Abstract
The aim of this prospective study was to compare the endometrial microbiome between pregnant and non-pregnant women after frozen embryo transfer (FET) with euploid embryos. Endometrial biopsies were collected from 30 women during the mid-luteal phase in a natural cycle. FET was performed with euploid embryos up to 3 months after the biopsy. Endometrial microbiota composition was analysed using 16S rRNA (v4-v5 region) next generation sequencing (NGS). The analysis of different clinical outcomes after the biopsy (no pregnancy (n = 14), and ultrasound confirmed pregnancy (n = 16)) revealed differences in the endometrial microbiome composition. In total, 271 distinct bacterial species and 668 bacterial genera were identified. The number of unique species found in non-pregnant women was 62 (22.88%), while in the patients who became pregnant after FET it was 39 (14.39%). Among them, bacteria with high frequency of occurrence such as Bacteroides spp., Cutibacterium granulosum, Isoptericola spp., Acetomicrobium spp., Marivivens spp. and Syntrophomonas spp. were found only in non-pregnant patients, while Bosea spp. was present only in pregnant women. The analysis of bacteria relative abundance revealed that Lactobacillus genus was not significantly different between the studied groups. In contrast, Serratia marcescens, Staphylococcus spp., Glutamicibacter spp. and Delftia spp. were significantly enriched in the non-pregnant group. In conclusion, specific bacteria taxa had higher relative abundance in the endometrium of patients with implantation failure after FET with euploid embryos. We hypothesize that an appropriate treatment for optimization of endometrial microbiome content in women with diagnosed microbiome dysbiosis could be beneficial for improvement of pregnancy rates.
Introduction
Worldwide infertility has been estimated to affect between 8% and 12% of reproductive age couples [Citation1]. Although assisted reproductive technologies (ARTs) have made great progress during the last decades, major challenges remain, like achieving successful implantation after embryo transfer, as well as early pregnancy loss.
Several studies have revealed a significant association between the endometrial microbiome composition and implantation failure [Citation2–4], miscarriage [Citation5] or spontaneous preterm birth [Citation6, Citation7]. The occurrence of bacterial dysbiosis and the prevalence of certain pathogenic bacterial taxa might be an indicator of impaired endometrial receptivity, which could negatively affect the clinical pregnancy rate in in vitro fertilization (IVF) patients [Citation8, Citation9].
Some authors report that a decrease in the number of the dominant Lactobacillus species in the endometrium would lead to a measurable increase in the pH of the endometrial fluid, which could affect embryo attachment and adhesion abilities and would allow a significant increase of certain pathogenic bacteria [Citation10]. In contrast, other studies suggest that the abundance of Lactobacillus spp. could not result in a significant change in uterine pH [Citation3]. These conflicitng results suggest that other endometrial factors associated with the microbiome could lead to impaired endometrial receptivity and implantation failure [Citation11].
However, there is still scarce information about the influence of the relative abundance of certain bacterial taxa on the pregnancy outcome in patients with idiopathic infertility and frozen embryo transfer (FET). The aim of this study was to compare the endometrial microbiome between pregnant and non-pregnant women after FET with euploid embryos.
Subjects and methods
Ethical approval
The study protocol was approved by Nadezdha Women’s Health Hospital Ethics Committee (No:56/29.12.2020). To ensure anonymity, patients’ information was deidentified. Informed consent was obtained from each patient and their partner.
Patient selection and experimental design
The study was performed at Nadezhda Women’s Health Hospital, Sofia, Bulgaria, and Medical University of Sofia, Sofia, Bulgaria, between March 2021 and December 2022. During the study period, 30 women eligible for inclusion were enrolled and analysed in this cohort prospective study. The inclusion criteria were: infertile women aged between 34 and 45 y, undergoing IVF treatment. The exclusion criteria for the patients were male factor infertility, history of recent inflammatory disease, chronic endometritis, history of recent antibiotic treatment, moderate or severe endometriosis, adenomyosis, uterine hyperplasia and endometrial polyps.
The collected data included participant baseline characteristics (age, body mass index (BMI)), and serum progesterone and estrogen. The patients enrolled in the study were grouped on the basis of the success of their FET performed with euploid embryos (confirmed by preimplantation genetic testing for aneuploidy (PGT-A)), up to 3 months after the biopsy. The first group included women who achieved clinical pregnancy, and the second group comprised of women who were not pregnant. Clinical pregnancy was confirmed by ultrasonographic evidence of an intrauterine gestational sac and foetal heartbeat, two weeks after determined biochemical pregnancy.
Sample collection
The endometrial biopsies were taken using a Pipelle endometrial suction curette 7 days after the luteinizing hormone surge in a natural cycle. All endometrial biopsies were collected in sterile tubes (Falcon 15 mL) and stored at −80 °C until further use.
Total DNA extraction and NGS sequencing
Total DNA was extracted from all endometrial samples using Quick-DNA Fecal/Soil Microbe Microprep Kit (D6010, Zymo Research, USA) in compliance with the manufacturer’s instructions. The extracted DNA was quantified using the NanoDrop™ Spectrophotometer 2000c (Thermo Scientific, Waltham, MA, USA).
PCR amplification of targeted regions was performed using specific primers connecting with barcodes. The PCR products with proper size were selected by 2% agarose gel electrophoresis. A standard procedure was used as described previously [Citation12]. The same amount of PCR products from each sample was pooled, end-repaired, A-tailed and further ligated with Illumina adapters. Libraries were sequenced on a paired-end Illumina platform to generate 250-bp paired-end raw reads. The library was checked with Qubit and real-time PCR for quantification and bioanalyzer for size distribution detection. Quantified libraries were pooled and sequenced on Illumina platforms, according to effective library concentration and data amount required.
Sequencing data processing
The DNA samples were shipped to the Novogene Company Ltd. (Cambridge, UK) at ambient temperature. The barcoded primers 515 F (5-GTGCCAGCMGCCGCGGTAA-3′) and 907 R (5-CCGTCAATTCCTTTGAGTTT-3′) were used to amplify 16S rRNA genes covering the V4–V5 region [Citation13, Citation14]. The sequencing was performed on the Illumina HiSeq 2 × 250 bp paired-end reads platform with 30 k tags per sample, using the V4-V5 region of the 16S rRNA genes as an amplicon target. The sequence reads were assigned to different operational taxonomic units (OTUs), and the background-contaminating bacteria were excluded from the analysis.
OTU species assignment
Sequence analysis was performed by Uparse software (Uparse v7.0.1090) [Citation15] using all the effective tags. Sequences with ≥97% similarity were assigned to the same OTUs. The representative sequence for each OTU was screened for further annotation. For each representative sequence, Qiime (version 1.7.0) [Citation16] in Mothur method was performed against the SSUrRNA database of SILVA138 database [Citation17] for species annotation at each taxonomic rank (Threshold: 0.8 ∼ 1) [Citation18] (kingdom, phylum, class, order, family, genus, species). OTUs abundance information was normalized using a standard of sequence number corresponding to the sample with the least number of sequences. These output normalized data served as a basis for subsequent analysis of alpha diversity and beta diversity.
Alpha diversity was applied to analyse the complexity of biodiversity for a sample through Shannon index. It was calculated with QIIME (version 1.7.0) and displayed with R software (version 2.15.3). Beta diversity analysis was used to evaluate differences between samples in species complexity. Beta diversity on unweighted unifrac was calculated by QIIME software (version 1.7.0).
Statistical analysis
Patient data were analysed with SPSS v.21 (IBM, Armonk, NY). Quantitative data were expressed as mean values with standard deviation (±SD), and percentage (%). Student’s t-test or Mann-Whitney U test for the relative abundance of each bacteria taxa were applied according to normal or not normal distribution of data. A Bonferroni correction was applied to the significant levels obtained. The significance level was set at p < 0.05.
Results
Baseline characteristics of study population
To analyse the potential differences in endometrial microbiome composition between patients with subsequent successful and unsuccessful FET, we compared a group of 16 women who became pregnant with a group of 14 non-pregnant women after the performed assisted reproduction procedure.
The baseline characteristics of patients with successful and non-successful subsequent pregnancy are shown in . The age of the women was 34–45 years, and their BMI was between 19 and 27 kg/m2. There were no statistically significant differences in age, BMI or serum levels of progesterone and estradiol between the two groups.
Table 1. Baseline characteristics of the patients in the two groups.
Endometrial microbiota composition and relative abundance in patients with subsequent successful or unsuccessful pregnancy after FET
In total, 2168 OTUs were identified across the 30 endometrial samples from patients with subsequent successful or unsuccessful pregnancy after FET. The dataset included bacteria from 271 species, 668 genera, 391 families, 245 orders, 112 classes and 50 phyla. The number of unique species found in non-pregnant women was 62 (22.88%), while in those who became pregnant after the FET, it was 39 (14.39%). Among them, bacteria with a high frequency of occurrence (found in more than 30% of the samples) such as Cutibacterium granulosum, Isoptericola spp., Acetomicrobium spp., Marivivens spp., Syntrophomonas spp. and Bacteroides spp. were found only in patients with unsuccessful pregnancy, while Bosea spp. was present only in women with successful pregnancy.
The taxonomic microbial profiling of the endometrial samples of women who became pregnant after FET revealed a bacterial community represented by 10 genera that accounted for more than 45% of the total genera (sequence reads). Among them, the Ralstonia genus (28.89%) was followed by Lactobacillus spp. (14.44%), Pseudomonas spp. (0.77%) and Delftia spp. (0.21%) in the group with pregnancy (). For non-pregnant women, Ralstonia genus (33.88%) was followed by Lactobacillus spp. (10.16%), Ureaplasma spp. (1.27%), Faecalibacterium spp. (0.89%), Pseudomonas spp. (0.87%) and Delftia spp. (0.72%).
Figure 1. Taxa relative abundance at the genus level. Y-axis represents ‘relative abundance’ and X-axis represents ‘samples name’ where ‘A’ are samples from non-pregnant women and ‘B’ are samples from women with subsequent pregnancy after FET. "others" represents the total relative abundance of the rest of the phyla besides the top 10 genera.
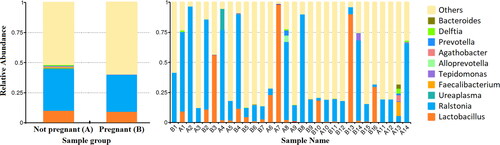
Based on the analysis of bacteria relative abundance, Lactobacillus genus was not significantly different between the studied groups. In contrast, Serratia marcescens, Staphyloccocus spp., Delftia spp. and Glutamicibacter spp. were significantly enriched in the non-pregnant group (p < 0.05) ().
Taxonomic abundance cluster heatmap
A heatmap was generated to examine the similarity and difference between the endometrial samples, on the basis of the abundance data of the top 35 genera of all samples (). The analysis revealed that most bacterial genera belonging to Bacteroidota, Proteobacteria, Firmicutes and Actinobacteriota were enriched in the non-pregnant group.
Figure 3. Taxonomic abundance cluster heatmap (plotted by sample name on the X-axis and the Y-axis represents the genus. The absolute value of ‘z’ represents the distance between the raw score and the mean of the standard deviation. ‘Z’ is negative when the raw score is below the mean, and vice versa. ‘A’ – samples from non-pregnant patients, ‘B’ – samples from pregnant patients (with subsequent pregnancy after FET).
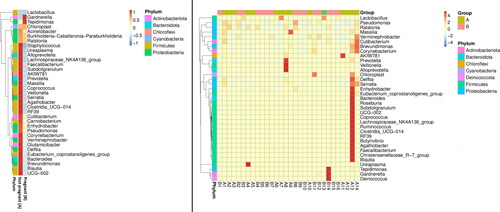
Alpha and beta diversity
Analysis of the microbial alpha diversity showed that Shannon indices in endometrial samples from patients who became pregnant and those who did not were similar (2.78 ± 1.73 vs. 2.43 ± 0.6, p = 0.46). Flower plots were used to show the shared and unique taxa (OTUs) in each studied group. The number of shared taxa (OTUs) was also similar between the studied groups (16 vs. 15) (). In addition, no discrepancy was found in beta diversity based on the unweighted UniFrac ANOSIM metric (qualitative, ANOSIM R = −0.002, p = 0.40).
Figure 4. Differences in the defined taxa between endometrial samples from non-pregnant women and those who became pregnant after FET. (A) Box plots showing the observed number of species; (B) Box plots showing the Shannon indices (alpha diversity); (C) Box plots showing unweighted analyses of similarities (ANOSIM) (beta diversity) based on the distance matrix of UniFrac dissimilarity of the endometrial microbial communities in the pregnant and non-pregnant patients. Respective ANOSIM R values show the community variation between the groups and P values are indicated. The axes represent the two dimensions explaining the greatest proportion of variance in the communities; (D) Venn diagram – each circle represents one group – pregnant and non-pregnant women. Values in overlapping parts represent common species. The rest are species specific for each group. (E) Flower diagram based on operational taxonomic units (OTUs); notes: each petal in the flower diagram represents one sample, with different colours for different samples. The core number in the Centre is for the number of OTUs present in all samples, while the number in the petals is for the unique OTUs only showing in each sample. ‘A’ – samples from non-pregnant patients, ‘B’ – samples from patients who became pregnant after FET.
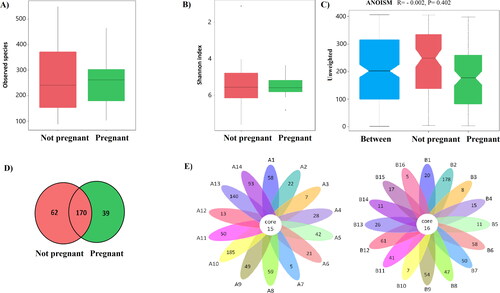
Discussion
Although important shifts in bacterial communities were observed in relation to women age, hormonal changes and menstrual cycle phase [Citation19–21], our study was focused on a representative group of women in our fertility clinic with progesterone support, and all samples were taken during the mid-luteal phase when the embryo implantation occurs.
When we compared the alpha and beta diversity of endometrial microbial community by cohort, no significant difference was obtained (p > 0.05). Our result was in accordance with another study that showed similar diversity between patients with recurrent implantation failure (RIF) and controls [Citation22]. In addition, we did not find a significant difference in the relative abundance of Lactobacillus spp. between women who became pregnant or those who did not. Similar results were observed in other studies where RIF women were compared to a control group [Citation22, Citation23]. However, the relative abundance analysis revealed that some anaerobic bacteria such as Cutibacterium granulosum and Acetomicrobium spp., were present only in the group of women with unsuccessful implantation. Cutibacterium granulosum is a pathogenic anaerobe previously described in the human endometrial microbiome [Citation24]. This species can trigger immune cell modifications that involve dendritic cells, natural killer cells and macrophages [Citation25]. In addition, high diversity and dominance of Bacteroides spp. (which were present only in non-pregnant women) have been found in endometrial microbiota of cancer patients [Citation26] and have been associated with recurrent pregnancy loss and miscarriage in previous studies [Citation27].
Our study also showed a significant increase in the relative abundance of two well-known pathogenic species in non-pregnant women, Serratia marcescens and Staphyloccocus aureus. This could be associated with their cell-to-cell interaction. Recently, it was found that Serratia marcescens produce and introduce a specific compound with antimicrobial activity, prodigiosin, into Staphyloccocus aureus and thus it can kill or decrease its viability [Citation28–30]. However, Serratia marcescens can not only reduce the quantity of some pathogenic bacteria, but may also cause spontaneous miscarriages [Citation31]. On the other hand, Staphylococcus aureus could impair endometrial function by inducing epithelial cell necroptosis, triggered by membrane disruption and ion imbalance that leads to the rapid generation of reactive oxygen species and mitochondrial damage [Citation32]. Similarly, Serratia marcescens secrete hemolysin that causes irreversible vacuolation and subsequent lysis of the endometrial cells [Citation33]. The negative effect of these two species and possible synergetic action on the spermatozoa and implantation outcome has been studied in a mouse model [Citation34]. These pathogens may participate in the development of non-receptive endometrium and implantation failure, presumably by the induction of local immune reaction [Citation35], including by increase of neutrophils [Citation36]. S. aureus and S. marcescens are resistant to many antibiotics, including penicillin, colistin, tetracycline, cephalosporin, nitrofurantoin, and macrolide [Citation37, Citation38]. Possible treatment of acute infections should include carbapenems or aminoglycosides in combination with third-generation (and eventually fourth-generation) cephalosporin and oxacillin [Citation38, Citation39].
Delftia is another OTU with high relative quantity in non-pregnant women. The increased abundance of Delftia in RIF women is in accordance with another study [Citation22]. These aerobic, gram-negative rods are seldom implicated in human infections [Citation40]. Possible treatments aiming to reduce their number should be carefully applied, because representatives of this genus, such as Delftia acidovorans are susceptible to meropenem or ceftazidime but resistant to gentamicin and colistin [Citation41].
Unlike the bacteria described above, Bosea spp. was observed only in women with successful implantation. Few previous studies reported the presence of this genus in endometrial samples [Citation42]. Species from this genus are known to be multi-drug resistant [Citation43, Citation44] and more abundant in patients with ovarian or cervical cancer [Citation32, Citation45]. However, their possible effect on endometrial receptivity is a matter of future research.
The main limitation of our study was the relatively small sample size. In addition, transcervical endometrial biopsy may contribute to possible contamination of the samples with cervical microflora. Special caution was taken to avoid contact between the curette and the vaginal walls during the collection of each endometrial sample. Another limiting factor is that the endometrial microbiome might change over time between the sample collection timepoint, and embryo transfer. We tried to minimize this effect by keeping the interval between the endometrial biopsy sampling and the embryo transfer as short as possible.
Conclusions
In this study, the relative abundance of certain pathogenic bacteria such as Serratia marcescens, Staphylococcus aureus and Delftia spp., and the presence of other taxa such as Cutibacterium granulosum, Acetomicrobium spp. and Bacteroides spp., together with the depletion of Bosea spp. in human endometrium are related to implantation failure. The present data showed that endometrial microbial imbalance might be considered an important factor for unsuccessful embryo implantation in patients with idiopathic infertility. Further studies are required to reveal the exact mechanism of the described microbial dysbiosis and to determine adequate microbiome-targeted treatments.
Authors’ contributions
All authors have read and approved the paper, and meet the criteria for authorship as established by the International Committee of Medical Journals Editors.
Acknowledgments
The authors wish to thank Yoanna Baleva (Nadezhda Women’s Health Hospital, Sofia, Bulgaria) for her valuable proofreading help. The authors wish to thank Novogene Company Ltd. (Cambridge, UK) for NGS analysis.
Disclosure statement
The authors have no conflict of interest in this study.
Data availability statement
The data that support the findings of this study are available on request from the corresponding author.
Additional information
Funding
References
- Vander Borght M, Wyns C. Fertility and infertility: definition and epidemiology. Clin Biochem. 2018;62:1–9. doi: 10.1016/j.clinbiochem.2018.03.012.
- Franasiak JM, Scott RT. Reproductive tract microbiome in assisted reproductive technologies. Fertil Steril. 2015;104(6):1364–1371. doi: 10.1016/j.fertnstert.2015.10.012.
- Moreno I, Codoñer FM, Vilella F, et al. Evidence that the endometrial microbiota has an effect on implantation success or failure. Am J Obstet Gynecol. 2016;215(6):684–703. doi: 10.1016/j.ajog.2016.09.075.
- Reschini M, Benaglia L, Ceriotti F, et al. Endometrial microbiome: sampling, assessment, and possible impact on embryo implantation. Sci Rep. 2022;12(1):8467. doi: 10.1038/s41598-022-12095-7.
- Peuranpää P, Holster T, Saqib S, et al. Female reproductive tract microbiota and recurrent pregnancy loss: a nested case-control study. Reprod Biomed Online. 2022;45(5):1021–1031. doi: 10.1016/j.rbmo.2022.06.008.
- Jefferson КK. The bacterial etiology of preterm birth. Adv. Appl. Microbiol. 2012;80:1–22. doi: 10.1016/b978-0-12-394381-1.00001-5.
- Park S, You YA, Kim YH, et al. Ureaplasma and prevotella colonization with lactobacillus abundance during pregnancy facilitates term birth. Sci Rep. 2022;12(1):10148. doi: 10.1038/s41598-022-13871-1.
- Moreno I, Garcia-Grau I, Perez-Villaroya D, et al. Endometrial microbiota composition is associated with reproductive outcome in infertile patients. Microbiome. 2022;10(1):1. doi: 10.1186/s40168-021-01184-w.
- Lozano FM, Lledó B, Morales R, et al. Characterization of the endometrial microbiome in patients with recurrent implantation failure. Microorganisms. 2023;11(3):741. doi: 10.3390/microorganisms11030741.
- Sirota I, Zarek S, Segars J. Potential influence of the microbiome on infertility and assisted reproductive technology. Semin Reprod Med. 2014;32(1):35–42. doi: 10.1055/s-0033-1361821.
- Toson B, Simon C, Moreno I. The endometrial microbiome and its impact on human conception. Int J Mol Sci. 2022;23(1):485. doi: 10.3390/ijms23010485.
- Rustanti N, Murdiati A, Juffrie M, et al. Effect of probiotic Lactobacillus plantarum dad-13 on metabolic profiles and gut microbiota in type 2 diabetic women: a randomized double-blind controlled trial. Microorganisms. 2022;10(9):1806. doi: 10.3390/microorganisms10091806.
- Caporaso JG, Lauber CL, Walters WA, et al. Global patterns of 16S rRNA diversity at a depth of millions of sequences per sample. Proc Natl Acad Sci U S A. 2011;108 (Suppl 1):4516–4522. doi: 10.1073/pnas.1000080107.
- Schwieger F, Tebbe CC. A new approach to utilize PCR-single-strand-conformation polymorphism for 16S rRNA gene-based microbial community analysis. Appl Environ Microbiol. 1998;64(12):4870–4876. doi: 10.1128/AEM.64.12.4870-4876.1998.
- Edgar RC. UPARSE: highly accurate OTU sequences from microbial amplicon reads. Nat Methods. 2013;10(10):996–998. doi: 10.1038/nmeth.2604.
- Altschul SF, Gish W, Miller W, et al. Basic local alignment search tool. J Mol Biol. 1990;215(3):403–410. doi: 10.1016/S0022-2836(05)80360-2.
- Wang Q, Garrity GM, Tiedje JM, et al. Naive bayesian classifier for rapid assignment of rRNA sequences into the new bacterial taxonomy. Appl Environ Microbiol. 2007;73(16):5261–5267. doi: 10.1128/AEM.00062-07.
- Quast C, Pruesse E, Yilmaz P, et al. The SILVA ribosomal RNA gene database project: improved data processing and web-based tools. Nucleic Acids Res. 2013;41:D590–6. doi: 10.1093/nar/gks1219.
- Chen C, Song X, Wei W, et al. The microbiota continuum along the female reproductive tract and its relation to uterine-related diseases. Nat Commun. 2017;8(1):875. doi: 10.1038/s41467-017-00901-0.
- Carosso A, Revelli A, Gennarelli G, et al. Controlled ovarian stimulation and progesterone supplementation affect vaginal and endometrial microbiota in IVF cycles: a pilot study. J Assist Reprod Genet. 2020;37(9):2315–2326. doi: 10.1007/s10815-020-01878-4.
- Wang J, Li Z, Ma X, et al. Translocation of vaginal microbiota is involved in impairment and protection of uterine health. Nat Commun. 2021;12(1):4191. doi: 10.1038/s41467-021-24516-8.
- Ichiyama T, Kuroda K, Nagai Y, et al. Analysis of vaginal and endometrial microbiota communities in infertile women with a history of repeated implantation failure. Reprod Med Biol. 2021;20(3):334–344. doi: 10.1002/rmb2.12389.
- Diaz-Martínez MDC, Bernabeu A, Lledó B, et al. Impact of the vaginal and endometrial microbiome pattern on assisted reproduction outcomes. J Clin Med. 2021;10(18):4063. doi: 10.3390/jcm10184063.
- Bozkurt H, Güdücüoğlu H, Bayram Y, et al. Anaerobic bacteria isolated from various clinical specimens and their antibiotic susceptibilities. Van Tıp Derg. 2004;11:85–91. doi: 10.26650/IUITFD.2019.0074.
- Corvec S. Clinical and biological features of cutibacterium (formerly propionibacterium) avidum, an underrecognized microorganism. Clin Microbiol Rev. 2018;31(3):e00064–17. doi: 10.1128/CMR.00064-17.
- Medina-Bastidas D, Camacho-Arroyo I, García-Gómez E. Current findings in endometrial microbiome: impact on uterine diseases. Reproduction. 2022;163(5):R81–R96. PMID: 35195535. doi: 10.1530/REP-21-0120.
- Verstraelen H, Vilchez-Vargas R, Desimpel F, et al. Characterisation of the human uterine microbiome in non-pregnant women through deep sequencing of the V1-2 region of the 16 S rRNA gene. PeerJ. 2016;4:e1602. doi: 10.7717/peerj.1602.
- Darshan N, Manonmani HK. Prodigiosin inhibits motility and activates bacterial cell death revealing molecular biomarkers of programmed cell death. AMB Express. 2016;6(1):50. doi: 10.1186/s13568-016-0222-z.
- Lapenda JC, Silva PA, Vicalvi MC, et al. Antimicrobial activity of prodigiosin isolated from Serratia marcescens UFPEDA 398. World J Microbiol Biotechnol. 2015;31(2):399–406. doi: 10.1007/s11274-014-1793-y.
- Lim S, Bhak J, Jeon S, et al. The kiss of death: serratia marcescens antibacterial activities against Staphylococcus aureus requires both de novo prodigiosin synthesis and direct contact. Microbiol Spectr. 2022;10(3):e0060722. doi: 10.1128/spectrum.00607-22.
- Kljakić D, Milosavljević MZ, Jovanović M, et al. Serratia marcescens as a cause of unfavorable outcome in the twin pregnancy. Open Med (Wars). 2020;16(1):81–86. doi: 10.1515/med-2021-0205.
- Yi Y, Gao K, Lin P, et al. Staphylococcus aureus-Induced necroptosis promotes mitochondrial damage in goat endometrial epithelial cells. Animals (Basel). 2022;12(17):2218. doi: 10.3390/ani12172218.
- Hertle R, Hilger M, Weingardt-Kocher S, et al. Cytotoxic action of Serratia marcescens hemolysin on human epithelial cells. Infect Immun. 1999;67(2):817–825. doi: 10.1128/IAI.67.2.817-825.1999.
- Jangra I, Chauhan A, Prabha V. Synergistic interactions of sperm impairing bacteria: impact on pregnancy outcome in mouse model. Clin J Obstet Gynecol. 2021;4:33–39. doi: 10.29328/journal.cjog.1001083.
- Shi L, Wang H, Lu Z. Staphylococcal infection and infertility. In: Darwish AM, editor. Genital infections and infertility. Rijeka, Croatia: InTech: 2016. p. 159–175. doi: 10.5772/62663.
- Deng L, Schilcher K, Burcham LR, et al. Identification of key determinants of Staphylococcus aureus vaginal colonization. mBio. 2019;10(6):e02321–19. doi: 10.1128/mBio.02321-19.
- Stock I, Grueger T, Wiedemann B. Natural antibiotic susceptibility of strains of Serratia marcescens and the S. liquefaciens complex: s. liquefaciens sensu stricto, S. proteamaculans and S. grimesii. Int J Antimicrob Agents. 2003;22(1):35–47. doi: 10.1016/s0924-8579(02)00163-2.
- David MZ, Daum RS. Treatment of Staphylococcus aureus infections. Curr Top Microbiol Immunol. 2017;409:325–383. PMID: 28900682. doi: 10.1007/82_2017_42.
- Zivkovic Zaric R, Zaric M, Sekulic M, et al. Antimicrobial treatment of Serratia marcescens invasive infections: systematic review. Antibiotics (Basel). 2023;12(2):367. doi: 10.3390/antibiotics12020367.
- Bilgin H, Sarmis A, Tigen E, et al. Delftia acidovorans: a rare pathogen in immunocompetent and immunocompromised patients. Can J Infect Dis Med Microbiol. 2015;26(5):277–279. doi: 10.1155/2015/973284.
- Højgaard SMM, Rezahosseini O, Knudsen JD, et al. Characteristics and outcomes of patients with Delftia acidovorans infections: a retrospective cohort study. Microbiol Spectr. 2022;10(4):e0032622. doi: 10.1128/spectrum.00326-22.
- Brewster WR, Burkett WC, Ko EM, et al. An evaluation of the microbiota of the upper reproductive tract of women with and without epithelial ovarian cancer. Gynecol Oncol Rep. 2022;42:101017. doi: 10.1016/j.gore.2022.101017.
- Falcone-Dias MF, Vaz-Moreira I, Manaia CM. Bottled mineral water as a potential source of antibiotic resistant bacteria. Water Res. 2012;46(11):3612–3622. doi: 10.1016/j.watres.2012.04.007.
- Passari, Ajit K, Gupta, Vijai K, Singh, Bhim P, Zothanpuia,. Detection of antibiotic-resistant bacteria endowed with antimicrobial activity from a freshwater lake and their phylogenetic affiliation. PeerJ. 2016;4:e2103. doi: 10.7717/peerj.2103.
- Lin S, Zhang B, Lin Y, et al. Dysbiosis of cervical and vaginal microbiota associated with cervical intraepithelial neoplasia. Front Cell Infect Microbiol. 2022;12:767693. doi: 10.3389/fcimb.2022.767693.