Abstract
Tomato (Solanum lycopersicum) is one of the highest-value vegetable crops worldwide, but tomato planting can be easily affected by leaf mold. Tomato leaf mold disease is caused by Cladosporium fulvum, and plants carrying the Cf genes have effective resistance to C. fulvum. To identify leaf mold resistant varieties and screen for specific cDNA amplified fragment length polymorphism (cDNA-AFLP) molecular markers, tomato plants harbouring genes Cf-5, Cf-11, Cf-19, and the moneymaker (MM) tomato line (without Cf gene) were used as plant materials. The changes in reactive oxygen species (ROS) levels and antioxidant activities of plant leaves after inoculation of C. fulvum were measured. Ethylene (ETH), abscisic acid (ABA) and salicylic acid (SA) contents were also determined. The results revealed that the ROS levels in Cf-19 tomato were the most sensitive to C. fulvum inoculation, while in MM they were the least. The antioxidant enzymes of Cf-carrying plants exhibited different activities as compared to those of MM. In the cDNA-AFLP analysis data, the expression of ERF109, sedoheptulose-1,7-bisphosphatase (SBPase), L-ascorbic acid oxidase (AAO) and Thioredoxin H2 (TRX2) were different between Cf-gene carriers and MM plants. In summary, in the process of leaf–mold interaction, the outbreak of ROS and development of systemic acquired resistance (SAR) are tightly combined with antioxidant enzymes, including thioredoxin. Tomato plants carrying the Cf-19 gene appear to have stronger C. fulvum resistance.
Introduction
Leaf mold, a common disease of tomato plants grown in enclosed structures, is caused by Cladosporium fulvum Cooke. So far, leaf molds detected in outdoor-grown tomatoes have evolved into a major disease in China [Citation1]. Cf genes encode receptor-like proteins (RLPs), type I transmembrane glycoproteins containing extracellular leucine-rich repeats (eLRRs), a transmembrane region and a short cytoplasmic domain with no similarity to known signalling = domains [Citation2]. During tomato infection, the fungus express avirulence effector proteins, i.e. Avr2, Avr4, Avr4E, Avr5 and Avr9, to suppress host immunity or protect itself [Citation3]. Notably, each of those effectors is recognized by the product of a corresponding dominant resistance gene (Cf-2, Cf-4, Cf-4E, Cf-5 and Cf-9) through gene-for-gene interaction [Citation4]. Employment of these Cf genes effectively controls the disease in most cultivation areas. This, however, has accelerated the differentiation of physiological races. Studies have identified more than 20 Cf resistance genes in tomato germplasm resistant to various physiological races of C. fulvum [Citation5]. In parallel, there are already some physiological races of C. fulvum that can overcome many of these Cf genes, including Cf-2, Cf-4 and Cf-6 [Citation6]. That is why it is important to identify novel Cf genes and develop a more efficient approach to control C. fulvum, including the utilization of key genes in the resistance response pathway.
cDNA amplified fragment length polymorphism (cDNA-AFLP) is one of the transcriptome analyses methods applied for various organisms, specifically those with insufficient sequence information in existing databases. Notably, cDNA-AFLP is a readily available, low-cost and robust technology for plants [Citation7]. As an alternative to next-generation sequencing (NGS), the cDNA-AFLP technique is an extensively adopted gene expression profiling method. cDNA-AFLP has been used in different plants for its reliability and repeatability [Citation8–10]. With the benefit of no requirement for prerequisite reference genome sequence, cDNA-AFLP has been used to unravel regulation mechanisms under various biotic and abiotic stress conditions affecting patterns of global gene expression in diverse crop species [Citation11–13].
Herein, we used tomato plants carrying Cf-5, Cf-11, Cf-19 genes and the Moneymaker tomato line (without a Cf gene) as plant materials. The specific genes were screened by analyzing the expression changes via cDNA-AFLP when plants were infected by C. fulvum. Our findings will provide data support to identify leaf mold resistant varieties and screen for specific cDNA-AFLP molecular markers.
Materials and methods
Tomato planting and C. fulvum inoculation
Four tomato lines: Ontrio7516 (carrying the Cf-5 gene), HN42 (carrying the Cf-11 gene), HN19 (carrying the Cf-19 gene) and Moneymaker (not carrying a Cf gene), were used as plant materials to analyze the physiological responses of C. fulvum. Moneymaker was the line susceptible to C. fulvum, while the other three lines were resistant. All these plant seeds were provided and identified by the Germplasm resources centre of the Chinese Academy of Agricultural Sciences. Seeds were directly sown into mixed peat with vermiculite (2:1) medium and placed in a constant temperature incubator at 25 °C with > 90% ambient humidity and 16 h light daily. In total, 60 tomato plants (15 plants per variety, one plant one pot) were used. For C. fulvum inoculation, the abaxial leaf surfaces were sprayed with prepared C. fulvum suspension (Race 1.2.3.4, 10 mL, 1 × 107 sporangia/mL) at the 4–6 leaves stage (4-week-old).
The physiological race 1.2.3.4 was obtained from the tomato research group of the Horticulture College, Northeast Agriculture University, China. Leaf samples (two leaves per plant) were harvested on the 0 (control group, sampling before spraying), 5th, 10th, 15th and 20th day post-infection (dpi) to measure the enzymatic activity, hormone levels and microscopic observation with three replications. Leaf samples (two leaves per plant) collected at 0, 7th and 14th day were used for trypan blue staining. After 20 days of inoculation, leaf samples were collected for cDNA-AFLP sequencing. The samples inoculated for 0 days were used as the control group (CK).
Trypan blue staining
Trypan blue staining of tomato leaves (0th, 7th, and 14th day after inoculation) was performed by soaking seedlings in a solution of 10 mg mL−1 trypan blue (Sigma) in water for 20 min. Then the leaves were washed three times using deionized water. The stained leaves were observed under a Quanta 200FEG microscope (Field Electron and Ion, USA) and OLYMPUS SZX10 microscope (Olympus, Japan).
Determination of reactive oxygen species (ROS) and malondialdehyde (MDA)
Samples were treated with 10 mol L−1 Na-EDTA to eliminate unbound Pb. Subsequently, 8 mL of pre-cooled phosphate buffer solution (PBS) was added to a 0.5 g plant sample in a pre-cooled mortar and pestle. Thoroughly homogenized samples were centrifuged at 12000 g at 4 °C for 20 min and supernatants, the enzyme extracts, were collected. ROS and MDA were determined following a previously published report with slight modifications [Citation14]. Briefly, The ROS level was determined by ROS Assay Kit (Beyotime, China). For MDA, 1.5 mL enzyme extracts and 2.5 mL mixture of 5% trichloroacetic acid (TCA) + 5% thiobarbituric acid (TBA) were incubated at 95 °C for 15 min then immediately cooled on ice. After short centrifugation at 4800 g, the absorbance of the MDA-TBA adduct was measured at 532 nm and 600 nm. The MDA content was determined using ε = 155 mM cm−1 and expressed as μmol·L−1·g−1 fresh weight [Citation14].
Determination of antioxidant activities
Plant leaves of different varieties were isolated and homogenized in PBS, then the supernatant was collected by centrifugation at 10000 g 4 °C for 10 min to detect the enzymatic activity. Superoxide dismutase (SOD), superoxide dismutase (SOD), peroxidase (POD), catalase (CAT), lipoxygenase (LOX) and polyphenol oxidase (PPO) activities as well as the proline (PRO) content in the supernatant were measured using a previously reported method [Citation15]. In detail, the POD activity was measured according to Klapheck et al. [Citation16]. The nitro blue tetrazolium (NBT) method was used to assay the SOD activity according to the methods of Giannopolitis and Ries [Citation17]. The PRO content was determined according to the method described by Shevyakova et al. [Citation18]. A method by Aebi was used to examine the CAT activity [Citation19]. PPO and LOX activity were determined as described by Ngadze et al. [Citation20]and Buranasompob et al. [Citation21], respectively.
Determination of ethylene (ETH), abscisic acid (ABA) and salicylic acid (SA) contents
Endogenous ETH, ABA and SA were extracted from leaves using the modified method of Llugany et al. [Citation22]. The content of ETH, ABA and SA was measured using the ETH (E-60049), ABA (E-60004) and SA (E-60046) ELISA kit, respectively, produced by Shanghai Jinma Experimental Equipment Co., Ltd.
cDNA-AFLP analysis
Total RNAs were extracted using the RNA extraction kit (Bio-Rad) and were inversely transcribed using the inverse transcription kit (Invitrogen, Carlsbad, CA). A total of 200 ng of cDNA was subjected to standard AFLP template production based on Ke’s report [Citation23]. The cDNA was first digested using MseI and EcoR I (NEB, England) then ligated to MseI and EcoR I adapters. Adapter ligated DNA acted as a template for pre-amplification, with PCR parameters of 30 cycles of 30 s at 94 °C, 60 s at 56 °C, and 60 s at 72 °C. Equal amounts of pre-amplified products were amplified using primers () with selective nucleotides at the 3′ end in a total volume of 20 μl. The first selective amplification cycle comprised 30 s at 94 °C, 30 s at 65 °C, and 60 s at 72 °C; annealing temperature was lowered by 0.7 °C per cycle during the next 12 cycles, followed by 23 cycles at 94 °C for 30 s, 56 °C for 30 s, and 72 °C for 60 s. All PCR reactions were performed in Applied Biosystem model 9902 Veriti thermal cycler. To each PCR product, 7.5 μl of formamide dye (98% formamide, 10 mmol L−1 EDTA, 0.005% xylene cyanol FF, and 0.005% bromophenol blue) was added, and 7 μl of each sample was loaded onto a pre-warmed 6% polyacrylamide gel using 1x Tris–borate–EDTA (TBE) buffer [Citation10, Citation23]. Thereafter, electrophoresis was run at 65 W for 2.5 h then the gels were silver stained using a silver staining kit (Promega cat. #Q4132, Madison, WI), according to the manufacturer’s instructions. The target bands were selected from the differential bans in the cDNA-APLP experiment, excised from the polyacrylamide gel and used as templates for re-amplification. The PCR products were purified with a kit (Tiangen, China), cloned into the pMD18-T vector (Takara, Dalina) and sequenced. After removal of the vector sequences, each transcript derived fragment (TDF) sequence was searched in the National Center for Biotechnology Information (NCBI) and SOL Genomics Network (SGN) databases [Citation24].
Table 1. Transcript-derived fragments (TDFs) identified in tomato plants following C. fulvum inoculation (NCBI data).
Quantitative real-time PCR analysis
Four TDFs were selected for qRT-PCR to verify the expression profiles obtained by cDNA-AFLP. The primers for the qRT-PCR were designed using primer 6.0 software (Supplemental Table S2). The qRT-PCR reaction was performed using an iQ5 system (Bio-Rad, USA). The real-time PCR used AceQⓇ qPCR SYBRⓇGreen Master Mix (Vazyme, USA). Each sample was replicated three times, and data were analyzed using the 2-ΔΔCT method with the EFα-1 as the reference for normalization [Citation5]. The following PCR protocol was used:95°Cfor 10 min and 40 cycles of 95 °C for 5 s, 59 °C for 15 s and 72 °Cfor 30 s. Subsequently, melting curve analysis was performed to detect primer dimerization and other artefacts of amplification.
Statistical analysis
Statistical significance was analyzed with t-tests using IBM SPSS Statistics (SPSS version 22.0; Armonk, NY), and the results were expressed as the means ± SD (standard deviation). Analysis of CAT, POD, SOD, PRO, LOX, PPO, ETH, ABA and SA was repeated three times, and similar results were obtained. Differences between groups were assessed by one-way analysis of variance (ANOVA).
Results
Trypan blue staining results
To analyze the molecular interactions between tomato and leaf mold fungus, lines displaying enhanced disease resistance and an exaggerated cell death response were screened after infection with C. fulvum Race 1.2.3.4. Cf-carrying plants grew normally up to 3 days after infection, whereas the MM showed remarkable lesions (). After 15 days, plants with Cf-5 and Cf-11 genes showed hypersensitive response; plants with Cf-19 gene showed lighter hypersensitive response, and the spots on MM leaves were blackened (). Trypan blue staining results showed that plants with a Cf gene had an apparent hypersensitive response (HR) to C. fulvum 72 h after inoculation (). MM exhibited compatible interaction (successful infection leading to disease) with C. fulvum that had not shown HR 72 h after inoculation (). The area of necrotic spots on the MM leaf was larger than that of the incompatible interaction (successful plant defense) tomatoes after 14 d of inoculation ().
Determination of ROS and MDA content
The content of ROS in Cf-19 and Cf-11 plants dramatically increased after C. fulvum inoculation and reached the peak on the 10th day (). The ROS content of Cf-5 plants increased significantly and reached a peak on the 15th day after infection with C. fulvum (). In the leaves of MM plants, the inoculation of C. fulvum caused a slow increase in ROS content with a peak on the 15th day (). The content of ROS in MM was the lowest of all the tomato lines at the same time point. The MDA content of all the tomato lines showed a gradual increasing trend. The highest MDA content was found in Cf-19 plants, whereas the lowest content was observed in MM ().
The activity of antioxidant enzymes
The antioxidant enzyme assays showed that plants with a Cf gene have some differences in the antioxidant enzyme activities as compared to those of MM plants. There were no notable differences in SOD activity among Cf-5, Cf-11, Cf-19 and MM plants () A significant increase was noticed in POD activity when Cf-11, Cf-19 and MM were inoculated with C. fulvum; while Cf-5 showed no significant impact by C. fulvum (). The content of PRO increased at first and then decreased, and there were obvious differences among different tomato varieties (). The PRO content of all the samples peaked at 15 d. The CAT activity was higher in Cf-5, Cf-11 and Cf-19, but moderate to weak activity was detected in MM (). The PPO activity increased at first and then decreased, and the PPO activity was lowest in MM plants (). The LOX activity was at a similar level in all non-inoculated plants. After inoculation, the LOX activity in MM showed an opposite behaviour to that of Cf -gene carrying plants (). Overall, except SOD, the activities of the most antioxidant enzymes in MM plants were lower than that in tomato plants carrying a Cf gene.
Figure 4. Antioxidant defense in tomato leaves 0–20 days after inoculation with C. fulvum. SOD activity (a), POD activity (b), PRO content (c), CAT activity (d), PPO activity (e) and LOX activity (f) of tomato plants after C. fulvum inoculation. Tomato lines: cf-5, Ontrio7516 (carrying the Cf-5 gene); cf-11, HN42 (carrying the Cf-11 gene); cf-19, HN19 (carrying the Cf-19 gene); and MM, Moneymaker (not carrying a Cf gene).
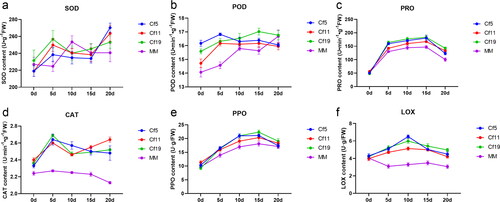
Determination of plant hormone
Ethylene (ETH) participates in plant development and regulates plant resistance to various stresses. When C. fulvum was not inoculated, the ETH content in the four varieties was at a similar level. However, after inoculation, the ETH content of all plants carrying a Cf gene increased, and the ETH content in Cf-19 significantly increased (p < 0.05). Nevertheless, the ETH content in MM plants kept fluctuating after inoculation (). Under stress conditions, ABA could regulate downstream genes to help plants adapt to stress. The ABA content in MM first increased then decreased with stress time. However, the ABA content in the other varieties did not show significant changes (). SA, the known disease resistance hormone, increased significantly and quickly after C. fulvum inoculation in Cf-5, Cf-19 and MM, but not in Cf-11 ().
cDNA-AFLP analysis and TDF detection
Each of the selective AFLP primer combinations amplified between 100 and 600 fragments, most of which were between 50 and 500 base pairs long. cDNA-AFLP analysis using 256 primer combinations resulted in the identification of more than 117 clear and unambiguous differentially expressed transcript-derived fragments (TDFs) (, ). Based on the characteristics of TDFs, 117 TDFS were subdivided into 11 types with differentially expressed polymorphic bands (). TDFs representing differentially expressed genes were classified into different categories based on their presence/absence (qualitative variation) or differences in expression levels. Among the differentially expressed TDFs, homologous sequences of 28 TDFS were found in the databases of the National Center for Biotechnology Information (NCBI) and SOL Genomics Network (SGN), while the rest were unknown (). According to the annotation information, the expression of ERF109 (TDF24), sedoheptulose-1,7-bisphosphatase (SBPase, TDF26), L-ascorbic acid oxidase (AAO, TDF27) and Thioredoxin H2 (TRX2, TDF48) were different between the plants with a Cf-gene and MM plants. To confirm the results of the cDNA-AFLP analysis, these four TDFs were validated by qRT-PCR (). Consequently, the results showed a consistent expression trend via cDNA -AFLP and qPCR.
Figure 6. Polyacrylamide gel electrophoresis of differentially expressed transcript-derived fragments (TDFs) in tomato plants following C. fulvum inoculation. Tomato lines: cf-5, Ontrio7516 (carrying the Cf-5 gene); cf-11, HN42 (carrying the Cf-11 gene); cf-19, HN19 (carrying the Cf-19 gene); and MM, Moneymaker (not carrying a Cf gene). Lanes 1–8: amplified bands of Cf5-CK, Cf11-CK, Cf19-CK, MM-CK, Cf5-Post inoculation, Cf11-Post inoculation, Cf19-Post inoculation and MM-Post inoculation by the primer combinations of E01/M03; Lanes 9–16: amplified bands of Cf5-CK, Cf11-CK, Cf19-CK, MM-CK, Cf5-Post inoculation, Cf11-Post inoculation, Cf19-Post inoculation and MM-Post inoculation by the primer combinations of E01/M05. CK stands for control, i.e. no C. fulvum inoculation. Post inoculation stands for 20 days after C. fulvum inoculation.
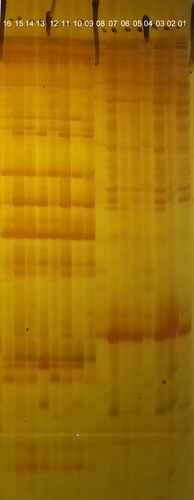
Figure 7. Quantitative real-time PCR (qRT-PCR) analyses of 4 selected genes. Tomato lines: cf-5, Ontrio7516 (carrying the Cf-5 gene); cf-11, HN42 (carrying the Cf-11 gene); cf-19, HN19 (carrying the Cf-19 gene); and MM, Moneymaker (not carrying a Cf gene). CK stands for control, i.e. no C. fulvum inoculation. Post inoculation stands for 20 days after C. fulvum inoculation.
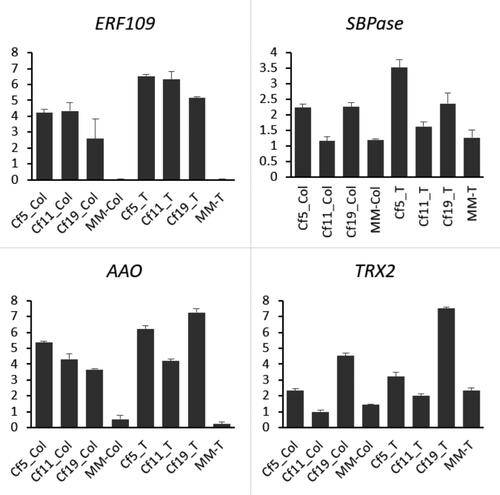
Discussion
The molecular mechanism of plant response to pathogens is a complex process. Nonetheless, the molecular mechanisms of different plants responding to pathogen infection are not similar. Tomato leaf mold caused by C. fulvum is an important disease, which has received significant research attention at Wageningen [Citation25]. Ietje Boukema developed near-isogenic lines of tomato in a Moneymaker background carrying the Cf-2, Cf-4, Cf-5, or Cf-9 genes for resistance against C. fulvum [Citation26]. In disease-resistant plants, the changes in ROS were similar to the trend in our previous report [Citation27]. We found that the first rapid increase of ROS occurred on the 5th or 10th day after inoculation, coinciding with the time when necrotic spots appeared on the leaves; in sensitive tomatoes, the accumulation of ROS reached a peak at 15 d after inoculation. The ROS content in resistant varieties was higher than that in the sensitive tomato plants, indicating that it should act as a signal molecule to initiate the disease resistance response [Citation28,Citation29]. MDA is an end product of lipid peroxidation, and increased MDA levels reflect oxidative stress. These results also indicate that C. fulvum inoculation increased ROS which stimulated the response of antioxidant defenses and decreased SOD, POD, CAT, PPO and LOX activities and the PRO level. Increased levels of activities of SOD, POD, CAT, PPO and LOX and PRO levels, indicate tissue healing after C. fulvum inoculation [Citation30].
C. fulvum infection induced the increase of antioxidant enzyme activities, which might be attributed to the increase in O2- in the leaves exceeding the removal capacity of the defense system, further leading to lipid membrane peroxidation [Citation31]. Our findings that LOX and CAT activity was lowest in MM tomato plants suggest that LOX and CAT may play a role in cellular antioxidant stress. Meanwhile, the symptoms of necrotic spots on the inoculated leaves showed that cell death was intensified at this time. The activities of LOX and CAT in Cf-gene carrying tomato plants increased in a time-dependent manner. We speculate that superoxide anion might play a major role in cell death.
In the plant hormones assays, we found that ETH, ABA and SA were the hormones that significantly changed in response to C. fulvum infection. After the plant was infected by C. fulvum, the ABA content in sensitive tomato plants was significantly higher than that in the resistant tomato lines, indicating that ABA probably inhibited the biological stress response caused by C. fulvum. Of note, biotic and abiotic stresses could lead to an increase in SA and ETH [Citation32,Citation33]. Therefore, SA and ETH may be involved in the plant defense mechanisms against biological stress. In the cDNA-AFLP analysis results, we identified four TDFs related to plant resistance. A previous study demonstrated that the expression level of VaERF092 and AtERF105 showed altered expression to cold stress; their expression reached a peak at the early stages of cold stress and then decreased [Citation34]. In plants, ERF109 acts under salt stress in inducing ROS scavengers, and as a ‘master switch’ mediator in promoting plant growth and re-adjustment to homeostasis due to the direct participation in auxin biosynthesis. These actions combine to increase the capacity of the plant to tolerate stress [Citation35]. Also, the reanalyzed transcriptome data confirmed that ERF109 expression and auxin biosynthesis were synergistic. Yang et al. reported that ERF109 mRNA abundance was quickly up-regulated by ethylene [Citation36]. In the ethylene signalling pathway, ERF plays a key role in signal transduction [Citation37]. ERFs like JERF1, OsERF1 and VaERF057 could be induced by ethylene, suggesting their potential roles in orchestrating the stress tolerance regulation [Citation38–41]. Based on our results, the synergistic effect of ERF109 mRNA and ETH hormone confirmed that tomato plants with the Cf gene were more resistant than MM plants. Additionally, AAO was highly expressed in response to C. fulvum infection. The reanalyzed transcriptome data revealed that the ABA signals were active after C. fulvum infection. Summarily, we believe that plant hormone signal transduction is the primary pathway for tomato plants to respond to biotic stress. Nonetheless, the SA related genes were not significantly expressed in the cDNA-AFLP analysis; this may be attributed to the insensitivity of cDNA-AFLP analysis.
Furthermore, our findings identified that TRX was activated and expressed by C. fulvum infection. TRX is a type of multifunctional acidic protein existing widely in organisms; it participates in a series of biochemical reactions in cells, such as regulating enzymatic activity and transcription factors. Xia et al. [Citation42] revealed that the TRX M1 gene has a certain correlation with biological environmental stress, particularly in oxidative stress, which enhances the tolerance of plants to adversity. In the process of leaf mold interaction, the outbreak of ROS and the process of systemic acquired resistance (SAR) are tightly combined with thioredoxin. Thus, thioredoxin probably regulates the process of Cf-19-mediated disease resistance.
Conclusions
In this study, the ROS content in Cf-19 tomato plants was the most sensitive to C. fulvum inoculation, whereas in the MM plants it was the least. Plants with a Cf gene had higher antioxidant enzyme activities than MM plants. The expression levels of ERF109, sedoheptulose-1,7-bisphosphatase (SBPase), L-ascorbic acid oxidase (AAO) and Thioredoxin H2 (TRX2) are different between plants with a Cf-gene and MM plants. In the process of leaf –mold interaction, the outbreak of ROS and the production of SAR resistance were tightly combined with antioxidant enzymes and thioredoxin. Tomatoes carrying the Cf-19 gene appeared to have stronger resistance to C. fulvum Race 1.2.3.4.
Authors’ contributions
GL performed the experiment; DZ, TZ, HY, JJ contributed tomato planting; GL, JL, HZ, XX contributed significantly to analysis; GL, XH contributed manuscript preparation;
Supplemental Material
Download Zip (16.9 KB)Data availability statement
All data that support the findings reported in this study are available from the corresponding author [XH Hu] upon reasonable request.
Disclosure statement
The authors report no conflict of interest.
Additional information
Funding
References
- Wang H, Shi Y, Wang D, et al. A biocontrol strain of Bacillus subtilis WXCDD105 used to control tomato Botrytis cinerea and Cladosporium fulvum cooke and promote the growth of seedlings. IJMS. 2018;19(5):1371.
- Thomma BPHJ, VAN Esse HP, Crous PW, et al. Cladosporium fulvum (syn. Passalora fulva), a highly specialized plant pathogen as a model for functional studies on plant pathogenic mycosphaerellaceae. Mol Plant Pathol. 2005;6(4):379–393.
- Stergiopoulos I, de Wit PJ. Fungal effector proteins. Annu Rev Phytopathol. 2009;47:233–263.
- Yoshida K, Asano S, Sushida H, et al. Occurrence of tomato leaf mold caused by novel race 2.4.9 of Cladosporium fulvum in Japan. J Gen Plant Pathol. 2021;87(1):35–38.
- Xue D-Q, Chen X-L, Zhang H, et al. Transcriptome analysis of the Cf-12-mediated resistance response to Cladosporium fulvum in tomato. Front Plant Sci. 2016;7:2012.
- Zhao T, Liu G, Li S, et al. Differentially expressed gene transcripts related to the Cf-19-mediated resistance response to Cladosporium fulvum infection in tomato. Physiol Mol Plant Pathol. 2015;89(Complete):8–15.
- Banikamali M, Soltanloo H, Ramezanpour SS, et al. Identification of salinity responsive genes in lavender through cDNA-AFLP. Biotechnol Rep (Amst). 2020;28:e00520–e00520.
- Bachem CW, van der Hoeven RS, de Bruijn SM, et al. Visualization of differential gene expression using a novel method of RNA fingerprinting based on AFLP: analysis of gene expression during potato tuber development. Plant J. 1996;9(5):745–753.
- Akihiro T, Umezawa T, Ueki C, et al. Genome wide cDNA-AFLP analysis of genes rapidly induced by combined sucrose and ABA treatment in rice cultured cells. FEBS Lett. 2006;580(25):5947–5952.
- Vuylsteke M, Peleman JD, van Eijk MJ. AFLP-based transcript profiling (cDNA-AFLP) for genome-wide expression analysis. Nat Protoc. 2007;2(6):1399–1413.
- Durrant WE, Rowland O, Piedras P, et al. cDNA-AFLP reveals a striking overlap in race-specific resistance and wound response gene expression profiles. Plant Cell. 2000;12(6):963–977.
- Dubos C, Plomion C. Identification of water-deficit responsive genes in Maritime pine (Pinus pinaster ait.) roots. Plant Mol Biol. 2003;51(2):249–262.
- Wang X, Tang C, Zhang G, et al. cDNA-AFLP analysis reveals differential gene expression in compatible interaction of wheat challenged with Puccinia striiformis f. sp. Tritici. BMC Genomics. 2009;10:289.
- Kaur G, Singh HP, Batish DR, et al. Exogenous nitric oxide (NO) interferes with lead (Pb)-induced toxicity by detoxifying reactive oxygen species in hydroponically grown wheat (Triticum aestivum) roots. PLoS One. 2015;10(9):e0138713.
- Abbas M, Imran F, Iqbal Khan R, et al. Gibberellic acid induced changes on growth, yield, superoxide dismutase, catalase and peroxidase in fruits of bitter gourd (Momordica charantia L.). Horticulturae. 2020;6(4):72.
- Klapheck S, Zimmer I, Cosse H. Scavenging of hydrogen peroxide in the endosperm of Ricinus communis by ascorbate peroxidase. Plant Cell Physiol. 1990;31(7):1005–1013.
- Giannopolitis CN, Ries SK. Superoxide dismutases: I. Occurrence in higher plants. Plant Physiol. 1977;59(2):309–314.
- Shevyakova N, Bakulina EA, Kuznetsov V. Proline antioxidant role in the common ice plant subjected to salinity and paraquat treatment inducing oxidative stress. Russ J Plant Physiol. 2009;56(5):663–669.
- Aebi H. Catalase in vitro. Methods Enzymol. 1984;105:121–126.
- Ngadze E, Icishahayo D, Coutinho TA, et al. Role of polyphenol oxidase, peroxidase, phenylalanine ammonia lyase, chlorogenic acid, and total soluble phenols in resistance of potatoes to soft rot. Plant Dis. 2012;96(2):186–192.
- Buranasompob A, Tang J, Powers JR, et al. Lipoxygenase activity in walnuts and almonds. LWT - Food Sci Technol. 2007;40(5):893–899.
- Llugany M, Martin SR, Barceló J, et al. Endogenous jasmonic and salicylic acids levels in the cd-hyperaccumulator Noccaea (Thlaspi) praecox exposed to fungal infection and/or mechanical stress. Plant Cell Rep. 2013;32(8):1243–1249.
- Ke L, Luo B, Zhang L, et al. Differential transcript profiling alters regulatory gene expression during the development of Gossypium arboreum, G.stocksii and somatic hybrids. Sci Rep. 2017;7(1):3120–3120.
- Zhang D, Xie L, Xu X. cDNA-AFLP analysis of salicylic acid- and calcium chloride-induced transcript derived fragments under drought in tomato (Solanum lycopersicum). Biotechnol Biotechnol Equip. 2020;34(1):587–594.
- de Wit PJGM. Cladosporium fulvum effectors: weapons in the arms race with tomato. Annu Rev Phytopathol. 2016;54(1):1–23.
- Kerr EA, Bailey DL. Breeding for resistance to Cladosporium fulvum in tomato. Acta Hortic. 1966;(4):145–148. (In Belgium)
- Liu G, Liu J, Zhang C, et al. Physiological and RNA-seq analyses provide insights into the response mechanism of the Cf-10-mediated resistance to Cladosporium fulvum infection in tomato. Plant Mol Biol. 2018;96(4–5):403–416.
- Tiwari BS, Belenghi B, Levine A. Oxidative stress increased respiration and generation of reactive oxygen species, resulting in ATP depletion, opening of mitochondrial permeability transition, and programmed cell death. Plant Physiol. 2002;128(4):1271–1281.
- Jabs T. Reactive oxygen intermediates as mediators of programmed cell death in plants and animals. Biochem Pharmacol. 1999;57(3):231–245.
- Carden DL, Granger DN. Pathophysiology of ischaemia-reperfusion injury. J Pathol. 2000;190(3):255–266.
- Ayala A, Muñoz MF, Argüelles S. Lipid peroxidation: production, metabolism, and signaling mechanisms of malondialdehyde and 4-hydroxy-2-nonenal. Oxid Med Cell Longev. 2014;2014:360438–360438.
- Trivedi VD, Jangir PK, Sharma R, et al. Insights into functional and evolutionary analysis of carbaryl metabolic pathway from Pseudomonas sp. strain C5pp. Sci Rep. 2016;6:38430–38430.
- Wei J, Zhang Z, Zhang P, et al. Regulation of ethylene biosynthesis and signal transduction by nitric oxide leading to resistance against Alternaria alternata in Hami melon. J Sci Food Agric. 2022;102(9):3535–3542.
- Bolt S, Zuther E, Zintl S, et al. ERF105 is a transcription factor gene of Arabidopsis thaliana required for freezing tolerance and cold acclimation. Plant Cell Environ. 2017;40(1):108–120.
- Bahieldin A, Atef A, Edris S, et al. Multifunctional activities of ERF109 as affected by salt stress in Arabidopsis. Sci Rep. 2018;8(1):6403.
- Yang C, Lu X, Ma B, et al. Ethylene signaling in rice and Arabidopsis: conserved and diverged aspects. Mol Plant. 2015;8(4):495–505.
- Liu M, Pirrello J, Chervin C, et al. Ethylene control of fruit ripening: revisiting the complex network of transcriptional regulation. Plant Physiol. 2015;169(4):2380–2390.
- Zhang H, Huang Z, Xie B, et al. The ethylene-, jasmonate-, abscisic acid- and NaCl-responsive tomato transcription factor JERF1 modulates expression of GCC box-containing genes and salt tolerance in tobacco. Planta. 2004;220(2):262–270.
- Hu Y, Zhao L, Chong K, et al. Overexpression of OsERF1, a novel rice ERF gene, up-regulates ethylene-responsive genes expression besides affects growth and development in Arabidopsis. J Plant Physiol. 2008;165(16):1717–1725.
- Sun X, Zhao T, Gan S, et al. Ethylene positively regulates cold tolerance in grapevine by modulating the expression of ETHYLENE RESPONSE FACTOR 057. Sci Rep. 2016;6:24066.
- Wang M, Dai W, Du J, et al. ERF109 of trifoliate orange (Poncirus trifoliata (L.) raf.) contributes to cold tolerance by directly regulating expression of Prx1 involved in antioxidative process. Plant Biotechnol J. 2019;17(7):1316–1332.
- Xia D. The functional of thioredoxin genes in Arabidopsis thaliana under environmental stress. Beijing: Northeast Forestry University; 2007.