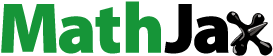
Abstract
Astragalus glycyphyllos L. is widely used in Bulgarian folk medicine. Extracts from this plant have antiproliferative, antitumor and immune stimulating effects in vitro and in vivo. The aim of this study was to examine the potential antiviral activity of a standardized extract from the aerial parts of the plant and to formulate it in a suitable dosage form. A dry extract was obtained, and its phytochemical composition was determined by ultra-high-performance liquid chromatography–high resolution electrospray ionization mass spectrometry (UHPLC-HRESIMS). The extract contained flavonoids and predominantly saponins. 17(R),20(R)-3β,6α,16β-trihydroxycycloartanyl-23-carboxylic acid 16-lactone 3-O-β-D-glucopyranoside was used as a reference for quantitation. The extract had 3% saponins. The antiviral activity of the extract was tested against Simplexvirus humanalpha type 1 (acyclovir sensitive) and 2 (acyclovir resistant) in vitro. Between 60% and 70% of protection was reached against both virus strains when the extract (at the maximal non-toxic concentration of 0.6 mg/mL) was added simultaneously with and 1 h after Madine and Darby bovine kidney cell inoculation. The extract was also tested in combination with acyclovir only against Simplexvirus humanalpha type 1, in vitro. Further, the lyophilized extract was mixed with appropriate excipients aiming to prepare tablets via direct compaction. The optimized tablets fulfilled the requirements of the pharmacopoeia for disintegration and friability. Thus, the study demonstrated the possibility to formulate a standardized extract of A. glycyphyllos in tablets and their potential for adjuvant antiviral application in further trials.
Introduction
Medicines used in medical practice to treat viral diseases are quite limited. This circumstance, together with the ever-increasing resistance to first-line drugs, calls for the development of new antiviral agents. A good approach that receives growing attention is the research of substances of plant origin and plant extracts for alternative treatment. Some plant extracts and substances have a wide spectrum of antiviral activity against a range of agents that cause respiratory to sexually transmitted infections. Astragalus glycyphyllos L. (Fabaceae) is an herbaceous perennial plant, commonly distributed on rocky soils in meadows near forests and amongst bushes, in mountainous regions of Bulgaria, to 1800 m a. s. l. [Citation1]. In folk medicine, an infusion of the aerial parts of the species is applied as a spasmolytic, diuretic, tonic and anti-inflammatory agent. It is used in the treatment of kidney stones, hypertension, arrhythmia, cardiac failure, cancer, dermatitis, constipation, rheumatism, nervous and gastrointestinal diseases, and to stimulate labor. Extracts from this plant exhibit antibacterial, anti-trichomonas and anti-mycotic effects [Citation2]. Phytochemical investigation of the species revealed that it contains flavonoids and triterpenoid saponins [Citation3]. Several flavones and flavonols and their glycosides have been identified in the plant [Citation4]. The species has predominately cycloartane saponins (askendozides C and F, 17(R),20(R)-3β,6α,16β-trihydroxycycloartanyl-23-carboxylic acid 16-lactone 3-O-β-D-glucopyranoside), alongside a small proportion of oleanane-type triterpenoids (soyasapogenol B and 3β,22β,24-trihidroxyolean-12-en-19-one) [Citation5,Citation6]. The pharmacological action of A. glycyphyllos is largely due to its saponin content. However, there are no reports on quantitation and standardization of an extract from this species in terms of triterpenoid saponins.
A defatted extract from the aerial parts of A. glycyphyllos showed hepatoprotective and antioxidant activities in vivo [Citation7]. A saponin mixture from the herb had antiproliferative and cytotoxic effects in vitro/in vivo on the Graffi myeloid tumor [Citation8]. The epoxycycloartane saponin displayed hMAO-B inhibition, neuroprotection on isolated rat brain synaptosomes [Citation5], as well as pronounced immunostimulating effect [Citation6].
There are many in vitro/in vivo studies on the biological activities of Astragalus species, but investigations of the antiviral activities are limited [Citation9]. For instance, Astragali radix showed curative effects on mice infected with Simplexvirus humanalpha type 1 (SvHA1) and protective effects against Japanese Encephalitis Virus infection in mice [Citation10]. The saponin astragaloside II exhibited a 100% protective effect on T-lymphocytes against HIV [Citation11]. Ethanol extract of Astragalus vulneraria possessed inhibitory effects on the multiplication of human herpesvirus 1 and poliovirus 2 in cell culture [Citation11]. Supplementation with Astragalus polysaccharides from Astragalus mongholicus can inhibit the replication of several animal viruses, including H9N2 avian influenza virus, foot and mouth disease virus, Newcastle disease virus and infectious bursal disease virus [Citation12]. To date, there are no reports on the antiviral effects of A. glycyphyllos.
Formulation of oral dosage forms containing extract of A. glycyphyllos is considered appropriate, taking into consideration the traditional oral route of administration of various aqueous extracts from the plant. Among oral formulations, tablets are the most preferred dosage forms. They are more stable and convenient for self-administration than liquid dosage forms. In addition, they could protect to a higher degree the active substance from different environmental factors in vitro (humidity, light) or in vivo (enzymes). Moreover, formulation of a dosage form containing a quantified extract from the species has not been previously done.
The aim of the present study was to prepare a dry standardized extract from the aerial parts of A. glycyphyllos, to evaluate its potential as an antiviral agent in vitro (alone and in combination with acyclovir (ACV) – the most used drug of choice against replication of SvHA1 and SvHA2) and to formulate an oral tablet dosage form that contains it.
Materials and methods
Plant material
The aerial parts of A. glycyphyllos were collected during the flowering stage in June 2021, from Vitosha Mountain (42°36′56.1″N 23°13′39.9″E), Sofia region, Bulgaria. The species was identified by Dr. Dolja Pavlova (Faculty of Biology, Sofia University), where a voucher specimen was deposited (SO-107613). The plant material was dried, pulverized and sieved (3 mm).
Preparation of extract
The material (200 g) was placed in a filter paper drum and then extracted with 50% MeOH in a semi-industrial scale Soxhlet-type extractor (3 L) for 22 h. The resulting extract (2 L) was concentrated and lyophilized for 96 h at −110 °C and 0.150 mbar of vacuum. The dried extract was named DEAG and used for the experiments.
Phytochemical analysis and standardization of DEAG
The qualitative analysis of flavonoids and saponins in DEAG was performed as reported previously [Citation13]. Quantitation of saponins in the extract was done by a known method [Citation14]. Both methods used ultra-high-performance liquid chromatography – high resolution electrospray ionization mass spectrometry (UHPLC-HRESIMS). The system consisted of an UHPLC (Dionex UltiMate 3000 RSLC, ThermoFisher Scientific, Bremen, Germany) connected to a Q Exactive Plus Orbitrap mass spectrometer with a heated electrospray ionization ion source (ThermoFisher Scientific). The operating conditions of the mass spectrometer were as reported before [Citation15]. The UHPLC conditions for the qualitative and the quantitative analyses are described previously [Citation13,Citation14].
For both analyses the sample was prepared by dissolving 200 mg of DEAG in MeOH and diluting with the same solvent in a 10.0-mL volumetric flask. Two-microliter aliquots were injected in the UHPLC system after filtration (Polyvinylidene difluoride [PVDF], 0.22 μm).
To identify compounds in DEAG, detection was performed in the (−) mode. Retention time, as well as a m/z filter, adjusted to the corresponding deprotonated molecule were used as criteria. MS/MS experiments supported the identification (by the aglycone and the sugar moiety loss) as reported [Citation13].
The reference used for the quantitation was 17(R),20(R)-3β,6α,16β-trihydroxycycloartanyl-23-carboxylic acid 16-lactone 3-O-β-D-glucopyranoside (epoxycycloartane saponin, ECAS, Supplemental Figure S1). The saponin was isolated with purity 99.7% and structurally elucidated by nuclear magnetic resonance (NMR) and HRESIMS [Citation5]. The HRESIMS spectrum of the compound showed an adduct [M + HCOO]− at m/z 623.3441 (calcd. 623.3432), which is stable and suitable as an ion marker to perform quantitation. Standard solutions of the reference saponin were prepared in MeOH [Citation14]. One microliter of each solution was injected into the UHPLC-MS system in triplicate to obtain the calibration curve.
Thermo Scientific Xcalibur®, Version 4.2 was used for data collection, processing, construction of the calibration curve and calculation of the results.
Cytotoxicity assay
Cell toxicity was monitored by determining the effect of the extract and ACV on cell viability (combined cytotoxicity). Cell viability was determined by a colorimetric method (MTT assay) [Citation16]. Confluent monolayers (100% confluence) of Madine and Darby bovine kidney (MDBK) cells in 96-well plates were overlaid with 0.1 mL/well maintenance medium, 0.1 mL/well of serial two-fold dilutions of the extract and ACV (in maintenance medium) or 0.1 mL/well only maintenance medium (in cell controls) and were incubated at 37 °C for 72 h. On day 3, 0.02 mL of MTT (5 mg/mL in phosphate buffered saline [PBS]) was added to each well and the plates were incubated for 2 h at 37 °C. The optical densities (OD) were determined via a plate reader at λ = 540 nm. The percentage of viable treated cells was calculated by the following formula [(ODexp.)/(ODcell control)] × 100, where (ODexp.) and (ODcell control) indicate the absorbance values of the test sample and the cell control, resp. The 50% cytotoxicity concentration (CC50) was calculated by regression analysis of the dose–response curves. The maximal non-toxic concentration (MNC) was defined as the highest concentration at which the calculated value for cell viability equals 100%.
Antiviral activity of DEAG
MDBK cells were obtained from the National Bank for Industrial Microorganisms and Cell Cultures, Sofia, Bulgaria (cat. No. 1031). They were grown in low glucose Dulbecco’s Modified Eagle Medium supplemented with 8% fetal calf serum (FCS). The maintenance medium contained FCS with reduced concentration of 4%. The ACV-sensitive F strain of SvHA1 and the ACV-resistant DD strain of SvHA2 were obtained from the National Center of Infectious and Parasitic Diseases (Sofia, Bulgaria). The viruses were propagated in MDBK cells and stored at −70 °C until used. The virus titer was determined by cytopathic effect (CPE) assay using the method of Reed and Muench [Citation17] and plaque assay [Citation18] in MDBK.
MTT-based colorimetric assay for detection of SvHA replication inhibition
A modification of the MTT assay developed for the screening of anti-SvHA compounds was used [Citation19,Citation20]. Two experimental designs were used in which the extract and ACV (used as a reference substance) were added at different points of time. A confluent monolayer in 96-well plates was plated with 0.1 mL/well of a 100 TCID50 (50% Tissue Culture Infectious Dose) virus suspension for both virus strains. TCID50 was used as a standard unit for endpoint dilution assays and similar methods in both cell cultures and tissue cultures. In the first experimental setup, 0.1 mL of serial two-fold dilutions of the extract or ACV (minimum three wells/dilution) or 0.1 mL of maintenance medium were added to the wells that served as viral controls immediately after virus inoculation. In the second experimental setup, the plates were incubated for 1 h at 37 °C for virus adsorption, and then dilutions of the extracts or ACV or maintenance medium (for the virus control) were added. Untreated wells were used as cell controls (where only 0.2 mL/well of support medium was added); this was designated as ‘mock infected control’. The plates were incubated at 37 °C for 120 h. On day 5, 20 μL of MTT (5 mg/mL in PBS) was added to each well and the plates were incubated for 1.5 h at 37 °C. The MTT medium was removed, and the resulting formazan precipitate was dissolved in dimethyl-sulfoxide (DMSO, 99.5% chromatography grade, Labscan). The optical density values were determined at λ = 540 nm. Cell protection (%) was calculated using the following formula:
where (ODT)HuAHV is the absorbance of the test sample, (ODC)HuAHV is the absorbance of the virus-infected control (no compound) and (ODC)MOCK is the mock-infected control.
The concentration of DEAG or ACV that effectively allowed 50% of the cells to maintain their effective survival (EC50) was calculated by linear regression analysis of the dose–response curves.
Effect of the extract on viral infectivity (virucidal activity)
The direct virus inactivating effect [Citation20] of DEAG (against both virus strains used) was tested by a direct contact assay. Undiluted stock virus suspensions were treated with equal volumes of DEAG in MNC, prepared in maintenance medium and incubated at 37 °C for 5, 15, 30, 60, 120 and 240 min. DEAG-free maintenance medium was used as viral control. At the end of each time interval, the control and the treated viruses were frozen. The infectious virus titers were determined via the plaque-forming assay in MDBK cells [Citation18].
Combined antiviral effect
The combined antiviral effect of DEAG and ACV was tested only against the F strain of SvHA type 1 (DD strain of SvHA type 2 is ACV-resistant). The concentrations used in the evaluation of the combined antiviral effects were significantly lower than MNC. Based on the combined cytotoxicity assay, in EC50 concentrations and below there was no detectable cytotoxicity. The following concentrations of each partner in the dual combinations were used: EC50, EC50/2, EC50/4, EC50/8, EC50/16. A complete MDBK cell monolayer in 96-well plates (three plates/experiment) was overlaid with 0.1 mL/well of virus suspension (low multiplicity of infection 100 TCID50/well). After 1 h of incubation at 37 °C (for virus to adsorb) the virus suspension was removed. Then based on a checkerboard design of the experiments, 0.1 mL of the corresponding dilution of DEAG and ACV was added. In wells that served for virus and cell control, 0.2 mL of maintenance medium was added. Plates were incubated at 37 °C for 120 h. On day 5, 20 μL of MTT (5 mg/mL in PBS) was added to each well and the monolayers were incubated for 1.5 h at 37 °C. The medium with MTT was removed and the resulting formazan precipitate was dissolved in DMSO. The extinction values were determined at λ = 540 nm.
Evaluation of the type of the combined effects was done according to the three-dimensional model of Prichard and Shipman [Citation21] using the MacSynergyTM II software [Citation22]. The dose–response curves for each drug tested individually were used for calculation of the theoretical additive interactions. The calculated theoretical additive surface was subtracted from the experimentally obtained dose–response surface. The 95% confidence interval around the experimental dose–response surface was used for statistical analysis of the data. Synergy was defined in the case of peaks above the horizontal plane at 0% inhibition. Antagonism was defined in the case of depression in the plane. The following criteria were applied to volumes of synergy or antagonism expressed as mg/mL2%: <25: insignificant; 25–50: minor but significant; 50–100: moderate; >100: strong synergy or antagonism [Citation23].
Data analysis
Data from antiviral activity assays are presented as mean values with standard deviation from triplicate experiments. The analysis of the combined antiviral effects was done via the MacSynergyTM II software. Data from all other experiments were processed using the Origin 9.1TM software.
Preparation and characterization of tablets
Microcrystalline cellulose (MCC; Vivapur 102) was purchased by JRC Pharma (Rosenberg, Germany) and lactose monohydrate was obtained from Meggle (Wasserburg, Germany). Talc and magnesium stearate were from Sigma Aldrich (Taufkirchen, Germany). Tablets were prepared by direct compaction following the main stages, in particular sieving of substances, dry mixing in a mixer and direct compression. The compaction was conducted using a single punch tablet press (Korsch, Erweka-Heusenstamm, Germany) with a 10-mm diameter of the punch. The tablets were characterized via tests for uniformity of mass, disintegration, resistance to crushing (Erweka TBH 30, Heusenstamm, Germany) and friability (Pharma test PTF E, Germany). An in vitro release study was carried out according to the USP paddle method (apparatus II) with a stirring rate of 50 rpm and purified water (180 mL) as dissolution medium (Erweka DT 600, Heusenstamm, Germany). Samples were withdrawn at certain time intervals (15, 30, 45, 60 and 75 min) and the dissolution volume was maintained by replacement with fresh medium.
Quantitative determination of saponins in the tablets
The dissolution media from each time interval was taken. Each sample was filtered through a membrane syringe filter (PVDF, 0.45 µm), and an aliquot (1 mL) was dried on a rotary evaporator. The residue was sonicated (35 kHz) for 15 min with 1 mL MeOH, centrifuged at 15400 g, the supernatant filtered (PVDF, 0.22 µm) and used for quantitation of the saponins by UHPLC-HRESIMS (see Phytochemical analysis and standardization of DEAG section). The results are expressed as a percent of the dose of DEAG in the formulated tablets (150 mg), recalculated as the initial saponins content in it (see Preparation, analysis and standardization of DEAG section).
Results
Preparation, analysis and standardization of DEAG
After semi-industrial scale Soxhlet extraction and lyophilisation, a dry extract was obtained (40.78 g), comprising 20.39% of the plant material. The UHPLC-HRESIMS qualitative analysis revealed that DEAG contained flavonoids (camelliaside A, mauritianin and rutin) and triterpenoid saponins. This was in accordance with previous reports [Citation13]. In DEAG, the main saponin (ECAS) was further used for the quantitative determination (standardization) as an analytical marker. Supplemental Figure S2 depicts the total ion chromatogram of a methanol solution of DEAG in the negative ion mode. The quantitative analysis showed that DEAG contained 3.09% total saponins, expressed as ECAS.
Cytotoxicity assay
The MTT colorimetric assay was used for evaluation of the cytotoxicity of the tested extract and ACV (used as a reference ‘gold standard’ substance) in MDBK cells. The combined cytotoxicity was determined and concentrations equivalent to EC50 and below showed no detectable cytotoxicity. The DEAG and ACV were applied in concentrations ranging from 0.00625 mg/mL to 8 mg/mL and from 0.00187 mg/mL to 1 mg/mL, respectively. The obtained values for MNC and CC50 are presented in .
Table 1. Toxicity (cell viability) and cell protection by DEAG and Acyclovir (ACV) added simultaneously with and 1 h after the inoculation of the MDBK cell monolayer with SvHA1 (F strain) and SvHA2 (DD strain).
MTT-based colorimetric assay for detection of HuAHV replication inhibition
In both experimental setups (see Materials and methods) MNC (see ) was used [Citation20]. ACV was used as the reference substance. The tested extract showed dose-dependent antiviral potential ( and ). Against strain F, the MNC of DEAG resulted in 65.065% ±3.135 cell protection (EC50 was 0.363 mg/mL ±0.06) when added simultaneously with the virus suspension and 74.49% ±5.26 cell protection (EC50 was 0.4 mg/ml ±0.056) when added 1 h after virus adsorption. A similar trend was observed for the DD strain (, ). The MNC of DEAG, when added simultaneously with the DD strain, gave up to 61.485% ±0.445 protection of the infected monolayer (EC50 was 0.385 mg/mL ±0.019). When added after adsorption of the DD strain, the test substances showed up to 68.865% ±6.705 of cell protection (EC50 was 0.268 mg/mL ±0.074). These results indicate that DEAG mainly affects the intracellular stages of viral replication (antiviral activity is not dependent on the time of administration).
Figure 1. Antiviral activity of DEAG according to an MTT-based colorimetric assay. Virus replication inhibition when DEAG was added simultaneously with the inoculation of a MDBK cell monolayer with SvHA1 (F strain) (


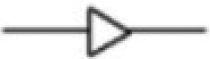

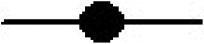
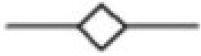
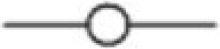
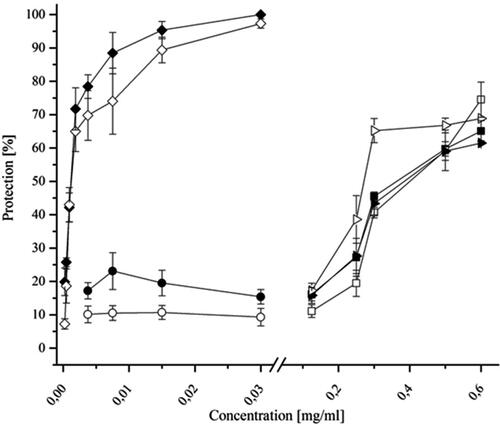
The data for ACV demonstrated its higher antiviral effect and selectivity index (SI) in comparison to those of the tested extract against the F strain of SvHA type 1. However, ACV was not active against the ACV-resistant DD strain of SvHA type 2, while DEAG maintained its activity.
Effect of the extract on viral infectivity (virucidal activity)
The effect of the extract on extracellular virions was investigated. The titers of the F strain of SvHA1 and the DD strain of SvHA2 were 88 × 106 PFU/mL and 38 × 106 PFU/mL, respectively. No virucidal activity was observed against either of the two virus strains: the titers of treated and control virus samples were similar.
Evaluation of the combined antiviral effects
Combining two or more antiviral substances in a suitable way limits the possibility for selection of resistant virus mutants. Also, a stronger antiviral effect can be achieved at lower concentrations of the active compounds than when they are administered alone.
First, the cytotoxic potential of the tested extract and ACV administered in combination was evaluated. No reduction in the viability of the treated cells was detected at the tested combinations of extract and ACV (the cell viability remained 100% after treatment with the extract and ACV in combination at the concentrations applied in this study.
To test the combined action of the extract and ACV on SvHA replication, concentrations corresponding to the EC50 value determined in an MTT-based colorimetric assay were used. The concentrations used in the evaluation of the combined antiviral effects are significantly lower than MNC. Combined cytotoxicity was determined and there was no detectable cytotoxicity in EC50 concentrations and below. In the experimental setting, the extract and ACV were added 1 h after inoculation of the virus into the cell monolayer (). The value of EC50 of DEAG and ACV correspond to that determined in the same experimental setting as that used in the MTT-based colorimetric assay for SvHA replication inhibition ().
When concentrations corresponding to EC50/2, EC50/4, EC50/8, EC50/16 of ACV were combined with all concentrations of DEAG, the highest observed synergism value reached 7.94 mg/mL2%, and that of the observed antagonism reached −19.41 mg/mL2% ().
Figure 2. Combined effects of acyclovir and DEAG on the replication of SvHA type 1 in MDBK cells. Acyclovir and DEAG were added 1 h after inoculation of the virus into the cell monolayer, and the inhibition % was determined following incubation for 120 h.

Following the guidelines for interpreting results obtained by MacSynergyTM II [Citation22,Citation23], the observed values of synergy at 95% confidence were regarded as insignificant. On the other hand, when all concentrations of DEAG were combined with the concentration corresponding to EC50 of ACV, the highest value of the observed antagonism was − 41.41 mg/mL2% (). The concentration corresponding to EC50/8 of ACV combined with the concentration corresponding to EC50 of DEAG led to insignificant synergism. Concentrations corresponding to EC50/2, EC50/4, EC50/8 and EC50/16 of ACV were combined with all concentrations of DEAG (excluding the mentioned above combination between the concentration corresponding to EC50/8 of ACV and the concentration corresponding to EC50 of DEAG), which led to insignificant antagonism. All concentrations of DEAG (EC50/2, EC50/4, EC50/8 and EC50/16) used in the experiment combined with the concentration corresponding to EC50 of ACV led to significant antagonism (). According to the same guidelines [Citation22,Citation23], the observed values of antagonism at 95% confidence were regarded as significant. These results indicate that DEAG is not suitable to be used as a partner in combination with ACV.
Formulation of tablets containing DEAG
In most cases, natural products have a SI that is much lower than the SI of chemotherapeutics. The advantages of natural products are in other areas of antiviral therapy such as more difficult development of antiviral resistance and fewer side effects. In most cases, production of tablets containing plant extracts is a technological challenge because of their tendency to absorb moisture and to become sticky. The encouraging results for the antiviral effect of the DEAG prompted an attempt to formulate the standardized extract into an appropriate dosage form. Since tablets are the most convenient oral dosage form, our attempts were to prepare tablets via direct compaction. Model tablets containing 150 mg of DEAG were developed with different excipients aiming to obtain optimal characteristics of the formulation. The selected excipients and their amounts in the formulations are presented in .
Table 2. Composition of the model tablets (mg/per tablet).
The resistance to crushing and disintegration ability is very important properties of tablets because they influence the release rate of the incorporated active substance. Our tests showed that the model tablets containing a higher amount of MCC possessed longer disintegration time than the required Pharmacopeial time for non-coated tablets (<15 min) (). These model tablets (I and II) showed 20- and 19-min disintegration time, which did not meet the Pharmacopeial criteria. The tablets formulated with a lower concentration of MCC disintegrated in less than 15 min and met the requirements. These tablets possessed lower resistance to crushing than model tablets I and II (), which probably contributed to a shorter disintegration time (). The lower resistance to crushing could be a prerequisite for an increased friability. However, the tests showed that all model tablets covered the Pharmacopeial criteria and demonstrated friability ranging from 0.5% to 0.7%.
Based on the results for disintegration time, resistance to crushing and friability, tablets Model IV were selected as more appropriate (). The in vitro release from these tablets was evaluated by applying the paddle method in distilled water. The samples taken at certain time intervals were examined with respect to the released saponins. The data showed that 89% of the active compound was released in 30 min (). The complete release of the active compound in 75 min is a prerequisite for further absorption of the active molecules.
Figure 4. Appearance of the model tablets IV (a) and in vitro release profile of the active compound from the developed tablets (b). Data are mean values ± SD from 3 replicates. Model tablets IV: 150 md DEAG, 43 mg microcrystalline cellulose, 150 mg lactose monohydrate, 5.25 talc and 1.75 magnesium stearate.
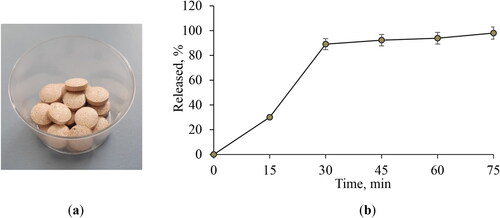
Discussion
More than 200 species of viruses can infect humans, and their number, albeit slowly, is increasing every year [Citation24]. Mortality and morbidity whose cause are viral infectious diseases negatively affect every aspect of life. Plants are a source of substances with biological activity. The lack of reports on the antiviral effects of A. glycyphyllos, its traditional use in folk medicine and existing knowledge about its phytochemical constituents prompted us to investigate the effect of DEAG in vitro alone and in combination with ACV (as the gold standard) against replication of SvHA type 1 and 2. The toxicity of the studied extract was significantly lower compared to the toxicity exhibited by the reference inhibitor ACV. This is one of the advantages of the plant extract compared to the nucleoside analogue. DEAG showed high antiviral potential in vitro (∼70% of protection) against replication of SvHA type 1 strain F and SvHA type 2 strain DD (ACV resistant). The antiviral activity did not differ when added simultaneously with or 1 h after virus inoculation into the cell monolayer. The extract showed significant efficacy against the ACV-resistant DD strain of SvHA type 2 while ACV (used as a reference substance) did not show any activity.
Resistance to the medicinal substances used often develops in the therapy of herpes infections. The activity of the studied DEAG against the ACV-resistant DD strain is of particular interest, given the increasingly difficult fight against emerging resistant virus strains. The absence of a significant difference in the activity of the extract in the two experimental setups used to determine its antiviral effect as well as the lack of virucidal activity, suggests that the action of the extract mainly affects the intracellular stages of viral replication.
The long-term and sometimes indiscriminate use of compounds in medical practice in the treatment of viral diseases leads to the emergence of viruses resistant to these compounds. Developed resistance to one substance very often leads to cross-resistance to other active compounds of the same class. This largely limits the choice of medications for adequate treatment. One of the most commonly used substances in the treatment of herpes infections is ACV. For this reason, and also for the reasons stated above, we chose it as the partner of DEAG in the experimental setup to evaluate the combined antiviral effects against SvHA replication. Combining substances that exhibit antiviral activity is a suitable way to limit the possibility of selection of resistant virus mutants and to apply lower concentrations of the active compounds than when they are administered alone. However, our results indicate that DEAG is not suitable for use as a partner in combination with ACV, and it is not promising to conduct experiments in vivo against infection caused by Simplexvirus humanalpha type 1. Positive values indicating statistically insignificant synergism and negative values indicating antagonism were reached when combining concentrations corresponding to EC50/2, EC50/4, EC50/8, EC50/16 of ACV with all concentrations of DEAG used in the experiment. In addition, all concentrations of DEAG used in the experiment combined with the concentration corresponding to EC50 of ACV led to negative values, indicating significant antagonism.
To our knowledge, this is the first study of antiviral effects of A. glycyphyllos. For the most used representative of the genus – Astragalus membranaceus, a recent study [Citation25] showed anti-influenza virus activity on RAW264.7 cells. Increased SOD activity and reduced MDA content in cells were found. The extract of A. membranaceus regulated the expression of TLR3, TAK1, TBK1, IRF3 and IFN-β in the TLR3-mediated signaling pathway [Citation25]. Antiviral activity of A. membranaceus aqueous and methanol root extracts was reported against Avian influenza H9 virus [Citation26]. The toxicity profile of extracts was evaluated using chicken embryos and the cytotoxic activity by MTT colorimetric assay using BHK-21 cells. None of the tested concentrations of aqueous and methanol A. membranaceus root extracts caused chicken embryo mortality [Citation26]. In agreement with these observations, no toxicity was found for DEAG in our study. The DEAG was standardized by its triterpenoid saponin content.
It is known that many saponins have immune stimulating effects. The main saponin in A. membranaceus, Astragaloside IV, has been approved as an antiviral agent by the China National Medical Products Administration for testing in people and its potential for repurposing has attracted attention [Citation27]. Thus, repurposing of saponins contained in other Astragalus species could also be a future strategy for antiviral treatment.
Secondary metabolites are the priority leading group of compounds when antiviral activities are tested. Lancifolamide isolated from Conocarpus lancifolius methanol extract, showed not only cytotoxic effects against a panel of cancer cells (human breast cancer, murine lymphocytic leukemia, and normal cells, human embryonic kidney cells and rat glioma cells), but also antiviral activity against HSV-1 [SvHA1] with 26% inhibition [Citation28]. The outcomes of that study support the use of plant-derived extracts and isolated compounds as possible/plausible antivirals [Citation28]. A methanol extract of Thymelaea microphylla containing saponins, exhibited prominent anti-proliferative activities against cancer A-549 cells, high anti-inflammatory activity in RAW 264.7 cells and anti-herpes (HSV-1) effects, acting both directly on the virus and on its adsorption [Citation29]. As a major antiviral drug to treat HSV (SvHA) infections, ACV has been proven unsatisfactory owing to frequent reports of resistance and severe side effects in pregnant mothers and infants. The high prevalence of HSV [SvHA], lack of vaccines and limited treatment options warrant an urgent need for more effective anti-HSV agents [Citation30]. An assay of the antiviral effects of yet another plant extract from Dichrocephala integrifolia, which contains flavonoids and saponins, similar to the DEAG tested in the present study, demonstrated that it inhibited HSV-1 virus from causing CPE [Citation30].
Formulation of a dosage form containing a quantified extract from A. glycyphyllos has not been previously reported. Our results contribute to the accumulating evidence for the antiviral activity of representatives of the genus Astragalus already documented [Citation25–27].
Conclusions
A dry extract of the aerial parts of A. glycyphyllos was prepared, analysed by UHPLC-HRESIMS and standardized in respect of saponins. The extract showed high antiviral potential against replication of F strain of SvHA type 1 and the DD strain of SvHA type 2 (ACV resistant). The activity of the extract did not differ when it was added simultaneously with and 1 h after the cell inoculation. This and the lack of virucidal activity suggests that the extract mainly affects the intracellular stages of viral replication. The extract turns out to be effective against the ACV-resistant DD strain of SvHA type 2 while ACV (used as a reference substance) did not show any activity. Combination between the extract and ACV against SvHA type 1 led to the conclusion that DEAG is not suitable to be used as a partner in combination with ACV against replication of SvHA type 1 in vitro. Finally, the standardized extract was successfully formulated in tablet form, which, after optimization of the composition, meets the Pharmacopeial requirements.
Authors’ contributions
AS, AH, VC, KS, DT, KY and IS performed the study and analysed the data. AS, AH, IK, SS, IS and KY wrote the paper. IK, KY and SS administered the project and provided funding. All authors read and approved the final manuscript.
Supplemental Material
Download PDF (798.7 KB)Data availability statement
The experimental data supporting this study are freely available from the corresponding author (I.K.) upon reasonable request.
Disclosure statement
No potential conflict of interest was reported by the authors.
Additional information
Funding
References
- Asyov B, Petrova A, Dimitrov D, et al. Conspectus of the Bulgarian vascular flora. Distribution maps and floristic elements. Bulgarian biodiversity foundation. Sofia (Bulgaria): Bulgarian Biodiversity Foundation; 2012.
- Lysiuk R, Darmohray R. Pharmacology and ethnomedicine of the genus Astragalus. IJPPE. 2016;3:1–11. doi: 10.18052/www.scipress.com/IJPPE.3.46.
- Krasteva I, Shkondrov A, Ionkova I, et al. Advances in phytochemistry, pharmacology and biotechnology of Bulgarian Astragalus species. Phytochem Rev. 2016;15(4):567–590. doi: 10.1007/s11101-016-9462-4.
- Bratkov V, Shkondrov A, Zdraveva P, et al. Flavonoids from the genus Astragalus: phytochemistry and biological activity. Pharmacogn Rev. 2016;10(19):11–32. Available from: http://www.phcogrev.com/text.asp?2016/10/19/11/176550 doi: 10.4103/0973-7847.176550.
- Shkondrov A, Krasteva I, Bucar F, et al. A new tetracyclic saponin from Astragalus glycyphyllos L. and its neuroprotective and hMAO-B inhibiting activity. Nat Prod Res. 2020;34(4):511–517. Available from: doi: 10.1080/14786419.2018.1491040.
- Ionkova I, Shkondrov A, Zarev Y, et al. Anticancer secondary metabolites: from ethnopharmacology and identification in native complexes to biotechnological studies in species of genus Astragalus L. and Gloriosa L. Curr Issues Mol Biol. 2022;44(9):3884–3904. Available from: https://www.mdpi.com/1467-3045/44/9/267 doi: 10.3390/cimb44090267.
- Shkondrov A, Simeonova R, Kondeva-Burdina M, et al. Study to evaluate the antioxidant activity of Astragalus glycyphyllos extract in carbon tetrachloride-induced oxidative stress in rats. EJMP. 2015;7(2):59–66. Available from: http://www.sciencedomain.org/abstract.php?iid=1018&id=13&aid=8249 doi: 10.9734/EJMP/2015/16259.
- Georgieva A, Popov G, Shkondrov A, et al. Antiproliferative and antitumour activity of saponins from Astragalus glycyphyllos on myeloid Graffi tumour. J Ethnopharmacol. 2021;267:113519. doi: 10.1016/j.jep.2020.113519.
- Salehi B, Carneiro JNP, Rocha JE, et al. Astragalus species: insights on its chemical composition toward pharmacological applications. Phyther Res. 2021;35(5):2445–2476. Available from: https://onlinelibrary.wiley.com/doi/abs/10.1002/ptr.6974 doi: 10.1002/ptr.6974.
- Pistelli LF. Secondary metabolites of genus Astragalus: structure and biological activity. In: Atta-ur-Rahman, editor. Studies in natural products chemistry. Karachi: Elsevier Science BV; 2002. p. 443–545.
- Verotta L, El-Sebakhy NA. Cycloartane and oleanane saponins from Astragalus sp. In: Atta-ur-Rahman, editor. Studies in natural products chemistry. Bioactive natural products (Part F) (Volume 25). Karachi: Elsevier Science BV; 2001. p. 179–234. Available from: https://www.sciencedirect.com/science/article/pii/S1572599501800089
- Zhang P, Liu X, Liu H, et al. Astragalus polysaccharides inhibit avian infectious bronchitis virus infection by regulating viral replication. Microb Pathog. 2018;114:124–128. Available from: https://www.sciencedirect.com/science/article/pii/S0882401017313979 doi: 10.1016/j.micpath.2017.11.026.
- Shkondrov A, Krasteva I. High resolution LC-MS/MS screening for secondary metabolites in Bulgarian species of genus Astragalus L. Quim Nova [Internet]. 2021;44(6):683–688. Available from: http://quimicanova.sbq.org.br/audiencia_pdf.asp?aid2=9283&nomeArquivo=AR2020-0353.pdf
- Shkondrov A, Krasteva I, Ionkova I, et al. Production of saponins from in vitro cultures of Astragalus glycyphyllos and their antineoplastic activity. Biotechnol Biotechnol Equip [Internet]. 2019;33(1):1413–1418. Available from: doi: 10.1080/13102818.2019.1671222.
- Shkondrov A, Krasteva I. Liquid chromatography – high resolution mass spectrometry screening of Astragalus hamosus and Astragalus corniculatus. PHAR. 2021;68(1):135–139. Available from: doi: 10.3897/pharmacia.68.e60621.
- Mosmann T. Rapid colorimetric assay for cellular growth and survival: application to proliferation and cytotoxicity assays. J Immunol Methods. 1983;65(1–2):55–63. doi: 10.1016/0022-1759(83)90303-4.
- Reed LJ, Muench H. A simple method of estimating fifty per cent endpoints. Am J Epidemiol. 1938;27(3):493–497. doi: 10.1093/oxfordjournals.aje.a118408.
- Dulbecco R. Production of plaques in monolayer tissue cultures by single particles of an animal virus. Proc Natl Acad Sci U S A. 1952;38(8):747–752. doi: 10.1073/pnas.38.8.747.
- Takeuchi H, Baba M, Shigeta S. An application of tetrazolium (MTT) colorimetric assay for the screening of anti-herpes simplex virus compounds. J Virol Methods. 1991;33(1-2):61–71. Available from: https://www.sciencedirect.com/science/article/pii/016609349190008N doi: 10.1016/0166-0934(91)90008-n.
- Hinkov A, Angelova P, Marchev A, et al. Nepeta nuda ssp. nuda L. water extract: inhibition of replication of some strains of human alpha herpes virus (genus simplex virus) in vitro, mode of action and NMR-based metabolomics. J Herb Med. 2020;21:100334. Available from: https://www.sciencedirect.com/science/article/pii/S2210803320300063 doi: 10.1016/j.hermed.2020.100334.
- Prichard MN, Shipman C. A three-dimensional model to analyze drug-drug interactions. Antiviral Res. 1990;14(4–5):181–205. Available from: https://www.sciencedirect.com/science/article/pii/016635429090001N doi: 10.1016/0166-3542(90)90001-n.
- Prichard MN, Aseltine KR, Shipman CJr. MacSynergyTM II. Ann Arbor (MI): University of Michigan; 1992.
- Prichard MN, Aseltine KR, Shipman CJr. User’s manual. Ann Arbor (MI): University of Michigan; 1993.
- Lopez-Santamarina A, Lamas A, del Carmen Mondragón A, et al. Probiotic effects against virus infections: new weapons for an old war. Foods [Internet]. 2021;10(1):130. Available from: https://www.mdpi.com/2304-8158/10/1/130 doi: 10.3390/foods10010130.
- Liang Y, Zhang Q, Zhang L, et al. Astragalus membranaceus treatment protects Raw264.7 cells from influenza virus by regulating G1 phase and the TLR3-Mediated signaling pathway. Evid Based Complement Alternat Med. 2019;2019:2971604. doi: 10.1155/2019/2971604.
- Khan HM, Raza SM, Anjum AA, et al. Antiviral, embryo toxic and cytotoxic activities of Astragalus membranaceus root extracts. Pak J Pharm Sci. 2019;32(1):137–142.
- Yang K, Xie Q, Tang T, et al. Astragaloside IV as a novel CXCR4 antagonist alleviates osteoarthritis in the knee of monosodium iodoacetate-induced rats. Phytomedicine. 2023;108:154506. doi: 10.1016/j.phymed.2022.154506.
- Saadullah M, Farid A, Ali A, et al. Molecular modeling study of novel lancifolamide bioactive molecule as an inhibitor of acetylcholinesterase (AChE), herpes simplex virus (HSV-1), and anti-proliferative proteins. Molecules. 2022;27(17):5480. doi: 10.3390/molecules27175480.
- Mansour RB, Chaouachi F, Falleh H, et al. Powerful anti-inflammatory, anti-Herpes and anticancer capacities of Thymelaea microphylla L. and TLC phenolic identification. J Nat Prod Res Appl. 2022;1(3):1–16. doi: 10.46325/jnpra.v1i03.24.
- Hadun AH, Mbaria J, Aboge GO. Phytochemical composition of Dichrocephala integrifolia crude extracts, antiviral activity and toxicity. Asian J Trop Biotechnol. 2022;19(2):72–81. doi: 10.13057/biotek/c190204.