Abstract
Cucumber (Cucumis sativus L.) is sensitive to the salinization of environment. Soil salinization in facility environment affects the yield and quality of cucumber. Melatonin (N-acetyl-5-methoxytryptamine) is an effective free radical scavenger in plants and could effectively alleviate the abiotic stress injury of plants. In this study, we investigated the effect of different concentrations of exogenous melatonin in mitigating salt stress in cucumber and some underlying molecular mechanisms. The results indicated that 100 μmol/L melatonin-treated plants increased significantly the content of the content of ascorbic acid (ASA), proline, glutathione (GSH), polyphenol and flavonoid, and superoxide dismutase (SOD) and peroxidase (POD) activity, soluble protein and sugars. We utilized RNA-seq technology to analyse the transcriptome profile of leaf tissues under M0 and M2 treatments. The analysis identified 699 differentially expressed genes (DEGs) with 506 up-regulated and 193 down-regulated genes. Most of the transcription factors identified were also involved in plant stress defense. Gene Ontology (GO) enrichment analysis of the DEGs showed significant enrichment of 328 GO terms (p ≤ 0.05). Kyoto Encyclopedia of Genes and Genomes (KEGG) analysis showed that the DEGs were significantly enriched in 63 KEGG pathways (p ≤ 0.05). Taken together, these results provided a valuable reference for exploring the mechanism of action of exogenous melatonin in cucumber in saline environment.
Introduction
Soil salinization is a widespread phenomenon, and one of the major threats to soil degradation worldwide [Citation1], especially in facility greenhouses. The soil in the facility remains in a closed environment for a long time, lacking of natural rain leaching, the cultivated crop is typically single, and fertilization for a long time leads to the soil salinization in the facility becoming more and more serious [Citation2,Citation3]. The salinization is mainly due to the accumulation of sodium ions (Na+) and chloride ions (Cl-) in the soil. The excessive accumulation of Na+ limits the hydraulic conductivity and soil porosity, and affects the growth of plant roots and plant growth [Citation4]. Thus, the changes in the soil properties, in turn, affect the growth and development of plants.
As the global climate changes, the degree of salt stress in many regions is expected to further increase [Citation5]. Salt stress is the main abiotic stress that restricts the growth and development of plants and limits the development of agriculture. Salt-induced stress pathways include osmotic stress, ion stress and secondary stress [Citation6]. In addition, reactive oxygen species (ROS) produced by plants under salt stress, such as hydroxyl free radicals, hydrogen peroxide and superoxide anions, accumulate in plant cells and damage the cell structure, proteins, lipids, nucleic acids and may also induce programmed cell death [Citation7–9]. Moreover, salt stress leads to a decline in the output of important crops such as corn and rice [Citation10]. Salt treatment has a significant inhibitory effect on the yield and quality of wheat [Citation11]. Prior to this study, we found that the soil in the test area was saline, which inspired our research on the salt tolerance of plants.
In recent years, exogenous substances have been widely used to improve the stress resistance of plants. Researchers have explored the potential of spraying exogenous hormones to alleviate the negative effects of salinization on plants. For example, seeds pretreated with melatonin could show improved resistance to low temperature stress by detoxifying ROS [Citation12]. Ren et al. [Citation13] found that melatonin application promotes plant growth under NaCl treatment. Some studies have reported that treatment with exogenous melatonin could improve the antioxidant capacity of plants [Citation14–16].
Cucumber (Cucumis sativus L.) is a one of the facility vegetables with the largest cultivated area, and is also a crop sensitive to salinization in the cultivation environment. Previous studies have shown that melatonin could alleviate the inhibitory effect of NaCl stress on cucumber seed germination [Citation17], but the mechanism of action is still unclear. Cucumber seedling stage is a critical period of growth and development; seedlings are sensitive to the cultivation environment. It is of great significance to explore the growth regulation of cucumber seedlings under salt stress. For this study, cucumber cultivar ‘Xintaimici’ was used to study the effects of different concentrations of melatonin on the content of osmotic substances and oxidoreductase activity in cucumber seedlings under salt stress, and to screen the optimal melatonin concentration to improve salt stress. In addition, transcriptome sequencing was performed to analyze some of the molecular mechanisms underlying the action of melatonin.
Materials and methods
Plant materials and treatments
The cucumber (Cucumis sativus L.) seed ‘Xintai Mizhi’ selected in this experiment is a variety commonly used in production. Cucumber seeds were immersed in warm water at 55 °C, were incubated on damp filter paper for 2 days. The germinated seedlings were selected and grown in 54 cm × 28 cm trays filled with a mix consisting of peat: vermiculite: perlite (2:1:1). The seedlings (60 seedlings and 3 trays per treatment) were grown at a 16 h light/8 h dark cycle, 60% humidity and 26 °C/18 °C during day/night in the sunlight greenhouse of Shanxi Agricultural University gardening cultivation base (N37°, E112°). One week later, these seedlings were divided into five groups: 0 μmol/L melatonin (M0), 50 μmol/L melatonin (M1), 100 μmol/L melatonin (M2), 150 μmol/L melatonin (M3) and 200 μmol/L melatonin (M4). The melatonin treatment was applied by spraying a 10 mL solution every other day around 8 p.m.; the M0 (control) group was sprayed with water instead of melatonin. Two weeks after that, the seedlings were cultured in a nutrient matrix with 150 mmol/L NaCl. After 15 days of saline treatment, physiological and biochemical parameters were measured. Each treatment was performed in three replicates for a total of 60 plants.
Determination of related enzyme activity
The physiological and biochemical parameters were determined in the fully expanded third true leaf from the top of the cucumber plants. For the enzyme assays, 0.5 g of leaf mass was ground with mortar and pestle in liquid nitrogen with 10 mL phosphate buffed saline (PBS) (pH 7.0), the homogenate was centrifuged at 12000 r/min (HC-2518R), 4 °C for 15 min, and then the supernatant was taken to measure the enzyme activity. All steps of enzyme extract preparation were carried out at 4 °C. The extracts were used to measure superoxide dismutase (SOD) activity, peroxidase (POD) activity and soluble protein content based on the method described by He et al. [Citation18]. Proline content and soluble sugars were measured using a modification of the procedures of Bates et al. [Citation19] and Zhang et al. [Citation20]. To determine the proline content, approximately 0.5 g of plant material was homogenized in 5 mL of 3% aqueous sulfosalicylic acid and boiled at 100 °C for 15 min, and the homogenate was filtered through filter paper. Then, 0.5 mL of filtrate was reacted with 1.5 mL water, 2 mL of glacial acetic acid and 2 mL acidic ninhydrin in a test tube for 30 mins at 100 °C, and the reaction was terminated in an ice bath. The reaction mixture was extracted with 5 mL toluene and was mixed vigorously with a test tube stirrer for 30 s. The chromophore containing toluene was aspirated from the aqueous phase, and the absorbance was read at 520 nm using toluene for a blank.
The content of ascorbic acid (ASA) was measured in extracts according to the method of Jiang et al. [Citation21]. The absorbance values at 340 nm and 412 nm were measured by UV2600 ultraviolet/visible spectrophotometer, and the glutathione S-transferase (GST) activity and reduced glutathione (GSH) content of the samples were calculated, respectively [Citation22].
The flavonoid content was determined according to the aluminum chloride colorimetric method as described by Mohd et al. [Citation23]. The total polyphenol content was determined according to Alina et al. [Citation24] with minor modification. Briefly, approximately 0.8 g of plant material was homogenized in 5 mL of 60% absolute ethanol and extracted in ultrasound for 1 h. The homogenate was centrifuged at 12000 r/min (HC-2518 R) at 4 °C for 10 min. The extract was obtained after two repetitions. Two milliliters of filtrate were allowed to react with 2.5 mL of 20% Na2CO3 and 6.0 mL water; then 0.5 mL of Folin-Ciocalteu reagent was added. The reaction was incubated in a dark environment for 2 h, and the absorbance was determined at 725 nm.
Each assay was performed in three independent replicates.
Total RNA extraction, transcriptome assembly and annotation
Cucumber seedlings at the same leaf position were taken as the experimental materials after 15 days of melatonin treatment, and the sampling time was uniformly chosen at 10 a.m. on sunny days, where only two treatment samples with the most obvious difference were gathered. The leaves were ground into a powder in liquid nitrogen, total RNA was isolated from four samples respectively using TIANGEN reagent and anhydrous ethanol, following the instructions of the kit. The total RNA concentration, purity, and integrity were analyzed by a nucleic acid detector; the A260/A280 ratio ranged from 1.8 to 2.1, indicating that the samples were of sufficient integrity. Four biological replicates were selected per treatment for transcriptome sequencing. Library preparation and RNA-Seq techniques were carried out at Hangzhou LianChuan Biotechnology (Hangzhou, China). The RNA-Seq data are available at: www.ncbi.nlm.nih.gov/bioproject/PRJNA871805.
The quality of raw data was evaluated after sequencing. Then the high quality reads were compared with the reference genome (Cucumber (Chinese Long) v3.0 Genome) to perform differential gene expression analysis among multiple samples. We further analyzed the transcripts by matching them against the Gene Ontology (GO) database and the Kyoto Encyclopedia of Genes and Genomes (KEGG).
Real-time quantitative polymerase chain reaction (PCR)
To validate the reliability of DEGs data obtained from the transcriptome sequencing in cucumber, 6 DEGs were randomly selected for qRT-PCR determination. Primers for qRT-PCR of each gene were devised using the Primer 5 online tool, the gene and primer sequences are shown in . The total RNA samples from leaves of control and treatment groups were reverse-transcribed to cDNA using PrimeScript™ RT reagent Kit (TaKaRa, Code No. RR036A). Quantitative real-time PCR amplifications were performed using the TB Green™ Premix Ex Taq™ II (TaKaRa, Code No. RR820A). The qRT-PCR cycling program was: 94 °C for 30 s, followed by 40 cycles of 94 °C for 30 s and 56 °C for 30 s, and 72 °C for 30 s using an ABI 7500 real-time PCR instrument. All reactions were performed in a 25 μL volume containing 20 ng of diluted cDNA. Three biological replicates and three technical repeats were implemented in qRT-PCR. The relative expression ratio of the selected genes was calculated by the 2-△△Ct method [Citation25].
Table 1. List of primers used in the present study.
Statistical analysis
Statistical analyses were performed using SPSS 22.0. The data were checked for normality and homogeneity of variance via the Kolmogorov-Smirnov test and Levene’s test, respectively, prior to multiple comparisons. The differences in the relative enzyme activity values between the control and melatonin treatments were assessed using one-way analysis of variance (ANOVA), followed by least significant difference multiple comparison tests. Data are mean values with standard error. Differences were considered statistically significant at the p < 0.05 level.
Results
Biochemical parameters in cucumber with different melatonin treatments under salt stress
The activity of GST, the content of ASA, proline, GSH, polyphenol, flavonoid, soluble protein and soluble sugars were biochemical endpoints to observe the response of cucumber seedlings to different concentrations of melatonin in saline environment. The ASA content of cucumber seedlings was significantly increased under exogenous melatonin treatment (). Melatonin treatment of M2 plants (100 μmol/L) had a significant effect (one-way ANOVA; F = 43.98; df = 4, 10; p<0.0001): ASA was 127.8% higher in M2 plants than in the control (M0). The content of proline showed a significant change in M2 treatment (), with a value 10.8% higher than the control. There was no significant difference between M1 (50 μmol/L), M4 (200 μmol/L) and the control (one-way ANOVA; F = 23.43; df = 4, 10; p<0.0001).
Figure 1. Effects of different concentrations of melatonin on ASA (A), proline (B), GST (C), GSH (D), SOD (E), POD (F), polyphenol (G), flavonoid (H), souble protein (I) and soluble sugars (J) in leaves of salt stressed cucumbers. Data are presented as means ± SD. Different letters (a, b, c, d) above the error bars indicate significant differences among the treatments (p < 0.05).
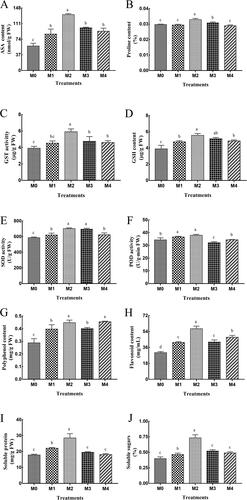
GST and GSH are important antioxidants that maintain the antioxidant balance of cells under stress. The trend of GST content was consistent with ASA (); the GST content of M2 cucumber seedlings was significantly higher than that of the control, M3 (150 μmol/L) and M4 treatments (one-way ANOVA; F = 12.46; df = 4, 10; p = 0.0007). The GSH content of cucumber increased after treatment (); the effects in M2 and M3 groups were more pronounced than that in the control, and groups M1 and M4 followed; the difference was significant (one-way ANOVA; F = 18.36; df = 4, 10; p = 0.0001).
As shown in , the SOD activity in treated cucumber seedlings was significantly higher than that in the control; M2 and M3 groups showed the best treatment effect (one-way ANOVA; F = 26.59; df = 4, 10; p<0.0001). In , the POD activity in M1 and M2 groups was higher than the control, and lower in group M3 and M4 than in the control (one-way ANOVA; F = 17.86; df = 4, 10; p = 0.0002).
It is known that the polyphenol content of cucumber is elevated by exogenous melatonin pretreatment. In comparison to the control, the polyphenol content increased by 37.9%, 55.2%, 39.1% and 57.9% in M1, M2, M3 and M4 groups, respectively (). Meanwhile, the trend of the flavonoid content was consistent with the polyphenol content, and the effect of M2 treatment was the most pronounced (), with a 90% higher flavonoid content than the control (one-way ANOVA; F = 68.82; df = 4, 10; p<0.0001).
The soluble protein and soluble sugars of M2 group increased by 59% and 83% compared to the control under exogenous melatonin treatment, respectively. M3 and M4 groups showed no significant difference from the control. The soluble protein and soluble sugars in the M1 group increased by 23% and 16% (p = 0.05), in contrast with the control, respectively ( and J).
Transcriptome sequence and assembly
The cDNA samples with four duplicates were collected from control and treatment groups. We utilized the Illumina HiSeq 4000 platform with cDNA libraries of ‘Chinese long’ cucumber v3.0 [Citation26]. After removing the redundant and short reads, we obtained 44,997,544 (94.83%), 40,0317,209 (94.07%), 47,110,894 (95.17%) and 35,821,896 (96.07%) clean reads from the control; 37,520,190 (95.80%), 33,538,824 (96.21%), 40,628,276 (96.14%) and 32,872,298 (96.28%) clean reads from the melatonin-treated groups (Supplementary Table S1). The result indicated that the quality scores of Q20 and Q30 in each sample were more than 99% and 97.5%, respectively; the GC content for the melatonin-treated groups and the control were all greater than or equal to 42%.
Sequencing of cDNA samples yielded an average of 41 million (97.7%) mapped reads in the control and 35 million (98.0%) mapped reads in the melatonin-treated groups. The number of unique mapped reads were 35,983,297 (85.7%) in control and 31,090,241 (86.0%) in melatonin-treated groups. The average number of multi mapped reads were 5,038,214 (12.0%) to 4,321,502 (12.0%) in the control and treatment groups, respectively. The average number of PE mapped reads in the control and treatment groups were 33,939,320.5 (81.0%) and 29,369,407.5 (81.0%), respectively (Supplementary Table S2).
Identification and analysis of DEGs
To identify DEGs in the control and melatonin-treated groups, comparisons between the control (M0) and each treatment group were performed using the DESeq method. Among the melatonin treatments, group M2 was selected for further analysis. The Volcano plot depicted that 699 DEGs had significantly changed expression level, 506 DEGs were significantly up-regulated in M2, and 193 DEGs were significantly down-regulated (). Using fold change ≥2 or ≤0.5, p-value <0.05 as thresholds, 489 transcripts (362 up-regulated and 127 down-regulated by exogenous melatonin treatment) were identified as DEGs. In the present study, many transcription factors were differentially expressed after cucumber seedlings were exposed to salt stress. The results showed that the number of DEGs in bHLH, NAC, MYB and ERF families was relatively higher than other families (), which suggested that these transcription factors may play important roles in the response of cucumber seedlings to salt stress.
GO and KEGG enrichment analysis of DEGs
To assess the biological roles of the DEGs, we carried out enrichment analysis of GO classification. The GO enrichment data indicated that GO terms were divided into three main categories. The major DEGs were in molecular function (MF), 641 DEGs; the biological processes (BP) term had 438 DEGs; and the cellular component (CC) term had 146 DEGs. The BP category consisted of 25 parts including 438 genes (), with the main ones involved in oxidation-reduction processes, protein phosphorylation, regulation of transcription, DNA-template and transmembrane transport. The CC category consisted of 15 parts containing 146 genes, mainly in these several aspects: integral component of membrane, membrane and transcription factor complex. The MF category contained 10 parts, with a total of 641 genes. And the most significant part was ATP binding. These analyses indicated the involvement of multiple molecular functions, cellular components and biological processes in the response of cucumber seedlings to melatonin treatment and salt environment. This provided a significant basis for exploring the possible function of DEGs.
Pathway enrichment analysis allowed deep biological recognition of DEGs functions. A total of 327 DEGs were divided into 17 pathways, of which 225 genes were up-regulated and 102 genes were down-regulated (). There were 17 important pathways including 111 up-regulated genes and 37 down-regulated genes (Supplemental Table S3). The pathways included Flavonoid biosynthesis, Glutathione metabolism, Carotenoid biosynthesis, MAPK signaling pathway and so on, which revealed their key role in response to melatonin treatment.
Validation of RNA-Seq data by qRT-PCR
To verify the accuracy of the obtained RNA-Seq data, six genes were randomly selected for RT-qPCR validation. The expression data of the six genes analyzed by RT-qPCR exhibited similar expression trends to the corresponding FPKM values in the RNA-seq data (). Among these DEGs, Csa1G150、Csa3G160、Csa1G440、Csa2G050、Csa2G530、Csa4G610 were up-regulated. Therefore, the relative expression of all six genes was in accordance with the trend of FPKM values, the accuracy of RNA-seq data was confirmed to be reliable.
Discussion
The survival of plants depends to a large extent on the ability to adapt to changes in the external environment, and also affects the growth and development of plants. The accumulation of ROS in plants associated with the environmental stresses (oxidative stress) adversely affects plant growth [Citation27]. Osmotic stress caused by salt stress strictly reduces the water conductivity of plant roots [Citation28], the plant height and fresh weight of rapeseed [Citation29]. Salt stress could lead to the accumulation of ROS in plants and can damage the cell membrane, which is mainly composed of proteins and lipids [Citation30,Citation31].
Melatonin was the first line of defense in plants against environmental oxidative stress, which could regulate plant growth and development and the defense against various environmental stresses [Citation32]. It has been shown in the literature that melatonin plays an important regulatory role in plant responses to biotic and abiotic stresses; it could scavenge free radicals and improve the antioxidant capacity in plants [Citation33,Citation34]. The study of Chen et al. [Citation35] found that slight differences in the antioxidant enzyme activities of corn seedlings were observed between different concentrations of melatonin. Melatonin treatment also had a beneficial effect on the antioxidant capacity and improved the salt tolerance in maize seedlings [Citation36]. The high activities of SOD and POD under salt stress in melatonin-treated plants support the suggestion that exogenous melatonin application can alleviate, at least in part, the damage that salt stress causes on the antioxidant capacity of cucumber seedlings. Similarly, Zhang et al. [Citation32] found that the antioxidant enzyme activities of cucumber seedlings under salt stress were increased after exogenous melatonin pretreatment. In addition, Reiter et al. [Citation37] found that melatonin was present in walnuts; it could increase blood melatonin concentrations when eaten; serum trolox equivalent antioxidant capacity and ferric reducing ability was enhanced. The AsA-GSH cycle in higher plants is an important antioxidant system that protects plants from oxidative stress. Melatonin treatment maintains higher levels of ascorbic acid and glutathione in the plant [Citation38,Citation39]. Melatonin treatment ameliorated salt stress-induced oxidative damage and further enhanced salt stress resistance ().
Under environmental stress, the accumulation of ROS in plants can lead to the destruction of cell membrane integrity and cell death [Citation35]. Theoretically, the addition of melatonin can alleviate the oxidative damage caused by stress by directly removing ROS [Citation40]. Moreover, osmoregulation substances play an important role in improving plant stress resistance [Citation41]. The study shows that melatonin and tryptophan treatments alleviated the detrimental effects of water deficiency and accelerated the recovery mainly via improving the tolerance of white lupine plants in terms of enhancing the content of osmotic regulators and antioxidant capacity [Citation42]. According to these reports, our study showed that melatonin significantly induced the content of specific organic solutes such as polyphenol、flavonoid、soluble protein and soluble sugars under salt stress (–J). It may be that various metabolic processes of plant cells were disturbed by the stress environment [Citation33,Citation43].
Li et al. [Citation44] found that melatonin could improve the stress resistance of maize seedlings. The same phenomenon has been found in Citrus aurantium [Citation45]. In the present study, exogenous melatonin treatment increased the content of osmotic regulators and the activity of related antioxidant enzymes in cucumber seedlings (), which was in accordance with the results of Gao et al. [Citation46], who found that exogenous melatonin increased SOD and POD. Furthermore, the effect of 100 μmol/L melatonin treatment was most pronounced. There are similar reports in watermelon [Citation47], maize [Citation36] and Naked oat [Citation46]. The responses shown in this work, in terms of melatonin treatment and antioxidant systems such as metabolite levels (ascorbic acid, glutathione and phenolic compounds) and enzymes (superoxide dismutase, peroxidase, ascorbic acid, glutathione reductase, etc.) would assist to understand the mechanism of action of melatonin in relieving salt stress in cucumber.
The effects of melatonin on the antioxidant capacity of plants have been studied widely. Previous studies have shown that high expression of transcription factors such as MYB, NAC, WRKY and bHLH was promoted after melatonin treatment of Bermudagrass [Citation32,Citation48]. The transcript levels of many kinases and some stress-related calcium signals are up-regulated. Weeda et al. [Citation49] have shown that melatonin treatment can induce the expression of multiple genes in Arabidopsis, including NAC, ERF and bZIP. The same transcription factors were also identified in our study, where 1.82%, 72.91%, 76.09% and 84.09% of the genes in the bHLH, NAC, MYB and ERF families showed an upward trend, respectively.
Conclusions
Many studies have found that melatonin, as a novel plant growth regulator, plays an important role in alleviating plant stress. The present study investigated the mitigating effect of melatonin on salt stress in cucumber. The scavenging of ROS, the content of ASA, proline, GSH, polyphenol and flavonoid compounds, and SOD, GSH and POD activity, soluble protein and sugars increased significantly when the concentration of melatonin treatment was 100 μmol/L. Transcriptomic method was used to analyze the effect of melatonin on the growth of cucumber under salt stress, and to provide a basis for exploring the molecular mechanisms underlying the action of exogenous melatonin in the alleviation of salt stress in cucumber seedlings. These effects may ultimately help improve the salt tolerance of cucumber seedlings, which has broad and far-reaching implications in agricultural production.
Competing interests
The authors declare that they have no competing interests.
Supplemental Material
Download PDF (852.1 KB)Acknowledgments
We would like to thank members of the Professor Zheng and Professor Chen, for his valuable comments and suggestions in the process of writing and revising this manuscript.
Disclosure statement
No potential conflict of interest was reported by the authors.
Data availability statement
The authors confirm that the data supporting the findings of this study are available upon reasonable request. Data and calculation tools are available from the corresponding author (SZ).
Additional information
Funding
References
- Daliakopoulos IN, Tsanis IK, Koutroulis A, et al. The threat of soil salinity: a European scale review. Sci Total Environ. 2016;573:727–739.
- Liu WB, Chen XH, Zhou Y, et al. Effect of salicylic acid on cucumber seed germination and seedling root growth under NaCl stress. Chin Agric Sci Bull. 2013;29(34):166–170.
- Chen SQ, Jiang YT. Causes, harm and control measures of secondary salinization of greenhouse soil. Liaoning Chem Ind. 2018;47(11):1146–1148.
- Kumar A, Singh S, Gaurav AK, et al. Plant growth-promoting bacteria: biological tools for the mitigation of salinity stress in plants. Front Microbiol. 2020;11:1216.
- Etesami H, Beattie GA. Mining halophytes for plant growth-promoting halotolerant bacteria to enhance the salinity tolerance of non-halophytic crops. Front Microbiol. 2018;9:148.
- Yang Y, Guo Y. Elucidating the molecular mechanisms mediating plant salt-stress responses. New Phytol. 2018;217(2):523–539.
- Arya A, Nyamathulla S, Noordin MI, et al. Antioxidant and hypoglycemic activities of leaf extracts of three popular terminalia species. E-J Chem. 2012;9(2):883–892.
- Genisel M, Erdal S, Kizilkaya M, et al. The mitigating effect of cysteine on growth inhibition in salt-stressed barley seeds is related to its own reducing capacity rather than its effects on antioxidant system. Plant Growth Regul. 2015;75(1):187–197.
- Golldack D, Li C, Mohan H, et al. Tolerance to drought and salt stress in plants: Unraveling the signaling networks. Front Plant Sci. 2014;5:151.
- Acquaah G. 2007. Principles of plant genetics and breeding. Oxford: Blackwell.
- Liang W, Cui W, Ma X, et al. Function of wheat Ta-UnP gene in enhancing salt tolerance in transgenic arabidopsis and rice. Biochem Biophys Res Commun. 2014;450(1):794–801.
- Shi H, Chen K, Wei Y, et al. Fundamental issues of melatonin-mediated stress signaling in plants. Front Plant Sci. 2016;7:1124.
- Ren J, Ye J, Yin L, et al. Exogenous melatonin improves salt tolerance by mitigating osmotic, ion, and oxidative stresses in maize seedlings. Agronomy. 2020;10(5):663.
- Li J, Yang Y, Sun K, et al. Exogenous melatonin enhances cold, salt and drought stress tolerance by improving antioxidant defense in tea plant (camellia sinensis (L.) O. Kuntze). Molecules. 2019;24(9):1826.
- Janas KM, Posmyk MM. Melatonin, an underestimated natural substance with great potential for agricultural application. Acta Physiol Plant. 2013;35(12):3285–3292.
- Koodziejczyk I, Posmyk MM. Melatonin–a new plant biostimulator? J Elem. 2016;21(4):1187–1198.
- Zhang HJ, Zhang N, Yang RC, et al. Melatonin promotes seed germination under high salinity by regulating antioxidant systems, ABA and GA4 interaction in cucumber (cucumis sativus L.). J Pineal Res. 2014;57(3):269–279.
- He MW, Wang Y, Wu JQ, et al. Isolation and characterization of S-Adenosylmethionine synthase gene from cucumber and responsive to abiotic stress. Plant Physiol Biochem. 2019;141:431–445.
- Bates L, Waldren R, Teare I. Rapid determination of free proline for water-stress studies. Plant Soil. 1973;39(1):205–207.
- Zhang W, Yuan J, Cheng T, et al. Flowering-mediated root-fungus symbiosis loss is related to jasmonate-dependent root soluble sugar deprivation. Plant Cell Environ. 2019;42(12):3208–3226.
- Jiang M, Zhang J. Effect of abscisic acid on active oxygen species, antioxidative defence system and oxidative damage in leaves of maize seedlings. Plant Cell Physiol. 2001;42(11):1265–1273.
- OuYang Q, Tao N, Zhang M. A damaged oxidative phosphorylation mechanism is involved in the antifungal activity of citral against penicillium digitatum. Front Microbiol. 2018;9:239.
- Amir M, Khan A, Mujeeb M, et al. Phytochemical analysis and in vitro antioxidant activity of zingiber officinale. Free Radical Antioxid. 2011;1(4):75–81.
- Alina VR, Vlad M, Elena MA, et al. The changes of polyphenols, flavonoids, anthocyanins and chlorophyll content in plum peels during growth phases: from fructification to ripening. Notulae Botanicae Horti Agrobotanici Cluj-Napoca. 2017;46(1):148.
- Livak KJ, Schmittgen TD. Analysis of relative gene expression data using real-time quantitative PCR and the 2− ΔΔCT method. Methods. 2001;25(4):402–408.
- Li Q, Li H, Huang W, et al. A chromosome-scale genome assembly of cucumber (cucumis sativus L.). GigaScience. 2019;8(6):giz072.
- Gholami M, Rahemi M, Kholdebarin B. Effect of drought stress induced by polyethylene glycol on seed germination of four wild almond species. Aust J Basic Appl Sci. 2010;4(5):785–791.
- Munns R. Comparative physiology of salt and water stress. Plant Cell Environ. 2002;25(2):239–250.
- Ma N, Hu C, Wan L, et al. Strigolactones improve plant growth, photosynthesis, and alleviate oxidative stress under salinity in rapeseed (brassica napus L.) by regulating gene expression. Front Plant Sci. 2017;8:1671.
- Iqbal M, Ahmad A, Ansari MKA, et al. Improving the phytoextraction capacity of plants to scavenge metal (loid)-contaminated sites. Environ. Rev. 2015;23(1):44–65.
- Taha RS, Seleiman MF, Alotaibi M, et al. Exogenous potassium treatments elevate salt tolerance and performances of Glycine max L. by boosting antioxidant defense system under actual saline field conditions. Agronomy. 2020;10(11):1741.
- Zhang T, Shi Z, Zhang X, et al. Alleviating effects of exogenous melatonin on salt stress in cucumber. Sci Hortic. 2020;262:109070.
- Tan DX, Hardeland R, Manchester LC, et al. Functional roles of melatonin in plants, and perspectives in nutritional and agricultural science. J Exp Bot. 2012;63(2):577–597.
- Arnao MB, Hernández-Ruiz J. Functions of melatonin in plants: a review. J Pineal Res. 2015;59(2):133–150.
- Chen YE, Mao JJ, Sun LQ, et al. Exogenous melatonin enhances salt stress tolerance in maize seedlings by improving antioxidant and photosynthetic capacity. Physiol Plant. 2018;164(3):349–363.
- Jiang C, Cui Q, Feng K, et al. Melatonin improves antioxidant capacity and ion homeostasis and enhances salt tolerance in maize seedlings. Acta Physiol Plant. 2016;38(4):1–9.
- Reiter RJ, Manchester LC, Tan DX. Melatonin in walnuts: influence on levels of melatonin and total antioxidant capacity of blood. Nutrition. 2005;21(9):920–924.
- Wang P, Yin L, Liang D, et al. Delayed senescence of apple leaves by exogenous melatonin treatment: toward regulating the ascorbate-glutathione cycle. J Pineal Res. 2012;53(1):11–20.
- Zhang N, Sun Q, Zhang H, et al. Roles of melatonin in abiotic stress resistance in plants. J Exp Bot. 2015;66(3):647–656.
- Zhang F, Wang Y, Liu C, et al. Trichoderma harzianum mitigates salt stress in cucumber via multiple responses. Ecotoxicol Environ Saf. 2019;170:436–445.
- Abbasi H, Jamil M, Haq A, et al. Salt stress manifestation on plants, mechanism of salt tolerance and potassium role in alleviating it: a review. Zemdirbyste-Agric. 2016;103(2):229–238.
- Sadak MS, Ramadan AAE. Impact of melatonin and tryptophan on water stress tolerance in white lupine (lupinus termis L.). Physiol Mol Biol Plants. 2021;27(3):469–481.
- Keutgen AJ, Pawelzik E. Impacts of NaCl stress on plant growth and mineral nutrient assimilation in two cultivars of strawberry. Environ Exp Bot. 2009;65(2-3):170–176.
- Li ZG, Xu Y, Bai LK, et al. Melatonin enhances thermotolerance of maize seedlings (Zea mays L.) by modulating antioxidant defense, methylglyoxal detoxification, and osmoregulation systems. Protoplasma. 2019;256(2):471–490.
- Kostopoulou Z, Therios I, Roumeliotis E, et al. Melatonin combined with ascorbic acid provides salt adaptation in citrus aurantium L. seedlings. Plant Physiol Biochem. 2015;86:155–165.
- Gao W, Feng Z, Bai Q, et al. Melatonin-mediated regulation of growth and antioxidant capacity in salt-tolerant naked oat under salt stress. Int J Mol Sci. 2019;20(5):1176.
- Li H, Chang J, Chen H, et al. Exogenous melatonin confers salt stress tolerance to watermelon by improving photosynthesis and redox homeostasis. Front Plant Sci. 2017;8:295.
- Shi H, Jiang C, Ye T, et al. Comparative physiological, metabolomic, and transcriptomic analyses reveal mechanisms of improved abiotic stress resistance in bermudagrass [cynodon dactylon (L). pers.] by exogenous melatonin. J Exp Bot. 2015;66(3):681–694.
- Weeda S, Zhang N, Zhao X, et al. Arabidopsis transcriptome analysis reveals key roles of melatonin in plant defense systems. PLoS One. 2014;9(3):e93462.