Abstract
Atherosclerosis is the pathological basis of cardiovascular and cerebrovascular diseases. Nelumbo nucifera Gaertn. leaves are edible, an effective traditional Chinese medicine in the treatment of atherosclerosis, but its mechanisms of action remain unclear. In this study, we employed network pharmacology and molecular docking approaches to explore it, and preliminarily verify it through animal experiments. Firstly, we obtained the active ingredients of N. nucifera leaves and their potential targets in several databases. Comparing with the atherosclerosis drug targets, we acquired their common targets by Venny 2.1.0 software, with which, a protein–protein interaction (PPI) network was then constructed. Then, we performed enrichment analysis of GO functions and KEGG pathways by Metascape. Molecular docking was also carried out between the active ingredients and their core targets to test their binding activity. Lastly, animal experiments with Apoe-/- mice were performed to initially verify the efficacy of N. nucifera leaves in treating atherosclerosis. As a result, a total of 16 major active ingredients of N. nucifera leaves and 219 potential targets were identified, and 11 core targets were obtained from the PPI network. The molecular docking results showed stronger binding activity of these core targets with the major active ingredients. The animal experiments indicated that N. nucifera leaves could effectively improve atherosclerosis by lowering the levels of lipids and inflammatory factors. Accordingly, our present study provided experimental foundation for the underlying mechanisms and clinical application in treating atherosclerosis via N. nucifera leaves. Further work still needs to be performed to verify them in future experiments.
Introduction
Atherosclerosis (AS) refers to the accumulation of fatty and/or fibrous material in the innermost layer of arteries, the intima, causing morbidity and mortality worldwide, including most myocardial infarctions, strokes and disabling peripheral artery diseases [Citation1]. It is also considered to be a lipid -driven inflammatory disease of the arterial intima, where the balance of pro-inflammatory and inflammation-resolving mechanisms dictates the final clinical outcomes [Citation2]. Today, atherosclerosis has become a major cause of mortality on a global scale [Citation1]. Atherosclerotic cardiovascular diseases (ASCVDs), including coronary heart disease, hypertension and stroke, have been the leading cause of cardiovascular and cerebrovascular diseases.
In general, the pathogenesis of atherosclerosis can be considered in three phases: initiation, progression and complications. Mass evidence confirmed that the key initiating event in atherogenesis is the retention of low-density lipoprotein cholesterol (LDL-C) and other cholesterol-rich apolipoprotein B-containing lipoproteins within the arterial wall [Citation3]. The cumulative exposure of an artery to LDLC over years remains a principal determinant of disease initiation and progression [Citation1, Citation3]. In addition, other risk factors in atherogenesis included hypertension, diabetes mellitus, cigarette smoking, obesity, unhealthy diet, physical inactivity and so on. New evidence also points to a role of the immune system, as emerging risk factors including inflammation and clonal haematopoiesis [Citation1]. Particularly, in 2017, the importance of anti-inflammation therapies in atherosclerosis was confirmed by the CANTOS trial [Citation4]. Today, the epidemiological data have yet shown that ASCVDs collectively comprise the No.1 cause of death globally, in which, more than 75% of deaths occur in low- and middle-income countries, putting a heavy burden on the economies of these countries [Citation5, Citation6].
At present, statins are still the first-line drugs of treatment for atherosclerosis, whose mechanism of action lies in lowering the synthesis of cholesterol in the liver by competitively inhibiting the enzyme HMG-CoA reductase, the rate-limiting step in cholesterol biosynthesis [Citation7]. Although the lipid-lowering effects of statins are significant, a number of drug interactions with statins remain and may increase the risk of adverse effects, such as myopathy, diabetes mellitus and other possible adverse effects.
Nelumbo nucifera Gaertn., also known as lotus, is a large aquatic herb. Its leaves have been used as food or medicine in Asia, and its medicinal values have been described in Ayurvedic and Traditional Chinese Medicine [Citation8]. N. nucifera leaves are usually dried, known as Folium nelumbinis, and their essential components are alkaloids and flavonoids. These components are closely related to its pharmacological activities, such as anti-obesity, anti-diabetic, anti-inflammatory, and anti-atherosclerotic [Citation8]. It is now well-recognized that atherosclerosis is a systemic cardiovascular disease with complicated pathogenesis, involving in endothelial dysfunction, lipid-accumulation, chronic inflammation and so on. Previous studies have shown that the extracts of N. nucifera leaves could effectively alleviate dyslipidaemia [Citation9, Citation10], prevent neo-intimal hyperplasia by suppressing the migration and proliferation of smooth muscle cells (SMCs) [Citation11, Citation12], and inhibit inflammation [Citation13, Citation14]. Yet, the underlying mechanisms of action of N. nucifera leaves in treating atherosclerosis are still poorly understood. In this study, we employed network pharmacology and molecular docking approaches to explore that. A rough flowchart was designed and illustrated below ().
Materials and methods
Active ingredients of N. nucifera leaves and their potential targets
The keywords of ‘Folium nelumbinis’, ‘Nelumbo nucifera’ or ‘Lotus Leaf’ were imported and searched in the Traditional Chinese Medicine Database and Analysis Platform (TCMSP) (http://tcmsp-e.com) and Encyclopaedia of Traditional Chinese Medicine (ETCM) database (http://www. tcmip.cn/ETCM), and the retrieved results of TCMSP were subsequently screened based on the following parameters: oral bio-availability (OB)≥30% and drug-likeness (DL)≥0.18. Then, the existing results were searched for further analysis involving effective ingredients of N. nucifera leaves for the treatment of atherosclerosis. The 2 D molecular structures of the active ingredients of N. nucifera leaves were obtained and saved as SDF format from the PubChem database (https://pubchem.ncbi.nlm.nih.gov), and then, the potential targets of these ingredients were also predicted by the SwissTargetPrediction platform (http://www.swisstargetprediction.ch). Finally, these active ingredients of N. nucifera leaves and the corresponding targets were imported into Cytoscape 3.7.2 to construct an active ingredient-targets network.
Prediction of atherosclerosis drug targets
The keyword of ‘atherosclerosis’ was used to search for the disease targets in the following databases: Online Mendelian Inheritance in Man (OMIM) (https://www.omim.org), DurgBank (https://www.drugbank.ca), and the GeneCards (https://www.genecards.org). With all the disease-targets obtained and sorted out, they were imported into the Uniprot platform (https://www.uniprot.org) for gene standardization.
Protein–protein interaction (PPI) network construction and analysis
The potential targets of the active ingredients of N. nucifera leaves and the atherosclerosis drug targets were imported into the Venny 2.1.0 software to generate a Venn diagram, and their common targets were obtained, which were mainly involved in the treatment of atherosclerosis. Then, these common targets were imported into the String platform (https://string-db.org) to construct a PPI network, with organism selected for ‘Homo sapiens’. Subsequently, the results were downloaded in ‘.TSV’ format and were inputted into the Cytoscape 3.7.2 software for visualization. The core targets of the PPI network were screened out by the parameters of degree value and closeness centrality value.
Enrichment analysis of GO function and KEGG pathways
The common targets of N. nucifera leaves and atherosclerosis were imported into the Metascape platform (https://metascape.org) for the enrichment analysis of GO function and KEGG pathways. The screening parameter was set as P value less than 0.01. The integrated analyses were also carried out via the Metascape platform, including gene annotation and enrichment analysis [Citation15]. Then, the results were visualized by the Enrichment Bubble Diagram plotted by http://www.bioinfor-matics.com.cn, a bioinformatic on-line platform. All the data mentioned above were based on the common targets of N. nucifera leaves and atherosclerosis.
Construction of active ingredient-target-pathway network
During the process of enrichment analysis for KEGG pathways, the targets enriched in pathways closely related to atherosclerosis were identified. The active ingredients of N. nucifera leaves corresponding to these targets were also confirmed. Thus, the interaction network among the active ingredients, related targets and pathways related to atherosclerosis can be established by using nodes to represent the active ingredients, targets and pathways, separately. The edges represent the association among the active ingredients, targets and the pathways. Then, all the interaction data files were imported into the Cytoscape 3.7.2 software to construct an ‘active ingredient-target-pathway’ network for visualization, according to the methods described in a previous study [Citation16].
Molecular docking simulation
To assess the binding activity between the active ingredients and the core targets, molecular docking was carried out by using AutoDockTools 1.5.6. software. The top 11 core targets with high median degree value in the PPI network were selected as protein receptors, and the active ingredients of N. nucifera leaves as small molecule ligands for molecular docking simulation. The crystal structures of the selected core targets were obtained from RCSB Protein Data Bank (https://www.rcsb.org), a protein crystal database, and saved as PDB format. The 2 D chemical structures of active ingredients were downloaded from the PubChem database (https://pubchem.ncbi.nlm.nih.gov). The 2 D structures of active ingredients were imported into the Chem3D software (CambridgeSoft, USA) to calculate the energy minimization and saved in Mol2 format, while the structures of the core targets were imported into PyMOL to delete the solvent and the organics. After corresponding processing by AutoDockTools 1.5.6, they finally were converted to PDBQT format for molecular docking with PyMOL 2.5.1 [Citation17, Citation18]. The AutoDock Vina software was used to calculate the affinity score of semisoft molecular docking between the active ingredients of N. nucifera leaves and the core targets. A docking score of<-4.25 indicates common binding activity between the ligand and the protein receptor, a score of<-5.0 for better binding activity, and score<-7.0 for the stronger docking activity [Citation19].
Animal experiments
Ethics statement
All animal care and experimental procedures complied with Guidelines for the Care and Use of Laboratory Animals formulated by the Ministry of Science and Technology of China. The Animals Ethics Committee at Guangzhou University of Chinese Medicine approved all animal protocols.
Animals, models and treatments
Male Apoe deficient (Apoe-/-) mice and same genetic background male wide-type (WT) C57BL/6J mice both aged 8 weeks, were purchased from Beijing Vital River Experimental Animal Technical Co., LTD. (http://www.vitalriver.com/) [Certification No. SCXK(Jing)2016-0006], weighing 20 ± 2 g. Apoe-/- male mice were randomly assigned to five groups: Model, Low-dose, Middle-dose (Moderate-dose), High-dose and Simvastatin, and 12 mice per group. Another 12 WT C57BL/6J mice were set as Control. All animals were housed at an ambient temperature of 21 °C under a 12/12 h light-dark schedule (lights off at 7:00). Apoe-/- mice were fed with western high-fat diet (HFD) for 8 weeks to make AS animal models, while C57BL/6J mice with normal chow diet as a control, with ad libitum access to water. The prepared N. nucifera leaves in small pieces were boiled out for concentrated decoction in our lab, corresponding to 60 g herbal decoction for an adult of 60 kg each day, according to the methods in Research Methods in Pharmacology of Chinese Materia Medica (Third edition, 2011). In 8 weeks, Apoe-/- mice were, respectively, given different lavage treatment, low-dose mice with the decoction of N. nucifera leaves (4.51 g/kg), middle-dose (9.01 g/kg), high-dose (18.02 g/kg) and Simvastatin solution (3.00 mg/kg). The model and C57BL/6J mice were both given the same volume of normal saline for control treatment (by weight). All mice were maintained on the same diet for another 8 weeks before sacrifice via cervical dislocation. At the end of the treatments, all mice were fasted overnight, and blood and the aortic vessels were separately collected for the following experiments.
Oil red O staining
The aortic vessels tissue samples were dissected, and stripped cleanly under a stereoscope. After fixation in 4% paraformaldehyde for 24 h, the aortic vessels were fully washed with water. Then, after immersed cleaning with 60% isopropyl alcohol, the vessels were adequately stained with 60% working solution of Oil Red O (Solarbio, Beijing) for 30 min. Next, the vessels were differentiated by 60% isopropyl alcohol to clear stroma. Lastly, the aortic vessels were washed clear again and were dried with blotting paper to take photographs under an inverted fluorescence microscope (Olympus, Japan).
Serum lipid examination
After the blood samples of mice were collected with vacuum blood tube, they were left at room temperature for over 2 h (for blood clots), centrifuged at 3000 rpm (Eppendorf, 5810 R), 4 °C for 15 min. Then, the serums of blood samples were obtained by collecting the supernatant carefully and were stored in a new EP tube. The levels of blood lipids of mice in each group were examined with an automatic biochemical analyser (Rayto, Shenzhen), with total cholesterol (TCHO), triglyceride (TG), low density lipoprotein (LDL-C) and high density lipoprotein (HDL) testing kit (Huili, Changchun), respectively.
Levels of inflammatory factors
As mentioned previously, we obtained the serum samples of mice in each group. We then used enzyme-linked immunosorbent assay (ELISA) to measure the inflammatory factors levels. As a matter of fact, the operating process of ELISA experiments was strictly carried out to ensure the accuracy of the experimental results, according to the reagent instructions. These inflammatory markers were examined to assess the effects of N. nucifera leaves on atherosclerotic inflammation, including TNF-α, IL-1β and IL-6 (Elabscience, Wuhan).
Results
Active ingredients of N. nucifera leaves and their potential targets
We first screened the active ingredients of N. nucifera leaves in the TCMSP database and ETCM database, based on the parameters of OB value and DL value. A total of 16 active ingredients of N. nucifera leaves were obtained from the databases (). Then, these active ingredients were inputted into PubChem to acquire the 2 D structure and saved in ‘.sdf’ format. These results were imported into the SwissTargetPrediction platform to predict the potential targets, and a total of 219 potential targets were obtained. The Cytoscape 3.7.2 software was employed to construct an active ingredient-target network for visualization, and the major active ingredients of N. nucifera leaves were: quercetin, kaempferol, O-nornuciferine, nuciferine, sitosterol, pronuciferine, (R)-N- -methylcoclaurine, anonaine and nelumboside ().
Figure 2. An active ingredient-targets network of N. nucifera leaves (Heye). The diamond nodes stand for the active ingredients of N. nucifera leaves, and the circular nodes for their potential targets. The triangle node stands for the N. nucifera leaf. The connecting lines represent the relationship between the ingredients and the targets.
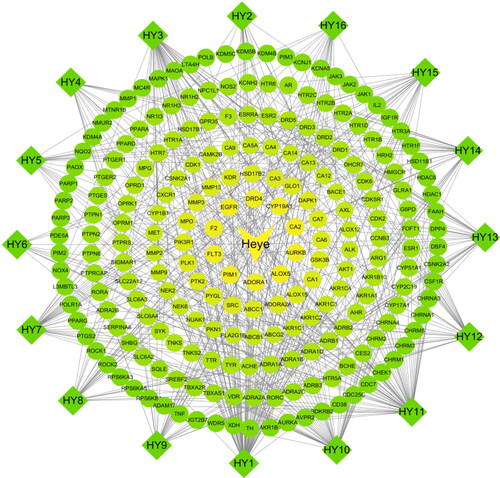
Table 1. Active ingredients of Nelumbo nucifera.
Potential atherosclerosis drug targets
The potential targets related to atherosclerosis were searched and retrieved in the following three disease databases: OMIM, DrugBank and GeneCards. As a result, a total of 1211 disease targets were obtained, after removing the duplicate targets. Notably, there were 144 targets from OMIM, 38 targets from DrugBank and 1145 targets from GeneCards. Then, all the disease targets and the potential targets of N. nucifera leaves were used to acquire the intersection targets, which were considered as the major targets for the treatment of atherosclerosis.
PPI (protein–protein interaction) network construction and analysis
The targets of N. nucifera leaves and the targets of atherosclerosis were separately inputted into Venny 2.1.0 software to generate a Venn diagram (), and a total of 75 overlapping targets were obtained. Then, these common targets were imported into the STRING platform(Version 11.0), with organism chosen for ‘Homo sapiens’, and a PPI network was constructed. The results showed that there were 75 nodes with 511 edges in the network, with an average node degree of 13.6 and average clustering coefficient of 0.567. Subsequently, all the results were downloaded and imported into the Cytoscape 3.7.2 software to construct a PPI network for visualization (), and we evaluated the targets with parameters of degree value and closeness centrality value. The top 11 targets were identified and considered as the core targets of the PPI network, indicating that they may play a key role in treating atherosclerosis, including AKT1, TNF, PTGS2, SRC, EGFR, MAPK1, MMP9, KDR, ESR1, PPARG and MMP2 ().
Figure 3. Venn diagram for the targets of active ingredients of N. nucifera leaf and atherosclerosis drugs.
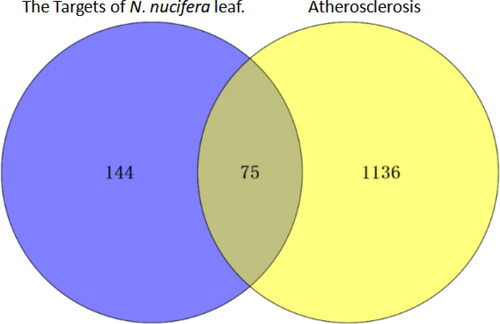
Figure 4. PPI network of the common targets of N. nucifera leaf and atherosclerosis. The octagons represent the common targets, and the lines stand for the interaction of targets.
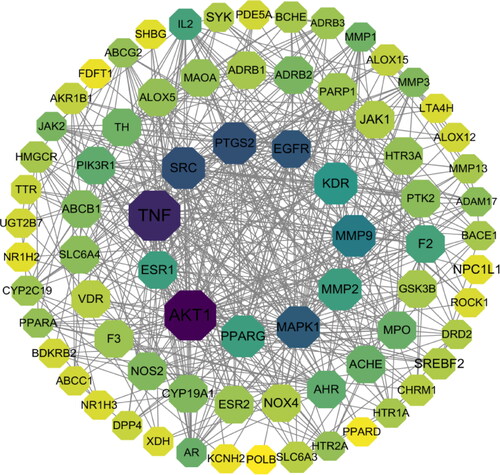
Table 2. Core targets of N. nucifera leaves in treating atherosclerosis.
Enrichment analysis of GO function and KEGG pathways of the common targets
After all the common targets were imported into the Metascape platform, the GO functions were enriched as follows (p<0.01, ), including the biological processes: circulatory system process, cellular response to organic cyclic compound, inflammatory response, lipid biosynthetic process, positive regulation of lipid metabolic process, phosphatidylinositol 3-kinase signalling, and cellular response to oxidative stress; Molecular functions: nuclear receptor activity, ligand-activated transcription factor activity, phosphatase binding, protein tyrosine kinase activity, lipid binding, G protein-coupled amine receptor activity and ABC-type xenobiotic transporter activity. In addition, the KEGG pathways were also enriched by these common targets (p<0.01, ). Among them, the pathways mainly related to atherosclerosis were these: PI3K-Akt, VEGF, TNF, PPAR signalling pathway, insulin resistance, and regulation of lipolysis in adipocytes, indicating that N. nucifera leaves may function in treating atherosclerosis by regulating these KEGG pathways.
Construction of the active ingredient-target-pathway network
In order to intuitively show the interactions and the underlying mechanisms of N. nucifera leaves in treating atherosclerosis, an ‘active ingredient-target-pathway’ network was constructed with the most relevant pathways related to atherosclerosis, the corresponding targets and the active ingredients of N. nucifera leaves (). The results showed that there were 48 nodes with 133 edges in the network, including 16 active ingredients of N. nucifera leaves, 26 targets and 6 signalling pathways. In this network, the active ingredients with more targets were these: quercetin, isorhamnetin, kaempferol, nuciferine, sitosterol, (R)-N-Methylcoclaurine, N-Nornuciferine and pronuciferine, indicating that they were the major molecular compound basis in treating atherosclerosis. In addition, AKT1, PIK3R1, MAPK1, PTGS2, TNF and MMP9 were the targets that connected with more ingredients and pathways, suggesting that these targets may be core targets for the treatment of atherosclerosis. More importantly, these results were mostly consistent with the PPI network analysis. Therefore, these active ingredients of N. nucifera leaves may function in treating atherosclerosis by regulating these signalling pathways through the corresponding targets.
Molecular docking between the active ingredients and the core targets
Based on the results of KEGG pathways and the PPI network analysis, we selected the top 11 core targets of the degree value and the closeness centrality value for further research (). These targets were molecularly docked with the active ingredients of N. nucifera leaves, including quercetin, isorhamnetin, kaempferol, sitosterol, nuciferine, pronuciferine, nelumboside, separately, according to the drug-target correspondence. The most stable conformation is the one with the lowest binding energy. Among them, we found that the Kaempfero-MMP9 complex had the lowest affinity value of −10.3 kcal/mol, indicating that they bind best with each other. shows the details of the molecular docking score, with a range of −10.3 to −5.5 kcal/mol.
Table 3. Results of molecular docking simulation.
Additionally, as shown in , some of the results of molecular docking were selected for visualization by using PyMOL, exhibiting the binding activity between the active ingredients and the core targets.
Figure 8. Results of molecular docking between the active ingredients and the core targets of N. nucifera leaves: quercetin to AKT1 (a); quercetin to SRC (b); quercetin to EGFR (c); quercetin to MMP9 (d); quercetin to VEGFR-2 (e); sitosterol to PPARG (f); pronuciferine to MMP9 (g); nuciferine to PTGS2 (h); nelumboside to TNF (i); nelumboside to PTGS2 (j); kaempferol to MMP9
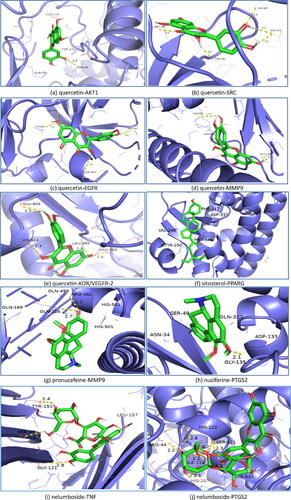
Oil red O staining
To assay the efficacy of N. nucifera leaves experimentally, we performed Oil Red O staining of the aortic vessels of mice treated by different interventions. The results showed that there were significant atherosclerotic plaques on the aortic vessels of mice in the model group, compared with the mice in the control group. The atherosclerotic lesions of aortic vessels of mice in the low, middle and high-dose groups were improved to various degrees, after the intragastrical administration of 8 weeks with different dosages of decoction. However, there were no remarkable differences in the atherosclerotic plaques observed between the middle-dose and high-dose group. Additionally, as a positive control, the mice in the simvastatin group had the fewest atherosclerotic plaques ().
Figure 9. Oil Red O staining of aortic vessels of male Apoe deficient (Apoe-/-) mice in different groups and wild-type (control) C57BL/6J mice. Treatment was initiated at 17 weeks of age and continued for another 8 weeks with intragastrical administration. Low-dose, Middle (Moderate)-dose and High-dose groups were, respectively, given a dose of 4.51 g/kg/d, 9.01 g/kg/d and 18.02 g/kg/d of herbal decoction of N. nucifera leaves, while the control and model group were given 0.9% normal saline. The Simvastatin group was given 3.00 mg/kg/d of Simvastatin solution as a positive control. The red areas represent the atherosclerotic plaques of aortic vessels of mice in different groups.
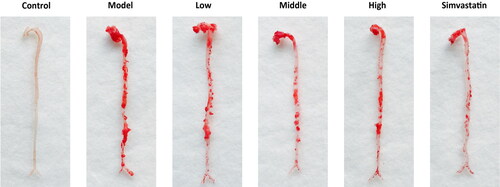
The levels of serum lipids
Lipids, the risk factors of vascular diseases, are also considered as an important driving force in atherogenesis. In this study, we examined the lipids levels of mice in different groups. The results showed that the levels of TG, TCHO and LDL of mice in the model group were significantly higher than those of mice in the control group. The levels of TG, TCHO and LDL in the low, middle and high-dose groups were reduced, compared with those in the model group. The best effects were in the mice in the high-dose group. On the contrary, the levels of HDL in the low, middle and high group were more or less enhanced. Simvastatin had the best effects in terms of lowering the lipids, as a positive control ().
Figure 10. N. nucifera leaves improve the levels of serum lipids in an AS mouse model. A to D, Serum TG (A), TCHO (B), HDL (C) and LDL (D) levels. The levels of serum TG, TCHO, HDL and LDL of mice in each group were examined with an automatic biochemical analyzer, with TG, TCHO, HDL and LDL testing kits, respectively.
Note: Values are means ± SEM (n = 6–8; each data point represents 1 mouse). ***P<0.001 (Control vs. Model) or (Model vs. Low, Middle, High, Simvastatin group), **P<0.01 for LDL (Model vs. Low, Middle, High, Simvastatin group), ns. P > 0.05 for TG (Middle vs. High) or HDL (Low vs. Middle) or LDL (Low vs. Middle) by one-way ANOVA followed by Bonferroni or Tamhane’s T2 post-test.
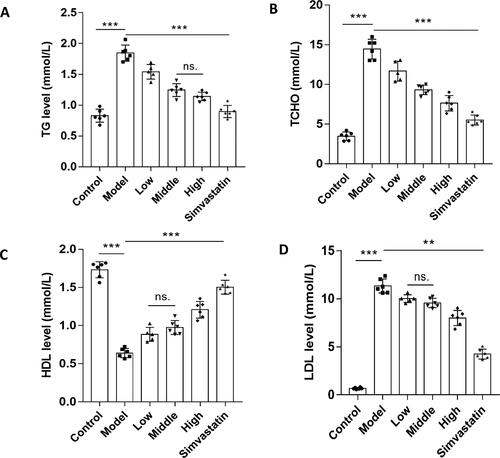
Inflammatory factors levels
Atherosclerosis is a lipid-driven chronic and inflammatory disease of the arterial intima, in which inflammation always exists, playing an important role in atherogenesis. To test the anti-inflammatory efficacy of N. nucifera leaves, we examined the levels of inflammatory factors of mice in different groups with an ELISA kit. The results showed that the levels of TNF-α, IL-1β and IL-6 of mice in the model group were higher than those of mice in the control group. In agreement with the lipid levels, the N. nucifera leaf improved the expressions of inflammatory factors in various degrees (). In addition, the anti-inflammatory effects of simvastatin were also significant in the positive control group. Based on above, we considered that N. nucifera leaves had positive effects of anti-inflammation, which were possibly achieved by inhibiting the inflammatory pathways.
Figure 11. N. nucifera leaves lowered the levels of inflammatory factors in an AS mouse model. Serum TNF-α (A), IL-1β (B) and IL-6 (C) levels measured by ELISA (n = 6–8; each data point represents 1 mouse).
Note: Values were shown as means ± SEM, ***P<0.001 (Control vs. Model) or (Model vs. Low-dose, Mid-dose, High-dose, Simvastatin), **P<0.01 for IL-1β (Mid-dose vs. High-dose), ns.P > 0.05 for TNF-α (Mid-dose vs. High-dose) by one-way ANOVA followed by Bonferroni or Tamhane’s T2 multiple comparisons test.
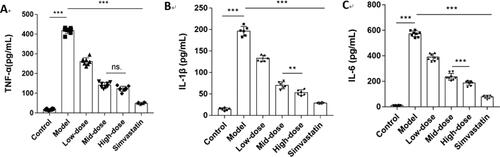
Discussion
Nelumbo nucifera Gaertn., a plant of Nymphaeaceae family, is widely distributed in Asian countries and now has been recognized as an effective medicinal plant whose extract contains a variety of bioactive ingredients, such as alkaloids, flavonoids (with their glycosides or terpenoids), steroids, unsaturated fatty acids etc. [Citation8, Citation20]. Modern pharmacological studies have shown that N. nucifera leaves have various therapeutic efficacy, including anti-hyperlipidaemia, anti-diabetic, insulin secretagogue, anti-obesity, anti-oxidant, anti-inflammatory, anti-atherosclerotic, anti-cancer and hepato-protective activity [Citation13, Citation14, Citation21–26]. Yet, it is poorly understood how its active ingredients function in the treatment of atherosclerosis. In this study, we employed network pharmacology and molecular docking approaches to explore that, and initially verified it by animal experiments.
As a result, a total of 16 active ingredients and 219 potential targets of N. nucifera leaf were identified from databases. The top 11 core targets of the PPI network were also obtained, which was constructed by the common targets of substances found in N. nucifera leaves and atherosclerosis drugs. Then, we performed enrichment analysis of GO functions and KEGG pathways with these common targets. The 6 most relevant signalling pathways related to atherosclerosis were PI3K/Akt, TNF, VEGF, PPAR signalling pathway, regulation of lipolysis in adipocytes and insulin resistance. Then, an ‘active ingredient-target-pathway’ network was constructed to further explore the underlying mechanisms of N. nucifera leaves in treating atherosclerosis. AKT1, PIK3R1, MAPK1 (ERK2), PTGS2 (COX2), TNF and MMP9 were the key targets, which had more connections with the active ingredients and pathways in the network. Moreover, we found that these results were closely consistent with the ones from the PPI network analysis, indicating that these active ingredients of N. nucifera leaves may function in treating atherosclerosis through these targets by regulating the six most relevant signalling pathways. Subsequently, we performed molecular docking between the active ingredients and their core targets to assess their binding activity, and the results showed they have stronger binding ability. Thus, we considered that these active ingredients and their core targets may play an important role in treating atherosclerosis. The underlying mechanisms of N. nucifera leaves in treating atherosclerosis may be closely associated with these KEGG pathways.
According to the parameters of degree value and closeness centrality value, the top 11 core targets of the PPI network were obtained, including AKT1, TNF, PTGS2(COX2), SRC, EGFR, MAPK1, MMP9, KDR, ESR1, PPARG and MMP2. Generally, these targets can be classified into three categories, namely targets associated with lipid metabolism, inflammation and atherosclerotic plaques. Simultaneously, these targets are closely related to the pathways referring to atherosclerosis. Guo et al. [Citation10] reported that nuciferine, a major active aporphine alkaloid from N. nucifera leaves, could improve the HFD-induced dyslipidaemia and regulate lipid metabolism by reducing the expression of lipid-related genes and/or proteins, such as PPAR-γ, LXR-α, SREBP-1c. Furthermore, nuciferine may ameliorate insulin resistance and metabolic syndrome by normalizing HFD-induce adipokine secretion. Its anti-inflammatory and anti-oxidative effects also acted an important role in preventing hepatic steatosis and injury. In another study, the extracts from N. nucifera leaves could modulate the lipid metabolism by reducing the adipocyte differentiation and fat accumulation via lipids-related genes, including PPAR-γ, C/EBP-α, PPAR-α, CPT1, CYP7A1, and alleviate inflammation by inhibiting the release of inflammatory cytokines, such as TNF-α, IL-1β, IL-6 and IFN-γ [Citation27].
Previous studies also showed that kaempferol isolated from N. nucifera leaves could effectively reduce the lipid accumulation in adipocytes presumably by inhibiting the expression of a wide range of adipogenic transcription factors and by increasing PPARα-mediated fatty acid oxidation signals [Citation21]. Zhang et al. [Citation28] found that nuciferine could improve the lipid profiles and attenuate hepatic steatosis by activating PPARα/PGC1α pathway and regulating hepatic lipid metabolism, and it could also restore the impaired glucose tolerance and insulin resistance. In another study, the researchers reported that nuciferine and pronuciferine isolated from N. nucifera leaves could decrease the lipid accumulation by enhancing the expression of GLUT4, and ameliorate insulin resistance via activating the AMPK signalling pathway [Citation23]. Furthermore, the flavonoids from N. nuciferine leaves, including quercetin, kaempferol, isorhamnetin, catechin, and isoquercitrin, exhibited lipolytic activity in visceral adipose tissue [Citation8], and this also plays a crucial role in cardiovascular diseases [Citation29]. Therefore, we speculate that the functions of N. nucifera leaves could be closely connected with the PPAR signalling pathway, insulin resistance, and regulation of lipolysis in adipocytes.
Atherosclerosis is considered as a chronic inflammatory disease, and inflammation acts an important role in atherogenesis. Accumulating previous studies have revealed the anti -inflammatory effects of N. nucifera leaves. Zhou et al. [Citation24] reported that alkaloids of N. nucifera leaves, such as nuciferine, nuciferine, isoliensinine, and other compounds might generally possess anti-inflammatory effects, which could block the NF-kB activity. Liu et al. [Citation30] found that N. nucifera leaves and its components, such as quercetin and catechin, could prevent the inflammatory responses in macrophages through JNK/NF-kB signalling pathway. And the results showed that both of them could significantly decrease the protein expression of iNOS, COX-2 and phospho-JNK, and reduce the level of IL-6 and TNF-α. Researchers also found that Nelumbo leaf extracts could significantly decrease the expression of pro-inflammatory mediators (such as IL-1β, IL-6, TNF-α) by inhibiting the NF-kB-mediated inflammatory responses [Citation31]. Besides, Zhang et al. [Citation32] found that nuciferine could inhibit pro-inflammatory cytokines via activating PPARα and PPARγin Raw 264.7 cells. Thus, the anti-inflammatory effects of the compounds in N. nucifera leaves and may act an important role in the treatment of atherosclerosis.
Other studies have shown that the vascular smooth muscle cells (VSMCs) took a part in the formation of atherosclerotic plaques [Citation33–36]. The migration of VSMCs from the media to the intima and following proliferation are considered the primary events in the development of intimal hyperplasia in atherogenesis. Targeting the migration and proliferation of SMCs may provide a therapeutic strategy in the prevention and treatment of atherosclerosis [Citation1]. Ho et al. [Citation11] reported that the extracts from nucifera leaves could reduce the development of atherosclerosis through inhibition of VSMCs proliferation and migration. Another study reported that N. nucifera leaf extracts could efficiently reduce intima thickening by suppressing the proliferation of VSMCs, and prevent migration by reducing the expression of MMP2 and MMP9 [12]. Lee et al. [Citation37] found that N. nucifera leaves extracts could also significantly inhibit the VEGF-induced angiogenesis, proliferation and tube formation in HUVECs, confirming its possible anti-angiogenic mechanism. Additionally, the flavonoid compounds of N. nucifera leaves, such as quercetin and isorhamnetin, were reported to inhibit the development of atherosclerotic plaques by regulating the PI3K/Akt signalling pathway [Citation38, Citation39]. Kaempferol could alleviate ox-LDL-induced apoptosis of endothelial cells by inhibiting the PI3K/Akt/mTOR pathway [Citation40]. Thus, the anti-atherosclerotic effects of N. nucifera leaves are relevant to VEGF and PI3K/Akt pathways.
In terms of the biological processes, we found that many important processes were probably involved, including the cellular response to lipids, inflammatory responses, lipid biosynthetic processes, positive regulation of lipid metabolic processes and cellular response to oxidative stress, which are closely related to atherosclerosis. As known, lipid accumulation, chronic inflammation and oxidative stress, are considered the most important aspects of atherosclerosis [Citation1, Citation41–43]. Thus, we considered that these biological processes are involved in the treatment of atherosclerosis by N. nucifera leaves. The molecular docking between the active ingredients of N. nucifera leaves and the core targets also showed their stronger binding activity, indicating that N. nucifera leaves exerted anti-atherosclerotic effects possibly via these active ingredients and the key targets by regulating the KEGG pathways and biological processes.
To preliminarily validate our network pharmacology and molecular docking results, we performed animal experiments and found that N. nucifera leaves could effectively reduce the atherosclerotic plaques and lower the levels of lipids and inflammatory factors. This indicated that N. nucifera leaves could improve atherosclerosis possibly by affecting the lipid metabolism and by exerting anti-inflammatory effects. Yet, further research is needed to elucidate the molecular mechanisms underlying the action of N. nucifera leaves in treating atherosclerosis.
Conclusions
We identified a group of active ingredients of N. nucifera leaves, with potential clinical application in the present study. These active ingredients could improve lipid metabolism possibly by regulating the PPAR signalling pathway, insulin resistance and lipolysis in adipocytes. They could also exert anti-inflammatory effects by decreasing the expression of TNF-α, IL-1β, IL-6, iNOS and COX2, and inhibiting the NF-kB-mediated inflammatory response. They may reduce the intimal hyperplasia via suppressing the migration and proliferation of SMCs. Thus, we speculated that N. nucifera leaves may function in treating atherosclerosis possibly by improving dyslipidaemia, inhibiting the inflammation and intimal hyperplasia, to attenuate the development of atherosclerosis. The molecular docking between the active ingredients of N. nucifera leaves and their core targets showed they had stronger binding activity. The animal experiments also preliminarily validated the results of network pharmacology and molecular docking. Accordingly, our present study provides experimental reference for the underlying mechanisms and clinical application of N. nucifera leaves in treating atherosclerosis and its complications. Still, further studies need to be performed to verify these observations.
Authors’ contributions
Linhai Wang and Zhuoji Guan are the first authors. Lingjun Wang and Shaoxiang Xian designed the study and re-examined the manuscript. Linhai Wang and Shaodong Li finished all the data collection, analysis and interpretation. Zhuoji Guan, Xin Dong, Jialin Jiang made all tables, graphs and performed the molecular docking. Linhai Wang, Zhuoji Guan finished the animal experiments. Linhai Wang wrote the manuscript.
Acknowledgments
The authors thank the Lingnan Medical Research Center of Guangzhou University of Chinese Medicine.
Data availability
The data used to support the findings of this study are available from the corresponding authors upon reasonable request.
Disclosure statement
All the authors declare that they have no conflicts of interest or personal relationships that have influenced the work reported in this article.
Additional information
Funding
References
- Libby P, Buring J, Badimon L, et al. Atherosclerosis. Nat Rev Dis Primers. 2019;5(1):56.
- Back M, Yurdagul AJ, Tabas I, et al. Inflammation and its resolution in atherosclerosis: mediators and therapeutic opportunities. Nat Rev Cardiol. 2019;16(7):389–406.
- Ference BA, Ginsberg HN, Graham I, et al. Low-density lipoproteins cause atheroslcerotic cardiovascular disease. 1. Evidence from genetic, epidemiologic, and clinical studies. A consensus statement from the European atherosclerosis society consensus panel. Eur Heart J. 2017;38(32):2459–2472.
- Ridker PM, Everett BM, Thuren T, et al. Antiinflammatory therapy with canakinumab for atherosclerotic disease. N Engl J Med. 2017;377(12):1119–1131.
- World Health Organization. Cardiovascular diseases (CVDs) fact sheet. 2017.
- GBD 2015 Disease and Injury Incidence and Prevalence Collaborators. Global, regional, and national incidence, prevalence, and years lived with disability for 310 diseases and injuries, 1995–2015: a systematic analysis for the global burden of disease study 2015. Lancet. 2016;388(10053):1545–1602.
- European Soc. Cardiology; European Atherosclerosis Soc. 2019 ESC/EAS guideline for the management of dyslipidaemias: lipid modification to reduce cardiovascular risk. Eur Heart J. 2020;41(1):111–188.
- Sharma BR, Gautam LN, Adhikari D, et al. A comprehensive review on chemical profiling of Nelumbo nucifera: potential for drug development. Phytother Res. 2017;31(1):3–26.
- Liu S, Li D, Huang B, et al. Inhibition of pancreatic lipase, alpha-glucosidase, alpha-amylase, and hypolipidemic effects of the total flavonoids from Nelumbo nucifera leaves. J Ethnopharmacol. 2013;149(1):263–269.
- Guo FC, Yang X, Li XX, et al. Nucifera prevents hepatic steatosis and injury induced by a high-fat diet in hamsters. PLoS One. 2013;8(5):E63770.
- Ho HH, Hsu LS, Kuei KC, et al. Extract from the leaf of nucifera reduced the development of atherosclerosis via inhibition of vascular smooth muscle cell proliferation and migration. Food Chem Toxicol. 2010;48(1):159–168.
- Rajendra KK, Eun-Raye J, Dong-Wook K. Nelumbo nucifera leaf extract inhibits neointimal hyperplasia through modulation of smooth muscle cell proliferation and migration. Nutrition. 2013;29(1):268–275.
- Lin HY, Kuo YH, Lin YL, et al. Antioxidative effect and active components from leaves of lotus (Nelumbo nucifera). J Agric Food Chem. 2009;57(15):6623–6629.
- Wang MX, Liu YL, Yang Y, et al. Nuciferine restores potassium oxonate-induced hyperuricemia and kidney inflammation in mice. Eur J Pharmacol. 2015;747:59–70.
- Zhou YY, Zhou B, Pache L, et al. Metascape provides a biologist-oriented resource for the analysis of systems-level datasets. Nat Commun. 2019;10(1):1523.
- Franz M, Lopes CT, Huck G, et al. Cytoscape.js: a graph theory library for visualisation and analysis. Bioinformatics. (Oxford, England). 2016;32(2):309–311.
- Trott O, Olson AJ. AutoDock vina: improving the speed and accuracy of docking with a new scoring function, efficient optimization, and multithreading. J Comput Chem. 2010;31(2):455.
- Zhu N, Hou J. Exploring the mechanism of action Xianlinggubao prescription in the treatment of osteoporosis by network pharmacology. Comput Biolo Chem. 2020;85:107240.
- Hsin K-Y, Ghosh S, Kitano H. Combining machine learning systems and multiple docking simulation package to improve docking prediction reliability for network pharmacology. PLoS One. 2013;8(12):e83922–129.
- Chang C-H, Ou T-T, Yang M-Y, et al. Nelumbo nucifera Gaertn leaves extract inhibits the angiogenesis and metastasis of breast cancer cells by downregulation connective tissue growth factor (CTGF) mediated PI3K/AKT/ERK signaling. J Ethnopharmacol. 2016;188:111–122.
- Lee B, Kwon M, Jae JS, et al. Kaempferol isolated from Nelumbo nucifera inhibits lipid accumulation and increases fatty acid oxidation signaling in adipocytes. J Med Food. 2015;18(12):1363–1370.
- Nguyen KH, Ta TN, Pham TM, et al. Nuciferine stimulates insulin secretion from beta cells -An in vitro comparison with glibenclamide. J Ethnopharmacol. 2012;142(2):488–495.
- Ma CJ, Li G, He YF, et al. Pronuciferine and nuciferine inhibit lipogenesis in 3T3-L1 adipocytes by activating the AMPK signaling pathway. Life Sci. 2015;136:120–125.
- Zhou MG, Jiang M, Ying XH, et al. Identification and comparison of anti-inflammatory ingredients from different organs of lotus Nelumbo by UPLC/Q-TOF and PCA coupled with a NF-kappa B receptor gene assay. PLoS One. 2013;8(11):E81971.
- Jun MY, Karki R, Paudel KR, et al. Alkaloid rich fraction from Nelumbo nucifera targets VSMC proliferation and migration to suppress restenosis in balloon-injured rat carotid artery. Atherosclerosis. 2016;248:179–189.
- Xu LM, Zhang XY, Li YN, et al. Neferine induces autophagy of human ovarian cancer cells via p38 MAPK/JNK activation. Tumour Biol. 2016;37(7):8721–8729.
- Wu Y, Tan F, Zhang TY, et al. The anti-obesity effect of lotus leaves on high-fat-diet-induced obesity by modulating lipid metabolism in C57BL/6J mice. Appl Biol Chem. 2020;63(1)
- Zhang C, Deng JJ, Liu D, et al. Nuciferine ameliorates hepatic steatosis in high-fat diet/streptozocin-induced diabetic mice through a PPAR alpha/PPAR gamma coactivator-1 alpha pathway. Br J Pharmacol. 2018;175(22):4218–4228.
- Vazhappilly CG, Ansari SA, Al-Jaleeli R, et al. Role of flavonoids in thrombotic, cardiovascular, and inflammatory disease. Inflammopharmacology. 2019;27(5):863–869.
- Liu SH, Lu TH, Su CC, et al. Lotus leaf (Nelumbo nucifera) and its active constituents prevent inflammatory responses in macrophages via JNK/NF-kB signaling pathway. Am J Chin Med. 2014;42(4):869–889.
- Park E, Kim GD, Go M-S, et al. Anti-inflammatory effects of Nelumbo leaf extracts and identification of their metabolites. Nutr Res Pract. 2017;11(4):265–274.
- Zhang C, Deng JJ, Liu D, et al. Nuciferine inhibits proinflammatory cytokines via the PPARs in LPS-induced RAW 264.7 cells. Molecules. 2018;23(10):2723.
- Osonoi Y, Mita T, Azuma K, et al. Defective autophagy in vascular smooth muscle cells enhances cell death and atherosclerosis. Autophagy. 2018;14(11):1991–2006.
- Wirka RC, Wagh D, Paik DT, et al. Atheroprotective roles of smooth muscle cell phenotypic modulation and the TCF21 disease gene as revealed by single-cell analysis. Nat Med. 2019;25(8):1280–1289.
- Zhu JM, Liu B, Wang ZY, et al. Exosomes from nicotine-stimulated macrophages accelerate atherosclerosis through miR-21-3p/PTEN-mediated VSMS migration and proliferation. Theranostics. 2019;9(23):6901–6919.
- Pan H, Xue C, Auerbach BJ, et al. Single-cell genomics reveals a novel cell state during smooth muscle cell phenotypic switching and potential therapeutic targets for atherosclerosis in mouse and human. Circulation. 2020;142(21):2060–2075.
- Lee JS, Shukla S, Kim J-A, et al. Anti-angiogenic effect of Nelumbo nucifera leaf extracts in human umbilical vein endothelial cells with antioxidant potential. PLoS One. 2015;10(2):E0118552.
- Lu XL, Zhao CH, Xin XL, et al. Quercetin attenuates high fructose feeding-induced atherosclerosis by suppressing inflammation and apoptosis via ROS-regulated PI3K/AKT signaling pathway. Biomed Pharmacother. 2017;85:658–671.
- Luo Y, Sun GB, Dong X, et al. Isorhamnetin attenuates atherosclerosis by inhibiting macrophage apoptosis via PI3K/AKT activation and HO-1 induction. PLoS One. 2015;10(3):e0120259–259.
- Che JB, Liang B, Zhang Y, et al. Kaempferol alleviates ox-LDL-induced apoptosis by up-regulation of autophagy via inhibiting PI3K/akt/mTOR pathway in human endothelial cells. Cardiovasc Pathol. 2017;31:57–62.
- Steven S, Frenis K, Oelze M, et al. Vascular inflammation and oxidative stress: major trigger for cardiovascular disease. Oxid Med Cell Longev. 2019;2019:7092151.
- Marchio P, Guerra-Ojeda S, Vila JM, et al. Targeting early atherosclerosis: a focus on oxidative stress and inflammation. Oxid Med Cell Longev. 2019;2019:8563845.
- Emilie D, Victoriane P, Annie T, et al. Oxidative stress in cardiovascular disease. Antioxidants. 2020;9(9):864.