Abstract
The content of linalyl acetate and the ratio of linalool to linalyl acetate (L/LA ratio) are one of the important parameters that determine the quality of lavender oil. The characterization of a segregating population derived from a self-pollinated Lavandula angustifolia var. Hemus resulted in the identification of a single quantitative trait locus controlling the L/LA ratio (L/LA-QTL) and located on chromosome 8 of the lavender reference genome. The L/LA data analysis demonstrated that plants homozygous for one of the L/LA-QTL alleles had significantly higher linalool content, lower linalyl acetate content and higher L/LA ratio, than the plants which were either heterozygous or homozygous for the other allele. No significant difference was observed for the sum of linalool and linalyl acetate content among these three groups of plants, suggesting that the identified L/LA-QTL is related to an enzyme conversion of linalool to linalyl acetate. The BLAST search revealed that the L/LA-QTL region included a sequence of the LiAAT4 gene of monoterpene acetyltransferase, considered as a candidate gene for the L/LA-QTL locus. Sequence analysis of the LiAAT4 gene of var. Hemus revealed the presence of two alleles differing in two nucleotides and predicted amino acid substitutions. The comparison of the allele configurations of SSR, SRAP and LiAAT4 loci and the L/LA ratio of plants showing recombination in the L/LA-QTL region provided further support that LiAAT4 is a candidate gene underlying the identified L/LA-QTL and controlling the L/LA ratio. The application of molecular markers for the identified L/LA-QTL is discussed.
Introduction
The industrial cultivation of species from the genus Lavandula is dominated by Lavandula angustifolia (lavender) and L. angustifolia × L. latifolia (lavandin), related to the essential oil production [Citation1–3]. Lavender essential oil has various biological activities and is used in a wide range of products and applications including aromatherapy, cosmetics, food and medicinal products [Citation4–8]. The quality of lavender oil largely depends on the composition and relative content of terpenes and terpenoids, some of which are a subject of national and international quality standards, e.g. the ISO 3515:2002 standard [Citation8,Citation9]. The monoterpene esters such as linalyl acetate, lavandulyl acetate, geranyl acetate and others have been considered to largely contribute to the characteristic aroma and flavor as well to the biological activities of lavender oil [Citation1,Citation8,Citation10]. The finest and most desired lavender oils have high content of linalool and linalyl acetate, and the higher content of linalyl acetate to linalool is preferable [Citation8,Citation11]. Lavender plants biosynthesize linalyl acetate through enzyme acetylation of linalool by the action of alcohol acetyltransferases, part of the monoterpene biosynthesis pathway [Citation8,Citation12–15] (Supporting Information S1). Sarker and Mahmoud [Citation13] proposed that the conversion of linalool to linalyl acetate is carried out by the LiAAT3 and/or the LiAAT4 acetyltransferase, but were not successful at detecting such conversion by purified recombinant LiAAT3 and LiAAT4 proteins. Recently Li et al. [Citation12] reported conversion of linalool to linalyl acetate by purified recombinant La06G01094 protein homologous to LiAAT4.
Since 2014, Bulgaria has been the world largest supplier of lavender oil [Citation2,Citation5,Citation16]. Lavender oil production in the country is based on the industrial cultivation of a small number of superior lavender varieties, all of them having high content of linalool and linalyl acetate and higher relative content of linalyl acetate compared to linalool [Citation9]. Despite the importance of the linalool-to-linalyl-acetate (L/LA) ratio for the quality of the produced lavender oil, the genetic background controlling the L/LA ratio in the cultivated lavender varieties has not been well studied and no molecular markers suitable for marker assisted selection for this trait are currently available.
Here, we report the identification of a QTL controlling the ratio of linalool to linalyl acetate in the segregating population of self-pollinated L. angustifolia var. Hemus and the identification of LiAAT4 as a candidate gene underlying this QTL.
Materials and methods
Plant material
A segregating population obtained by self-pollinating a L. angustifolia var. Hemus plant (AgroBioInstitute accession number: Lva1), described in Vassileva et al. [Citation17], was used in the study. Three-year-old plants cultivated in the experimental field of Agrobioinstitute (Kostinbrod, Bulgaria) were used for collection of leaves and florets samples. Young leaves were collected in plastic tubes, frozen in liquid nitrogen and stored at −80 °C in a deep freezer until DNA extraction. Florets sample collection for GC/MS analysis of flower volatiles was performed as described in Zagorcheva et al. [Citation18]. Floret samples were collected during the 2022 and 2023 growing seasons. Thirty fully open florets at stage 3 according to Guitton et al. [Citation11] were collected in a 10 mL headspace glass vial. After the addition of 3 mL of hexan (Sigma) and 200 mg of anhydrous sodium sulfate (Sigma), the glass vials were tightly capped using a crimp and temporally kept at 4 °C until analysis. Prior to analysis, the extraction of volatile compounds from the floret samples was assisted by vortexing of the vials at 1000 rpm on a multi-tube vortex (Vortex Genius 3, VWR) for 30 min.
GC/MS analysis of flower volatiles
Following the opening of the glass vials, 1 mL of the resulting extract was transferred to a 2 mL GC vial and subjected to GC/MS analysis on an Agilent 7890 A/5975C GC/MS system as described earlier [Citation18]. Briefly, the compounds were separated on a polar HP-INNOWax column (Agilent), 30 m × 0.25 mm, film thickness 0.25 μm, containing PEG as a stationary phase. Carrier gas was helium (purity 99.999%) at a flow rate of 0.8 mL/min. The injector temperature was 250 °C, using a splitless injection of 1 μL per sample. The oven program was as follows: 65 °C to 170 °C at 2 °C/min, then, 60 °C/min to 240 °C, hold for 15 min. The mass selective detector was operated at a transfer line temperature of 250 °C and electron impact ionization voltage of 70 eV. Individual compounds were detected and identified using the AMDIS Ver. 2.73 software (National Institute of Standards and Technology (NIST), Gaithersburg, MD, USA) and a custom-built MS library consisting of compounds identified previously in lavender oils. The ratio of linalool to linalyl acetate was calculated as the ratio of the peak areas of the two compounds, determined by manual integration using the MSD ChemStation (Agilent Technologies, Inc., Santa Clara, CA, USA) software. The relative content of linalool and linalyl acetate as a percentage of the total area of the chromatogram was determined using the same software.
SSR and SRAP analysis
The frozen leaf samples were ground to fine powder using liquid nitrogen and a Qiagen TissueLyser II Mill at 30 Hz for 2 min. Genomic DNA was purified according to the cetyl trimethylammonium bromide (CTAB) protocol [Citation19]. Genomic DNA concentration was measured spectrophotometrically using Nanodrop 2000 (Thermo Fisher Scientific, Waltham, MA, USA) and diluted to a final concentration of 25 ng/μL with ultrapure water. The SSR fragments were amplified in 16 µL reaction containing 1 µL of forward primer (10 pmol) labelled with FAM, 1 µL of reverse primer (10 pmol), 8 µL of 2x MyTaqTM Mix (Bioline), 4.7 µL of ultra-pure water and 1.3 µL of gDNA (25 ng/μL), using the following PCR conditions: 95 °C for 3 min followed by 33 cycles of 95 °C for 15 s, 58 °C for 30 s, 72 °C for 30 s and final elongation at 72 °C for 10 min. The following SSR primers were used, (Supporting Information S2): 123 F 5′-aggatgattgcatcacatactgc, 123 R 5′-agattagcaatgccgcgaac, 257 F 5′-tatctgcaccagccctcttg, 257 R 5′-tccctgaaaagaagcaaaaccc, 339 F 5′-tcaactatgcagtcggaagc, 339 R 5′-actttggagcatcaacgcag, 481 F 5′-tcaataccaggtgaaggttgc, 481 R 5′-cgccccagctagagtttttg. SRAP analysis was performed as described in Zagorcheva et al. [Citation20]. fragments were amplified using the ME4 5′-tgagtccaaaccggacc (5′-FAM labeled) and EM6 5′-gactgcgtacgaattttt primers and the following conditions: 20 µL reaction containing 1 µL of ME4 primer (10 pmol), 1 µL of EM6 primer (10 pmol), 10 µL 2x MyTaqTM Mix (Bioline), 6.5 µL ultrapure water and 1.5 µL gDNA (25 ng/μL), using the following PCR conditions: 5 min at 94 °C; 3x cycles of 1 min at 94 °C, 1 min at 35 °C and 1 min at 72 °C; 35x cycles of 1 min at 94 °C, 1 min at 50 °C and 1 min at 72 °C and a final elongation step of 3 min at 72 °C. Fragment analysis was performed on an automated capillary sequencer 3130 Genetic Analyzer (Thermo Fisher Scientific, Waltham, MA, USA) using 36-cm long capillaries, POP-7 polymer (Thermo Fisher Scientific, Waltham, MA, USA) and GeneScanTM 500 LIZTM (Thermo Fisher Scientific, Waltham, MA, USA) as a size standard. GeneMapper Analysis Software v4.0 (Thermo Fisher Scientific, Waltham, MA, USA) was used for fragment sizing.
Data analysis
The genetic linkage map used in the study was created using JoinMap 5.0 [Citation21] with SSR and SRAP markers as described in Vassileva et al. [Citation17]. QTL analysis was carried out using the interval mapping function of MapQTL 6.0 [Citation22]. Significant QTLs (p < .05) were identified on a genome-wide scale based on a permutation test including 100,000 permutations. Box plots were built using MS Excel 2021. Analysis of variance (ANOVA) was performed using IBM SPSS Statistics 25.
Results and discussion
Identification of QTL controlling the ratio of linalool to linalyl acetate
The content of linalool and linalyl acetate, as well as the L/LA ratio of the content of both compounds are essential parameters that determine the quality of the lavender essential oil. In the present study, QTL analysis employing a segregating population of 95 plants was carried out to identify QTL that control the L/LA ratio in lavender flowers. The segregating population was derived from a self-pollinated plant of the industrially cultivated L. angustifolia var. Hemus, which is one of the most widely cultivated Bulgarian lavender varieties [Citation9]. The population was previously genotyped using 289 SSR and SRAP markers resulting in the construction of a genetic linkage map consisting of 24 linkage groups/LG/, spanning a total of 219,352 cM, as described in details in Vassileva et al. [Citation17]. The volatile composition, relative content of the compounds and ratio of linalool to linalyl acetate was determined for each plant of the population through GC/MS analysis of hexane extracts from fully open florets. The QTL analysis resulted in the identification of a single QTL controlling the L/LA ratio (designated as L/LA-QTL) and located on LG8, (Supporting Information S3) corresponding to chromosome 8 of the recently reported L. angustifolia genome assembly [Citation23]. Further analysis demonstrated that the L/LA-QTL is located in the 6.8 cM region spanning the loci of SSR markers 123 and 481 A on LG8 ().
Figure 1. Mapping of L/LA-QTL and allele configuration of loci in the L/LA-QTL region in the recombinant plants. (a) LOD profile and molecular marker locations in the L/LA-QTL region on LG8 (the results of QTL analysis for all LGs of the L. angustifolia genome are presented in Supporting Information S1); (b) genotypes of plants according to the alleles of SSR, SRAP and ATT4 loci located in the L/LA-QTL region. The plant names correspond to the names of groups of plants from the segregating population which are presented in . The low/high value of the L/LA ratio is indicated for each plant genotype.
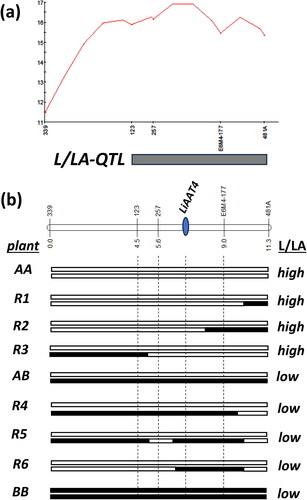
L/LA ratio in plants with different allele combinations of the L/LA-QTL
A total number of 252 plants from the same segregating population were genotyped using the three SSR markers (123, 257 and 481 A) and a SRAP marker (E6M4-177) located in the identified L/LA-QTL region (). Allele analysis of the genotyped plants resulted in the identification of 41 plants homozygous for the ‘A’ alleles of the loci corresponding to all four analyzed markers, 54 plants homozygous for the ‘B’ allele of these loci, as well as 124 plants heterozygous for these loci (). The rest of the 33 plants showed various recombination events at different locations in the L/LA-QTL region, and information on some of them is shown in and . Further analysis of the data for the L/LA ratio of the plants with no detected recombination in the L/LA-QTL region revealed significantly higher values of the L/LA ratio in the plants homozygous for allele ‘A’ of the four loci in comparison to the set of plants homozygous for allele ‘B’ or heterozygous for these loci ( and ). Additionally, the group of plants at heterozygous state had a significantly higher average L/LA ratio than those of the group of plants heterozygous for allele ‘B’ (). Tо understand the reason for the observed differences in the L/LA ratio, the average values of the relative content of linalool and linalyl acetate were calculated for each group and compared. The results demonstrated significantly higher accumulation of linalool in the florets of ‘AA’ homozygous plants than those of heterozygous or ‘BB’ homozygous plants (). On the contrary, the content of linalyl acetate was significantly lower in the group of ‘AA’ homozygous plants than in the other two groups. At the same time, no significant difference in the total content of linalool and linalyl acetate taken together was observed for the three studied groups of plants (). The obtained results are well explained by the hypothesis that L/LA-QTL is related to the enzymatic conversion of linalool to linalyl acetate. Accordingly, the L. angustifolia var. Hemus plant used for the establishment of the segregating population has two alleles in the L/LA-QTL: allele ‘A’ related to lower rate of linalool to linalyl acetate conversion and allele ‘B’ providing high rate of linalyl acetate production. Thus, in the plants of the ‘AA’ group, linalool is converted at a significantly lower rate to linalyl acetate, resulting in much higher L/LA ratio compared to the plants of the other two groups. Correspondingly, the presence of a functional ‘B’ allele in the heterozygous plants resulted in production of higher content of linalyl acetate and reduction of the L/LA ratio. Furthermore, the presence of two ‘B’ alleles in the ‘BB’ plants additionally provide the highest rate of linalool to linalyl acetate conversion, highest level of linalyl acetate accumulation and lowest L/LA ratio among these three groups of plants.
Figure 2. Box and whiskers plot showing the distribution of data for the L/LA ratio among groups of plants with different zygosity state for the L/LA-QTL region.
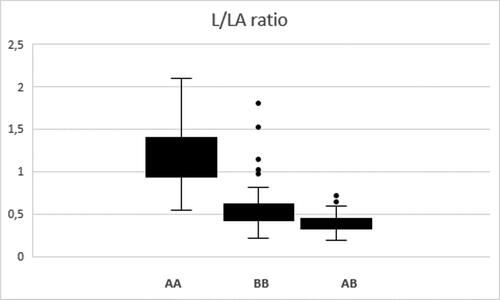
Table 1. Allele configurations of loci located in the identified L/LA-QTL region, L/LA ratio, relative contents of linalool (L) and linalyl acetate (LA) and total relative content of the sum of linalool and linalyl acetate (L + LA). The names of groups of plants with the same genotypes for the analyzed loci in the L/LA-QTL region are shown in bold.
LiAAT-4 is a candidate gene underlying the L/LA-QTL
The ratio of linalool to linalyl acetate in plants depends on the rate of enzymatic conversion of linalool to linalyl acetate [Citation8,Citation10,Citation15]. Sarker and Mahmoud [Citation13] reported the cloning and functional characterization of two monoterpene acetyltransferase genes (LiAAT3 and LiAAT4) associated with the efficient production of geranyl acetate, lavandulyl acetate and neryl acetate from their respective monoterpene substrates, but the purified recombinant protein did not convert linalool to linalyl acetate. Recently, the study by Li et al. [Citation12] reported the enzyme activity associated with the La14G01394 and La06G01094 genes from L. angustifolia, which are homologous to the LiAAT3 and LiAAT4 genes. The results demonstrated that the purified recombinant La06G01094 protein successfully converts linalool to linalyl acetate [Citation12].
Searching for a candidate gene underlying the L/LA-QTL, we carried out a BLAST search of the chromosome 8 sequence of the reference lavender genome using reported sequences for alcohol acetyltransferases, including those of LiAAT3 and LiAAT4. The result of the search demonstrated the presence of a sequence homologous to the LiAAT4 sequence in the L/LA-QTL region of chromosome 8 (Supporting Information S4). Further PCR amplification and sequence analysis of the LiAAT4 region in var. Hemus revealed that the LiAAT4 gene has two alleles differing in two nucleotides (Supporting Information S4). The nucleotide sequence of one of the LiAAT4 alleles (designated as ‘LiAAT4(B)’) of var. Hemus is identical to the LiAAT4 sequence reported by Sarker and Mahmoud [Citation13]. The other allele (designated as ‘LiAAT4(A)’) differs in two nucleotide substitutions in the coding region, resulting in predicted A258>V and A296>T substitutions in the respective LiAAT4 amino acid sequence. The two identified SNPs were further used to determine the LiAAT4 allele configurations of the analyzed plants from the studied segregating population. The allele combinations of the applied SSR and SRAP markers and the LiAAT4 gene for each plant were compared with the respective L/LA ratio. The results demonstrate that plants with a high L/LA ratio are homozygous for the LiAAT4(A) allele, whereas the heterozygous LiAAT4(AB) or homozygous LiAAT4(B) plants show a lower L/LA ratio related to higher rate of conversion of linalool to linalyl acetate. The possible involvement of the LiAAT4(B) allele into the observed higher level of conversion of linalool to linalyl acetate was further supported by the subsequent comparison of the L/LA ratio and the allele configurations of six groups of L/LA-QTL recombinant plants from the segregating population. The genotypes of these plants for the studied SSR, SRAP and ATT4 loci were the result of recombinations within the L/LA-QTL genomic region (). The results demonstrate that higher levels of conversion of linalool to linalyl acetate are always associated with the presence of at least one LiAAT4(B) allele, but not with B alleles of the sub-regions in the L/LA-QTL, which flank the LiAAT4 locus (plants R4-R6 in ). On the contrary, low linalyl acetate production and high L/LA ratio was observed in the R1-R3 plants which were homozygous for the LiAAT4(A) allele, despite the presence of ‘B’ alleles of some of the flanking regions (). On the other hand, the results from the present study show that the plants homozygous for the LiAAT4(A) allele still produce and accumulate linalyl acetate as a result of a lower rate conversion of linalool to linalyl acetate. The cause for the latter is not clear and could be a result of the detected amino acid substitutions and/or changes in the expression of the LiAAT4(A) allele, or a result of the expression of other alcohol acetyltransferase genes in the L. angustifolia genome.
The high linalyl acetate content and optimal L/LA ratio is a very important trait for the industrially cultivated lavender varieties to ensure high quality of the produced essential oil. Accordingly, the results of the present study provide useful molecular markers for marker-assisted selection in lavender breeding. The markers could be efficiently applied to select plants homozygous for the LiAAT4 alleles providing a high rate of linalyl acetate production in the newly developed varieties. During the last decade, the increasing demands of lavender flower and essential oil production resulted in the expansion of lavender cultivation in new non-traditional agro-ecological regions [Citation9]. This, together with the climate changes and the specific agro-ecological conditions occasionally leads to undesirable changes of the linalyl acetate content, L/LA ratio and quality of the produced lavender oil [Citation24–26].
The results of the present study demonstrate that the allele state of the LiAAT4 gene could have a significant impact on the linalyl acetate content of the plant. Although some of the reported studies point out variations in the linalyl acetate content due to environmental factors, their impact on the different LiAAT4 alleles remain unclear. Thus, studying the allele diversity of the LiAAT4 gene in the current lavender varieties and genetic resources as well as evaluation of the impact of the environmental conditions on the expression of the different LiAAT4 alleles could be beneficial for lavender breeding related to essential oil production.
Conclusions
QTL analysis of а segregating population from а self-pollinated plant of the industrially cultivated L. angustifolia var. Hemus resulted in identification of a single L/LA-QTL controlling the conversion of linalool to linalyl acetate and the L/LA ratio between these two compounds. The search of genomic sequence corresponding to the identified L/LA-QTL region resulted in the identification of LiAAT4 as a candidate gene underlying the L/LA-QTL. The study of recombinant plants within the L/LA-QTL region supports the identified LiAAT4 candidate gene. The obtained metabolite data suggest that one of the alleles of the L/LA-QTL and LiAAT4 is related to a lower rate of linalool to linalyl acetate conversion. The obtained molecular marker and allele data could be efficiently used for marker assisted selection in lavender breeding.
Authors’ contributions
Conceptualization, I.A. and K.R.; Methodology, I.A., K.R., M.R. and P.V.; Software, K.R., P.V. and I.A.; Investigation, P.V.; Resources, I.A.; Writing, I.A. and K.R.; Funding Acquisition, I.A. All authors have read and approved the final version of the article.
Supplemental Material
Download Zip (3.4 MB)Acknowledgment
The authors would like to thank Rumyana Velcheva (AgroBioInstitute, Sofia, Bulgaria) for the excellent technical assistance.
Disclosure statement
No potential conflict of interest was reported by the authors.
Data availability statement
All data included in this study are available from the corresponding author [I.A.] upon request.
Additional information
Funding
References
- Habán M, Korczyk-Szabó J, Čerteková S, et al. Lavandula species, their bioactive phytochemicals, and their biosynthetic regulation. Int J Mol Sci. 2023;24(10):1. doi: 10.3390/ijms24108831.
- Lesage-Meessen L, Bou M, Sigoillot J-C, et al. Essential oils and distilled straws of lavender and lavandin: a review of current use and potential application in white biotechnology. Appl Microbiol Biotechnol. 2015;99(8):3375–7. doi: 10.1007/s00253-015-6511-7.
- Lis-Balchin M. Lavender: the genus Lavandula. London (UK): CRC Press; 2002.
- Diass K, Merzouki M, Elfazazi K, et al. Essential oil of Lavandula officinalis: chemical composition and antibacterial activities. Plants. 2023;12(7):1571. doi: 10.3390/plants12071571.
- Kozuharova E, Simeonov V, Batovska D, et al. Chemical composition and comparative analysis of lavender essential oil samples from Bulgaria in relation to the pharmacological effects. PHAR. 2023;70(2):395–403. doi: 10.3897/pharmacia.70.e104404.
- Malloggi E, Menicucci D, Cesari V, et al. Lavender aromatherapy: a systematic review from essential oil quality and administration methods to cognitive enhancing effects. Appl Psychol Health Well Being. 2022;14(2):663–690. doi: 10.1111/aphw.12310.
- Wells R, Truong F, Adal AM, et al. Lavandula essential oils: a current review of applications in medicinal, food, and cosmetic industries of lavender. Nat Prod Commun. 2018;13(10):1934578X1801301. doi: 10.1177/1934578X1801301038.
- Woronuk G, Demissie Z, Rheault M, et al. Biosynthesis and therapeutic properties of Lavandula essential oil constituents. Planta Med. 2011;77(1):7–15. doi: 10.1055/s-0030-1250136.
- Stanev S, Zagorcheva T, Atanassov I. Lavender cultivation in Bulgaria-21st century developments, breeding challenges and opportunities. Bulg J Agric Sci. 2016;22(4).
- Fontez M, Bony A, Nicole F, et al. Lavandula angustifolia Mill. a model of aromatic and medicinal plant to study volatile organic compounds synthesis, evolution and ecological functions. Bot Lett. 2023;170(1):65–76. doi: 10.1080/23818107.2022.2154839.
- Guitton Y, Nicolè F, Moja S, et al. Differential accumulation of volatile terpene and terpene synthase mRNAs during lavender (Lavandula angustifolia and L. x intermedia) inflorescence development. Physiol Plant. 2010;138(2):150–163. doi: 10.1111/j.1399-3054.2009.01315.x.
- Li J, Li H, Wang Y, et al. Decoupling subgenomes within hybrid lavandin provide new insights into speciation and monoterpenoid diversification of Lavandula. Plant Biotechnol J. 2023;21(10):2084–2099. doi: 10.1111/pbi.14115.
- Sarker LS, Mahmoud SS. Cloning and functional characterization of two monoterpene acetyltransferases from glandular trichomes of L. x intermedia. Planta. 2015;242(3):709–719. doi: 10.1007/s00425-015-2325-1.
- Seira E, Poulaki S, Hassiotis C, et al. Gene expression of monoterpene synthases is affected rhythmically during the day in Lavandula angustifolia flowers. Physiologia. 2023;3(3):433–441. doi: 10.3390/physiologia3030030.
- Zaks A, Davidovich-Rikanati R, Bar E, et al. Biosynthesis of linalyl acetate and other terpenes in lemon mint (Mentha aquatica var. citrata, Lamiaceae) glandular trichomes. Isr J Plant Sci. 2008;56(3):233–244. doi: 10.1560/IJPS.56.3.233.
- Giray FH. An analysis of world lavender oil markets and lessons for Turkey. J Essent Oil-Bear Plants. 2018;21(6):1612–1623. doi: 10.1080/0972060X.2019.1574612.
- Vassileva P, Rusanov K, Rusanova M, et al. Development of SSR markers and construction of a genetic linkage map in lavender (Lavandula angustifolia Mill. Plants. 2023.
- Zagorcheva T, Rusanov K, Stanev S, et al. A simple procedure for comparative GC-MS analysis of lavender (Lavandula angustifolia Mill.) flower volatile composition. IOSR. 2016;11(04):09–14. doi: 10.9790/3008-1104030914.
- Murray M, Thompson W. Rapid isolation of high molecular weight plant DNA. Nucleic Acids Res. 1980;8(19):4321–4325. doi: 10.1093/nar/8.19.4321.
- Zagorcheva T, Stanev S, Rusanov K, et al. SRAP markers for genetic diversity assessment of lavender (Lavandula angustifolia Mill.) varieties and breeding lines. Biotechnol Biotechnol Equip. 2020;34(1):303–308. doi: 10.1080/13102818.2020.1742788.
- Van Ooijen JW. JoinMap 5: software for the calculation of genetic linkage maps in experimental populations. Wageningen, The Netherlands: Kyazma B. V.; 2006.
- Van Ooijen JW. MapQTL 6: software for the mapping of quantitative trait loci in experimental populations of diploid species. Wageningen, The Netherlands: Kyazma B. V.; 2006.
- UGA_lav_v1 L a g a. Available from: https://www.ncbi.nlm.nih.gov/datasets/genome/GCA_028984105.1/. 2023
- Ashraf A, Sultan P, Qazi P, et al. Approaches for the genetic improvement of lavender: a short review. J Pharmacogn Phytochem. 2019;8(2):736–740.
- Crișan I, Ona A, Vârban D, et al. Current trends for lavender (Lavandula angustifolia Mill.) crops and products with emphasis on essential oil quality. Plants. 2023;12(2):357. doi: 10.3390/plants12020357.
- Détár E, Németh ÉZ, Gosztola B, et al. Effects of variety and growth year on the essential oil properties of lavender (Lavandula angustifolia Mill.) and lavandin (Lavandula x intermedia emeric ex loisel.). Biochem Syst Ecol. 2020;90:104020. doi: 10.1016/j.bse.2020.104020.