Abstract
Immunosenescence is driven by repeated and continuous immune activation. Sensitive markers are warranted to detect and monitor the process of premature immune ageing. We analysed the β-Klotho expression on T-cell subsets from healthy donors: young adults, n = 12 (20–30 y); middle-aged, n = 62 (30–50 y) and elderly, n = 18 (>70 y). The absolute counts (AC) and percentages of total, CD4+, CD8+ and CD8 + CD27-CD57+ T-cells, CD4/CD8 ratio, and the share of β-Klotho + T-cell subsets were analysed by multicolour flow cytometry. AC of T-cell subsets showed non-significant differences among the groups. The percentages of β-Klotho(+) total, CD4+ and CD8+ T-cells in young adults exceeded significantly the expression in the elderly and middle-aged subjects: (mean) 2.13 vs. 0.48; 3.23 vs. 0.48; 6.87 vs. 2.28, and 0.75 vs. 2.21; 0.93 vs. 3.32; 6.62 vs. 6.62, respectively (p < 0.05 all). The β-Klotho expression on total, CD4+ and CD8+ T-cells among middle-aged participants was heterogeneous and significantly higher from the elderly only in CD8 + T-cells (mean): 0.75 vs. 0.47 (p = 0.1); 0.93 vs. 0.72 (p = 1.0) and 3.62 vs 1.75 (p = 0.01), respectively. The proportion of β-Klotho(+) CD8+ cells did not correlate with age, but correlated inversely and significantly with the share of CD27-CD57 + CD8+ T-cells in middle-aged subjects (R = −0.4, p < 0.01). We hypothesise that CD8 + T-cells showed higher sensitivity to the effects of immune-senescence, at least in part, due to the higher expression and dependence on the effects of KLOTHO gene products. We propose that a reduced share of β-Klotho + CD8+ T-cells indicates exhaustion due to immune activation, and may serve as an early marker of premature immunosenescence.
Keywords:
Introduction
The pathology of old age is closely related to the physiological phenomenon of immunosenescence. Immunological ageing is characterised by quantitative and qualitative changes affecting mainly the adaptive immune response: decreased production of T and B lymphocytes with limited potential for proliferation and differentiation, narrowed repertoire of naive lymphocytes, increased production of proinflammatory cytokines at the expense of regulatory cytokines, elevated levels of activation and increased susceptibility of lymphocytes to apoptosis [Citation1]. In fact, the ageing immune system loses the ability both to neutralise real threats such as pathogens and tumor-modified antigens, and to limit and regulate immune inflammation. Age-related T-lymphocyte dysfunction is key to the onset of immunological ageing [Citation2, Citation3] and is causally related to the increased incidence of neoplasms, infections with complications and chronicity [Citation4, Citation5], autoimmune conditions, generalised inflammation, atherosclerosis [Citation6] and overall mortality [Citation7] in advanced age. A number of contemporary environmental and life-style factors, such as stress, pollution, unhealthy diet, excessive antibiotic use and immunostimulating drugs, directly affect the processes of cellular ageing and their repair, leading to premature immunosenescence. Therefore, a lot of recent studies have focused on the mechanisms and biomarkers of this phenomenon.
A lot of data has been accumulated on the association between one particular gene, KLOTHO, and the process of ageing. KLOTHO overexpression increased the life expectancy of mice by 20–30% [Citation8], while experimental animals with KLOTHO deficiency showed signs of ageing and premature death [Citation9]. KLOTHO encodes a family of related proteins including α-, β- and γ-Klotho isoforms with pleiotropic neuro-endocrine-immunological effects [Citation10–12]. β-Klotho forms complexes with key Fibroblast growth factor (FGF) receptors. By selectively increasing affinity for FGF23, KLOTHO regulates phosphate and calcium homeostasis; β-Klotho is an obligate co-receptor for FGF21, a hormone with a central role in carbohydrate and lipid homeostasis, preventing obesity and diabetes. The secretory form of Klotho regulates the effects of molecules engaged in the process of inflammation, such as insulin, insulin-like growth factor 1 (IGF-1), transforming growth factor beta (TGF-β) and interferon gamma (IFN-γ). Intracellular Klotho blocks the multimerisation of retinoic-acid-inducible gene-I (RIG-I), a protein associated with increased expression of proinflammatory cytokines (interleukins IL-6 and IL-8) and oxygen radicals [Citation13, Citation14].
β-Klotho is expressed mainly in the liver, pancreas and adipose tissues [Citation15]. While Nakai et al. [Citation16] demonstrated expression of β-Klotho in macrophages, Jackson et al. [Citation17] found β-Klotho among different central nervous system cell types; however, the expression and effects of Klotho on cells of the immune system have not been clarified yet. In this study, we investigated the expression levels of β-Klotho on peripheral blood CD4 and CD8 T lymphocytes from healthy subjects and its association with signs of premature ageing. To this end, we analysed the expression of β-Klotho in young, middle-aged and elderly healthy volunteers.
Subjects and methods
Ethics statement
Informed consent was obtained from all participants and the study was approved by the Ethical Committee of the National Centre of Infectious and Parasitic Diseases, Sofia, Bulgaria (Institutional Review Board/Institutional Ethics Committee (IRB/IEC) number: IRB 00006384), protocol No 5/2020.
Study populations
Whole peripheral blood samples were obtained from 92 healthy subjects (41 male and 51 female) during routine prophylactic examinations at Acibadem City Clinic Tokuda, Sofia, Bulgaria. Exclusion criteria were as follows: history of chronic disease (including diabetes, impaired renal or hepatic function), hospitalisation in the last 2 months preceding the study, administration of immunostimulatory or immunosuppressive drugs, abnormal complete blood count and/or biochemical status, increased C-reactive protein, chronic HIV infection, recurrent HSV, EBV or CMV infection, a positive screening test for the presence of anti-nuclear antibodies, body mass index > 30, alcohol addiction and other dependencies. According to age, the following groups were defined: A: young adults, n = 12 (6 male and 6 female) 20 - 30 y (mean, ±SD: 26.8 ± 3.01); B: middle-aged adults, n = 62 (28 male and 34 female), 30 - 50 y (mean, ±SD: 41.73 ± 6.66); C: elderly, n = 18 (7 male and 11 female), aged > 70 y, mean 76.13 ± 5.7. The rationale behind this grouping was as follows. The groups of young (<30 y) and elderly subjects (>70 y) served as ‘models’ of the young and the ageing immune system. We chose to define the age range of the middle-aged group (30–50 y) with a significant gap from the elderly (>70) group in order to avoid a possible overlap between pathological and physiological ageing.
Multicolour flow cytometry
The absolute counts (AC) of lymphocytes (CD45+), the share and AC of T-cells (CD3+), helper T-cells (CD4+), cytotoxic T-cells (CD8+) lymphocyte subsets, CD4/CD8 ratio, CD8 + CD27-CD57+ subpopulation and the share of lymphocytes expressing β-Klotho in each subset were evaluated using a combination of anti-CD45-PerCP, anti-CD3-FITC; anti-CD8-PE, anti-CD4-APC and anti- βKlotho/Alexa Fluor 700, TRUCount tubes (BD Biosciences) and a standard lysis-no-wash procedure. Flow cytometry analysis was performed on a BD FACSCanto™ II system with FACSDiva 6.1.3. software (BD Biosciences). An FMO sample without β-Klotho antibody staining was included as autofluorescence control.
Data analysis
Data are presented as mean values with standard deviation (±SD). Comparisons between groups were evaluated statistically using non-parametric Kruskal–Wallis ANOVA rank tests, followed by post-hoc group pairwise comparisons, with p-value adjustment for multiple comparisons using Dunn’s test. Correlations were evaluated with Spearman’s rank correlation test. Differences were considered statistically significant at the level of p < 0.05 and rho > 0.3, at CI 0.95. Analyses were performed using IBM SPSS v21.
Results
Absolute counts and percentages of CD3+, CD3 + CD4+ and CD3 + CD8+ lymphocytes in the studied groups
The absolute counts of total T-cells and CD8 + T but not of CD4+ T-lymphocytes decreased with age, although the differences between the groups were statistically non-significant. No significant differences were established between the percentages of the populations or CD4/CD8 ratios, either ().
Table 1. Absolute counts and proportions of lymphocytes and lymphocytes subset in the studied groups (mean ± SD).
Our results showed clear differences in the expression of β-Klotho between the studied groups. The highest percentages of β-Klotho (+) total, CD4+ as well as CD8+ T cells were found among the youngest participants (group A) and they exceeded significantly the expression observed in the elderly (group C): (mean ± SD) 2.21 ± 1.51 vs. 0.47 ± 0.64 (p = 0.0001); 3.32 ± 2.61 vs. 0.72 ± 0.85 (p = 0.003); 6.62 ± 3.92 vs. 1.57 ± 2.03 (p = 0.0001), respectively (, ). There was a significant difference in the expression of β-Klotho on CD8+ T-cells between the middle-aged (group B) and the elderly subjects (group C): (mean ± SD) 3.62 ± 2.67 vs.1.57 ± 2.03 (p = 0.016), respectively (, ). Such a difference was not observed for CD4+ T-cells (mean ± SD) 0.93 ± 1.70 vs. 0.72 ± 0.85 (p = 1.0). In addition, in all tested subjects, the expression of β-Klotho was more abundant on CD8+ as compared to CD4 + T-cells. Noteworthy, the β-Klotho expression on CD4+ and CD8+ T-cells among middle-aged participants (group B) was quite heterogeneous, especially on CD8 T-cells. Interestingly, in 29/62 (46.7%) of group B participants, the expression of β-Klotho on CD8 T-lymphocytes was lower than the minimal expression level observed in group A (3.20) and did not differ significantly from that in the elderly group (mean 1.59 vs. mean 1.42, p > 0.05). At the same time, there was no correlation between the age and percentage of β-Klotho + CD8+ T-cells (p > 0.05, data not shown). Therefore, we reasoned that the heterogeneity of β-Klotho expression was rather associated with a premature senescence of CD8+ T-cells. To this end, we analysed the proportion of CD8+ CD27-CD57+ T-cells which have reduced proliferative potential, increased cytotoxic activity and propensity for apoptosis and their increased proportion is a marker of exhausted T cell pool [Citation18, Citation19]. A significant inverse correlation () between β-Klotho (+) CD8 + T cells and the percentage of CD8+ CD27-CD57+ T-lymphocytes in the middle-aged adults (group B; 30–50 y) (rho= −0.4, p < 0.01) was established.
Figure 1. Expression of β-Klotho on total CD3+ (A, circles), CD4+ (B, triangles) and CD8+ (C, diamonds) T-cells in healthy subjects. Group A: young adults (open symbols); group B: middle-aged adults (light grey symbols) and group C: elderly (dark grey symbols); ***p < 0.001; **p < 0.01; *p < 0.05; ns, not significant, p > 0.05. Means ± SD are denoted. Comparisons between groups were done by using non-parametric Kruskal–Wallis ANOVA rank tests, followed by post-hoc group pairwise comparisons, with p-value adjustment for multiple comparisons using Dunn’s test.
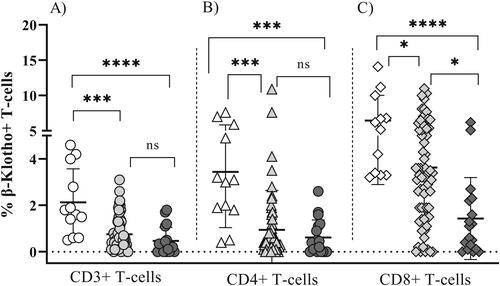
Discussion
Immunosenescence is a multifactorial phenomenon that affects all compartments of the immune system [Citation20]. The main driving forces of immunosenescence, which is not necessarily related to age, are repeated and continuous immune activation and chronic inflammation. We studied the expression of β-Klotho on T-cells from donors with no clinical data or history of pathological condition, and observed significant differences not only between age-defined groups but within the group of middle-aged adults.
Age-associated changes in T-lymphocytes are well documented, comprising accumulation of memory cells at the expense of naïve T-cells [Citation21], decreased expression of co-stimulating molecules (CD28, CD27, CD40L), expression of apoptosis-inducing and inhibitory receptors (Programmed cell death protein 1 - PD1, cytotoxic T lymphocyte–associated antigen 4 - CTLA-4, Lymphocyte activation gene-3 - LAG-3, CD160, T-cell immunoreceptor with Ig and ITIM domains - TIGIT) and markers of replicative exhaustion (CD57) [Citation7].
There is a large amount of accumulated data on the association of Klotho with the premature ageing and inflammation-related pathology [Citation12, Citation22], chronic kidney disease [Citation23, Citation24] and cardiovascular disease [Citation9, Citation25, Citation26]. A limited number of studies have looked at the effects of Klotho on immune functions. Okada et al. demonstrated thymic atrophy, reduced number of splenocytes and severe B-lymphopenia in adult kl/kl mice [Citation22]. According to Witkowski et al. the expression of KLOTHO in CD4+ lymphocytes of healthy elderly donors and patients with rheumatoid arthritis was downregulated at the mRNA and protein levels and this was associated with lower expression of CD28 and increased TNF-α expression [Citation27]. Similar results were reported for patients with inflammatory bowel disease [Citation28]. Klotho was shown to reduce inflammation through inhibition of proinflammatory cytokine expression (IL-6, IL-8 and TNF-α) and by increasing the secretion of inhibitory IL-10 [Citation24]. Since Klotho downregulates NF-kB pathway activity and upregulates IL-10 production, it possesses anti-inflammatory characteristics through suppression of proinflammatory cytokine expression.
The relationship between Klotho and the process of premature ageing of the immune system is not fully clarified. To our knowledge, this is the first study to examine β-Klotho expression on total, CD4+ and CD8+ T-cells in healthy adults. The most abundant and heterogeneous expression of β-Klotho was observed on CD8+ T-cells, with no correlation to age. It is well established that the increased share of CD8+ T-cell pool and, hence, decreased CD4/CD8 ratio may reflect low-level immune activation in the settings of chronic viral infection or chronic inflammation [Citation29, Citation30].
A major limitation of this study is the small number of tested individuals in the groups of young adults and elderly. Therefore, these observations need to be confirmed by increasing the number of donors in the age-defined groups and quantification of KLOTHO gene expression in lymphocytes via reverse transcription polymerase chain reaction (RT-PCR).
Conclusions
The expression level of Klotho on CD4 and CD8 T-cells tends to decrease with ageing. CD8 T-lymphocytes seem to be more sensitive to the effects of immune senescence, and in part, this could be due to the higher expression and dependence on the effects of KLOTHO gene products. This hypothesis is supported by the inverse correlation between the proportion of β-Klotho+ CD8+ T-lymphocytes and CD8+ T-cells expressing CD57 marker of replicative senescence.
Authors’ contributions
Conceptualisation, Y.T. and R.E.; conducted the experiments Y.T, R.E and I.I; writing—original draft preparation, Y.T and R.E.; writing—review and editing, Y.T. and M.N.; visualisation, Y.T.; collection of biological material, V.K.; writing—review and editing Y.T., I.I, I.S and R.E; supervision, M.N. All authors have read and agreed to the published version of the manuscript.
Data availability statement
The data that support the findings of this study are available from the corresponding author (YT), upon reasonable request.
Additional information
Funding
References
- Aiello A, Farzaneh F, Candore G, et al. Immunosenescence and its hallmarks: how to oppose aging strategically? A review of potential options for therapeutic intervention. Front Immunol. 2019;10:339. PMID: 31608061; PMCID: PMC6773825.
- Accardi G, Caruso C. Immune-inflammatory responses in the elderly: an update. Immun Ageing. 2018;15:11. PMID: 29507595; PMCID: PMC5833087.
- Song Y, Wang B, Song R, et al. T-cell immunoglobulin and ITIM domain contributes to CD8+ T-cell immunosenescence. Ageing Cell. 2018;17(2):e12716. Epub 2018 Jan 19. PMID: 29349889; PMCID: PMC5847879.
- Pawelec G, Ferguson FG, Wikby A. The SENIEUR protocol after 16 years. Mech Ageing Dev. 2001;122(2):132–344. PMID: 11166351
- Strindhall J, Skog M, Ernerudh J, et al. The inverted CD4/CD8 ratio and associated parameters in 66-year-old individuals: the swedish HEXA immune study. Age (Dordr). 2013;35(3):985–991. Epub 2012 Mar 14. PMID: 22415616; PMCID: PMC3636392.
- Schurich A, Henson SM. The many unknowns concerning the bioenergetics of exhaustion and senescence during chronic viral infection. Front Immunol. 2014;5:468. PMID: 25309548; PMCID: PMC4174864.
- Weiskopf D, Weinberger B, Grubeck-Loebenstein B. The aging of the immune system. Transpl Int. 2009;22(11):1041–1050. Epub 2009 Jul 16. PMID: 19624493.
- Kuro-O M, Matsumura Y, Aizawa H, et al. Mutation of the mouse klotho gene leads to a syndrome resembling ageing. Nature. 1997;390(6655):45–51. PMID: 9363890.
- Xu Y, Sun Z. Molecular basis of klotho: from gene to function in aging. Endocr Rev. 2015;36(2):174–193. Epub 2015 Feb 19. PMID: 25695404; PMCID: PMC4399270
- Hu MC, Shiizaki K, Kuro-O M, et al. Fibroblast growth factor 23 and klotho: physiology and pathophysiology of an endocrine network of mineral metabolism. Annu Rev Physiol. 2013;75:503–533.
- Kuro-O M, Moe OW. FGF23-αKlotho as a paradigm for a kidney-bone network. Bone. 2017;100:4–18. Epub 2016 Nov 12. PMID: 27847255.
- Kuro-O M. The klotho proteins in health and disease. Nat Rev Nephrol. 2019;15(1):27–44. PMID: 30455427.
- BonDurant LD, Potthoff MJ. Fibroblast growth factor 21: a versatile regulator of metabolic homeostasis. Annu Rev Nutr. 2018;38:173–196. Epub 2018 May 4. PMID: 29727594; PMCID: PMC6964258.
- Zhou HJ, Li H, Shi MQ, et al. Protective effect of klotho against ischemic brain injury is associated with inhibition of RIG-I/NF-κB signaling. Front Pharmacol. 2017;8:950. PMID: 29403373; PMCID: PMC5778393
- Kuro-o M. Endocrine FGFs and klothos: emerging concepts. Trends Endocrinol Metab. 2008;19:239–45. Wu X, Ge H, Gupte J, et al. Co-receptor requirements for fibroblast growth factor-19 signaling. J Biol Chem. 2007;282:29069–72
- Nakai K, Haba R, Kushida Y, et al. Macrophages express βKlotho in skin lesions of psoriatic patients and the skin of imiquimod-treated mice. J Dermatol. 2018;45(12):1475–1477. Epub 2018 Oct 10. PMID: 30302794.
- Jackson TC, Janesko-Feldman K, Carlson SW, et al. Robust RBM3 and β-klotho expression in developing neurons in the human brain. J Cereb Blood Flow Metab. 2019;39(12):2355–2367.
- Brenchley JM, Karandikar NJ, Betts MR, et al. Expression of CD57 defines replicative senescence and antigen-induced apoptotic death of CD8+ T cells. Blood. 2003;101(7):2711–2720. Epub 2002 Nov 14. PMID: 12433688
- Kasakovski D, Xu L, Li Y. T cell senescence and CAR-T cell exhaustion in hematological malignancies. J Hematol Oncol. 2018;11(1):91. PMID: 29973238; PMCID: PMC6032767.
- Lu X, Yang YM, Lu YQ. Immunosenescence: a critical factor associated With organ injury After sepsis. Front Immunol. 2022;13:917293. PMID: 35924237; PMCID: PMC9339684.
- Wherry EJ, Kurachi M. Molecular and cellular insights into T cell exhaustion. Nat Rev Immunol. 2015;15(8):486–499. PMID: 26205583; PMCID: PMC4889009.
- Okada S, Yoshida T, Hong Z, et al. Impairment of B lymphopoiesis in precocious ageing (klotho) mice. Int Immunol. 2000;12(6):861–871. PMID: 10837414
- Rodelo-Haad C, Santamaria R, Muñoz-Castañeda JR, et al. FGF23, biomarker or target? Toxins. 2019;11(3):175.
- Typiak M, Piwkowska A. Antiinflammatory actions of klotho: implications for therapy of diabetic nephropathy. IJMS. 2021;22(2):956. PMID: 33478014; PMCID: PMC7835923.
- Zhu L, Stein LR, Kim D, et al. Klotho controls the brain-immune system interface in the choroid plexus. Proc Natl Acad Sci U S A. 2018;115(48):E11388–E11396. Epub 2018 Nov 9. PMID: 30413620; PMCID: PMC6275534.
- Chen K, Wang S, Sun QW, et al. Klotho deficiency causes heart via impairing the Nrf2-GR pathway. Circ Res. 2021;128(4):492–507. Epub 2020 Dec 18. PMID: 33334122; PMCID: PMC8782577.
- Witkowski JM, Soroczyńska-Cybula M, Bryl E, et al. Klotho–a common link in physiological and rheumatoid arthritis-related ageing of human CD4+ lymphocytes. J Immunol. 2007;178(2):771–777. PMID: 17202338
- Thurston RD, Larmonier CB, Majewski PM, et al. Tumor necrosis factor and interferon-gamma down-regulate klotho in mice with colitis. Gastroenterology. 2010;138(4):1384–1394. 1394.e1-2. Epub 2009 Dec 11. PMID: 20004202; PMCID: PMC3454518.
- Serrano-Villar S, Pérez-Elías MJ, Dronda F, et al. Increased risk of serious non-AIDS-related events in HIV-infected subjects on antiretroviral therapy associated with a low CD4/CD8 ratio. PLoS One. 2014;9(1):e85798. PMID: 24497929; PMCID: PMC3907380
- Cao W, Mehraj V, Kaufmann DE, et al. Elevation and persistence of CD8 T-cells in HIV infection: the achilles heel in the ART era. J Int AIDS Soc. 2016;19(1):20697. PMID: 26945343; PMCID: PMC4779330.