB enzyme activity. This effect was close to that of the classic MAO-B inhibitor selegiline, with 5 inhibiting the enzyme by 28%, theophylline by 25%, and selegiline by 45% ().
Figure 11. Influence of a series of compounds 3, 4–13, theophylline [Citation1] and selegiline, administered alone, on the activity of hMAOB. **p < 0.01;***p < 0.001 compared to the control (pure hMAOB).
![Figure 11. Influence of a series of compounds 3, 4–13, theophylline [Citation1] and selegiline, administered alone, on the activity of hMAOB. **p < 0.01;***p < 0.001 compared to the control (pure hMAOB).](/cms/asset/eb3e180f-3acf-4716-940c-28b06c2aa9f2/tbeq_a_2098819_f0011_c.jpg)
Discussion
Chemistry
The corresponding yields for the newly synthesized products vary between 55 and 99% with reaction times between 4 and 15 h. The obtained products are colorless (white) to pale yellow crystalline compounds, insoluble in water and polar solvents, soluble in DMF and DMSO. The structures of the newly obtained compounds were confirmed by data from FTIR, 1H-NMR, UV and LS – MS spectroscopy and are presented in the Materials and methods section for each product.
The values of the chemical shifts of the protons registered by 1H NMR spectra were compared with values simulated using SPINUS-WEB server [Citation32–Citation34]. There were some deviations of the theoretical values from the experimental values in the 1H NMR spectra of the tested compounds. They are probably due to the impossibility to take into account the influence of the solvent. Nevertheless, the simulated and the calculated data are in good correlation.
Regardless of the observed small deviations of the values computed using a web-based tool [Citation32,Citation33,Citation35] from the experimental values, the simulated 13C NMR spectra are in good correlation with the real ones.
Neurotoxicity evaluation
Effects on isolated rat brain synaptosomes
Synaptosomes are a subcellular fraction isolated from brain tissue by homogenation followed by fractionation based on size or density. They have a large part of the released postsynaptic formations mixed with the presynaptic part of the synapses.
Synaptosomes are an important source of information about neurotransmitters, cell membrane polarization and ion exchange. This largely allows their use as an in vitro system for assessing the degree and rate of release of neurotransmitters in the CNS. In addition, they are a commonly used approach in drug evaluation at the subcellular level.
Effects of the studied compounds on synaptosomal viability in isolated rat brain synaptosomes
The analysis of the obtained data identified most of the target structures to show low cytotoxic effect on both evaluated parameters. One of the main indicators providing preliminary information on the presence of subcellular toxicity is the level of reduced glutathione in the synaptosomal fraction. The data obtained from this indicator confirm the results of the synaptosomal viability studies, indicating that the tested compounds are low toxic, with 8, 10 and 13 showing the highest toxicity. The least toxic compounds from this series were further subjected to in vitro neurotoxicity screening on isolated rat microsomes.
Effects on isolated brain microsomes
The brain has become one of the most studied organs due to the possible pharmacological and toxicological effects of drug metabolism on it. Microsomes are the most suitable model for studying the drug metabolism and its influence on the brain. They are small sealed vesicles that originate from fragmented cell membranes (often the endoplasmic reticulum) and contain specific individual human metabolizing enzymes, such as cytochrome (CYP) and uridine-5′-diphosphoglucuronosyl transferase (UGT). This makes them extremely important models for in vitro studies of drug metabolism, in particular for the study of the contribution of enzymes involved in the biotransformation of drugs and xenobiotics.
Based on the above, we investigated the presence of a probable toxic effect on isolated rat brain microsomes. The effect of selected newly synthesized molecules on the production of malonaldehyde (MDA) in isolated brain microsomes was used as a quantitative parameter for the manifestation of neurotoxicity. It is also observed that on this parameter the replacement of the O-atom [Citation8] with S-atom [Citation13] in the side chain results in an increase of the MDA production from the structures [Citation8,Citation13] evaluated in the study.
Effects on isolated brain mitochondria
Derivatives 8, 10 and 13 showed the highest neurotoxicity on all evaluated subcellular fractions and parameters. The remaining compounds, the parent hydrazide and theophylline did not show statistically significant neurotoxic effects on isolated subcellular fractions, including brain synaptosomes, microsomes and mitochondria. In the compounds of the studied series, the presence of an aliphatic chain of two or more carbon atoms in the side chain [Citation10] and/or the replacement of the aromatic nucleus (8 and 13) leads to increased neurotoxicity, which we believe is due to increased lipophilicity of longer chain saturated substitutes. The obtained results also demonstrate that the replacement of the O-atom in the semicarbazide fragment of 8 with S-atom in the thiosemicarbazide representative 13 does not affect the neurotoxicity noticeably.
Studies on the neuroprotective activity of newly obtained derivatives
Neuroprotection is a process of relative preservation of neural structure and/or function [Citation36]. It aims to prevent or slow down the progression of disease or the subsequent damage by preventing or slowing down the loss of neurons [Citation37]. Despite the differences in symptoms and damage associated with CNS disorders, many of the mechanisms of neurodegeneration are similar. These include oxidative stress, mitochondrial dysfunction, excitotoxicity, inflammatory changes, iron accumulation and protein aggregation [Citation37–Citation39].
GSH depletion, disorders of cellular metabolism and cell death are known to be major mechanisms associated with oxidative and free radical damage in neuronal tissue. Treatment with exogenous inducers of oxidative stress, such as 6-hydroxydopamine (6-OHDA), tert-butylhydroperoxide (t-BuOOH) and iron ascorbate (Fe/AA) make it possible to model oxidative damage and to study the protective effects of substances with antioxidant activity.
Protective effects on reduced glutathione (GSH) levels in isolated rat synaptosomes
It should be noted that the introduction of semi- and thiosemicarbazide fragment on the seventh position of the theophylline core affects the neuroprotective properties of the initial xanthine, where the appearance of a single methyl group in the thiosemicarbazide side chain of 5 alters the neuroprotective effect by a slight increase.
Protective effects in an iron/ascorbate (Fe2+/AA) model of non-enzyme-induced lipid peroxidation in isolated rat microsomes
Unsaturated fatty acids in the cell membrane are particularly sensitive to the action of ROS. Therefore, lipid hydroperoxides alter membrane density and membrane protein function. Lipid hydroperoxides, in turn, can undergo iron-mediated, one-electron reduction and oxidation to form epoxyallyl peroxide radicals to trigger lipid peroxidation processes. The end-products of lipid peroxidation are reactive aldehydes (e.g. 4-hydroxynonenal and malondialdehyde), which are toxic to the cell. A widely used experimental model for oxidative stress is the Fe2 +/AA-induced lipid peroxidation model. The formation of reactive-free radicals alters membrane integrity by inducing lipid peroxidation [Citation40,Citation41].
It should be mentioned that in this model the removal of the alkyl substituent in the semi- and thiosemicarbazide fragment (11 and 12) decreases the neuroprotective activity in the same manner as the introduction of an aromatic [Citation4], substituted aromatic (6, 7 and 9), cycloalkane (8 and 13) and ethyl [Citation10] radical.
Protective effects in a model of tert-butyl hydroperoxide (t-BuOOH)-induced oxidative stress in isolated rat brain mitochondria
The performed experiments on the neuroprotective properties of the target new theophylline semi- and thiosemicarbazide derivatives identified the initial theophylline and compound 5 as the most promising agents, showing comparable neuroprotective effects on all evaluated parameters on all tested models of induced oxidative stress.
Evaluation of the influence of the newly obtained products on the activity of the human recombinant MAO-B enzyme (hMAOB)
The obtained results identified that the presence of a methyl group in the thiosemicarbazide fraction is promising for the performance of MAO-B inhibitory activity in the series of evaluated semi- and thiosemicarbazide derivatives of theophylline.
Conclusions
The performed biological tests with 11 newly synthesized compounds show that compound 5 stands out as the most promising for future in vivo research and a leader in the design of new xanthine derivatives affecting the neurodegenerative pathologies. The obtained results can be used as initial information in the future design of analogous derivatives, with potential hMAOB inhibitory activity and pronounced neuroprotection in case of low neurotoxicity.
Authors’ contributions
Javor Mitkov – contributed to the synthesis of the evaluated compounds.
Magdalena Kondeva-Burdina – contributed to the neurotoxicity evaluation and MAO B determination.
Lily Peikova – contributed to the spectral identification of the compounds.
Maya Georgieva – contributed to the synthesis and neuroprotection evaluation.
Alexander Zlatkov – contributed to the initiation of the scientific idea, composing the manuscript and spectral data evaluation.
Supplemental Material
Download PDF (147.7 KB)Acknowledgements
The author thanks Assoc. Prof. Dr. Paraskev Nedyalkov for his help in recording the MS spectra of the newly obtained compounds.
Data Availability Statement
The data used to support the findings of this study are included within the article and Supplementary material.
Disclosure statement
No potential conflict of interest was reported by the author(s).
Funding
The author(s) reported there is no funding associated with the work featured in this article.
References
- Bosquesi PL, Melo TRF, Vizioli EO, dos Santos JL, Ching MC. Anti-inflammatory drug design using a molecular hybridization approach. Pharmaceuticals. 2011;4:1450–1474.
- Kumar HMS, Herrmann L, Tsogoeva SB. Structural hybridization as a facile approach to new drug candidates. Bioorg Med Chem Lett. 2020;30:127514.
- Ivasiv V, Albertini C, Goncalves AE, Rossi M, Bolognesi ML. Molecular hybridization as a tool for designing multitarget drug candidates for complex diseases. Curr Top Med Chem. 2019;19:1694–1711.
- Mishra SS, Sing P. Hybrid molecules: the privileged scaffolds for various pharmaceuticals. Eur J Med Chem 2016;124:500–536.
- Mobinikhaledi A, Foroughifar N, Kalhor M, Ebrahimi S, Fard MB. Synthesis of some symmetrical novel bis-thiosemicarbazides, 1,2,4-triazoles, 1,3,4-thiadiazoles, and their derivatives. Phosphorus Sulfur Silicon. 2010;186:67–73.
- Hu K, Yang Z, Pan S-S, Xu H, Ren J. Synthesis and antitumor activity of liquiritigenin thiosemicarbazone derivatives. Eur J Med Chem. 2010;45:3453–3458.
- Pachuta-Stec A, Rzymowska J, Mazur L, Mendyk E, Pitucha M, Rzaczynska Z. Synthesis, structure elucidation and antitumour activity of N-substituted amides of 3-(3-ethylthio-1,2,4-triazol-5-yl)propenoic acid. Eur J Med Chem. 2009; 44(9): 3788–3793.
- Pitucha M, Mazur L, Kosikowska U, Pachuta-Stec A, Malm A, Popiolek K, et al. Synthesis, structure, and antibacterial evaluation of new N-substituted-3-amino-5-oxo-4-phenyl-2,5-dihydro-1H-pyrazole-1-carbothioamides. Heteroat Chem 2010;21(4):215–221.
- Ghosh S, Misra AK, Bhatia G, Khan MM, Khanna AK. Syntheses and evaluation of glucosyl aryl thiosemicarbazide and glucosyl thiosemicarbazone derivatives as antioxidant and anti-dyslipidemic agents. Bioorg Med Chem Lett. 2009;19 386–389.
- Chłoń-Rzepa G, Jankowska AW, Zygmunt M, Pociecha K, Wyska E. Synthesis of 8-alkoxy-1,3-dimethyl-2, 6-dioxopurin-7-yl-substituted acetohydrazides and butanehydrazides as analgesic and anti-inflammatory agents. Heterocycl Commun. 2015;21(5):273–278.
- Mathew GE, Oh JM, Mohan K, Tengli A, Mathew B, Kim H. Development of methylthiosemicarbazones as new reversible monoamine oxidase-B inhibitors for the treatment of Parkinson’s disease. J Biomolec Struct Dyn. 2021;39(13):4786–4794.
- García-Ramírez VG, Suarez-Castro A, Villa-Lopez MG, Díaz-Cervantes E, Chacón-García L, Cortes-García CJ. Synthesis of novel acylhydrazone-oxazole hybrids and docking studies of SARS-CoV-2 main protease. ChemProc. 2021;3(1):2–6.
- Vilar S, Ferino G, Quezada E, Santana L, Friedman C. Predicting monoamine oxidase inhibitory activity through ligand-based models. Curr Top Med Chem. 2012;12(20):2258–2274.
- Azam F, Alksakas IA, Khokra SL, Prakash O. Synthesis of some novel N4-(naphtha[1,2-d]thiazol-2yl)semicarbazides as potential anticonvulsant. Eur J Med Chem. 2009;44:203–211.
- Salah BA, Kandil AT, Abd El-Nasser MG. A therapeutic journey of semicarbazide and thio semicarbazide derivatives and their transition metals complexes: mini review. Res Rev J Chem. 2018;7(1):38–48.
- Yogeeswari P, Sriram D, Saraswat V, Ragavendran JV, Kumar MM, Murugesan S, et al. Synthesis and anticonvulsant and neurotoxicity evaluation of N4-phtalimido phenyl(thio)semicarbazides. Eur J Pharm Sci. 2003;20:341–346.
- Garg BS, Jain VK. Analytical applications of thiosemicarbazones and semicarbazones. Microchem J. 1988;38:144–169.
- Guglielmi P, Carradori S, Ammazzalorso A, Secci D. Novel approaches to the discovery of selective human monoamine oxidase-B inhibitors: is there room for improvement? Exp Opin Drug Discov. 2019:1–41.
- Peikov P, Sidzhakova D, Gagausov J. Laboratory technology of synthesis of 1-(3-iodoropropyl)-3,7-dimethylxanthine. Pharmacia (Sofia). 1988;38:1–4.
- Andrés M., Castillo LP, Wist J. Fast and accurate algorithm for the simulation of NMR spectra of large spin systems. J Magn Reson. 2011;209:123–130.
- Banfi D, Patiny L. www.nmrdb.org: resurrecting and processing NMR spectra on-line. Chimia. 2008;62(4):280–281.
- Aires-de-Sousa J, Hemmer M, Gasteiger J. Prediction of 1H NMR chemical shifts using neural networks. Anal Chem. 2002;74(1):80–90.
- Steinbeck C, Krause S, Kuhn S. NMRShiftDB constructing a free chemical information system with open-source components. J Chem Inform Comput Sci. 2003;43(6):1733–1739.
- Taupin P, Zini S, Cesselin F, Ben-Ari Y, Roisin MP. Subcellular fractionation on Percoll gradient of Mossy fiber synaptosomes: morphological and biochemical characterization in control and degranulated rat hippocampus. J Neurochem 1994;62 1586–1595.
- Mosmann T. Rapid colorimetric assay for cellular growth and survival: application to proliferation and cytotoxicity assays. J Immunol Methods. 1983;65:55–63.
- Casson RJ, Chidlow G, Ebneter A, Wood JPM, Crowston J, Goldberg I. Translational neuroprotection research in glaucoma: a review of definitions and principles. Clin Exp Ophthalmol. 2011;40:350–357.
- Seidl SE, Potashkin JA. The promise of neuroprotective agents in Parkinson’s disease. Front Neurol. 2011; 2:art. no. 68.
- Andersen JK. Oxidative stress in neurodegeneration: cause or consequence? Nat Med 2004;10:S18–S25.
- Dunnett SB, Björklund A. Prospects for new restorative and neuroprotective treatments in Parkinson’s disease. Nat Med. 1999;399:A32–A39.
- Gáspárová Z, Ondrejičková O, Gajdošíková A, Gajdošík A, Šnirc V, S. Š. Oxidative stress induced by the Fe2+/ascorbic acid system or model ischemia in vitro: effect of carvedilol and pyridoindole antioxidant SMe1EC2 in young and adult rat brain tissue. Interdiscip Toxicol. 2010;3:122–126.
- Yara S, Lavoie J-C, Beaulieu J-F, Delvin E, Amre. D., Marcil V, et al. Iron-ascorbate-mediated lipid peroxidation causes epigenetic changes in the antioxidant defense in intestinal epithelial cells: impact on inflammation. PLoS One. 2013;8:e63456.
- Sims NR, Anderson MF. Isolation of mitochondria from rat brain using Percoll density gradient centrifugation. Nat Protoc 2008;3(7):1228–1239.
- Mungarro-Menchaca X, Ferrera P, Moran J, Arias C. Beta-amyloid peptide induces ultrastructural changes in synaptosomes and potentiates mitochondrial dysfunction in the presence of ryanodine. J Neurosci. 2002; 68 89–96.
- Robyt F, Ackerman RJ, Chittenden CG. Reaction of protein disulfide groups with Ellman’s reagent: a case study of the number of sulfhydryl and disulfide groups in Aspergillus oryzae - amylase, papain, and lysosome. Arch Biochem Biophys. 1971;147:262–269.
- Stokes AH, Freeman WM, Mitchell SG, Burnett TA, Hellmann GM, Vrana KE. Induction of GADD45 and GADD153 in neuroblastoma cells by dopamine-induced toxicity. Neurotoxicology 2002;23:675–684.
- Kondeva-Burdina M, Voynova M, Aluani D, Tzankova V, Krasteva I. Effects of amanita muscaria extract on different in vitro neurotoxicity models at sub-cellular and cellular levels. Food ChemToxicol. 2019;132:110687.
- Karlsson J, Emgard M, Brundin P, Burkitt MJ. Trans-resveratrol protects embryonic mesencephalic cells from tert-butylhydroperoxide: electron paramagnetic resonances pintrapping evidence for radical scavenging mechanism. J Neurochem. 2000;75(1):141–150.
- Shirani M, Alizadeh S, Mahdavinia M, Dehghani MAMd. The ameliorative effect of quercetin on bisphenol A-induced toxicity in mitochondria isolated from rats. Environ Sci Pollut Res Int. 2019 26(8):7688–7696.
- Ravindranath V, Anandatheerthavarada HK. Preparation of brain microsomes with cytochrome P450 activity using calcium aggregation method. Anal Biochem. 1990 187(2):310–313.
- Mansuy D, Sassi A, Dansette PM, Plat M. A new potent inhibitor of lipid peroxidation in vitro and in vivo, the hepatoprotective drug anisyldithiolthione. Biochem Biophys Res Commun. 1986;135(3):1015–1021.
- Bautista-Aguilera OM, Esteban G, Bolea I, Nikolic K, Agbaba D, Moraleda I, et al. Design, synthesis, pharmacological evaluation, QSAR analysis, molecular modeling and ADMET of novel donepezil-indolyl hybrids as multipotent cholinesterase/monoamine oxidase inhibitors for the potential treatment of Alzheimer’s disease. J Eur J Med Chem. 2014;75:82–95.