Abstract
Rhizosphere microorganisms are important for maintaining soil and plant health and promoting plant growth and development. Screening rhizosphere growth-promoting bacteria with unique habitats, high activity and multiple functions is conducive to exploring the potential stress resistance of growth-promoting bacteria, and further improving the applicability of microbial fertilizers in agricultural production in response to the development concept of green agriculture. The aim of this study was to investigate the physiological activity of the culturable core growth-promoting bacteria of Dicranopteris dichotoma and verify their growth-promoting effect on mung bean (Vigna radiata) growth. A total of 41 bacterial strains were isolated in this study; 21 strains had more than one physiological activity, among which the highest siderophores units (SU) were 97.1%, and the highest IAA yield was 134.46 mg/L. According to morphology and 16S rRNA, three strains of Bacillus sp. and one strain of Pantoea sp. were screened, all of which were predicted to have the functions of phosphorous solubilization, cellulase production, IAA production and siderophores production. In a pot experiment, these four strains were inoculated in the rhizosphere soil every 7 days for a total of 4 times, and the aerial and underground biomass, plant height, root length and leaf number of V. radiata plants increased significantly 30 days after the last inoculation. The strains isolated in this study have excellent plant growth promoting (PGP) properties and were expected to be used as a new biological inoculant in practical production.
Introduction
At present, chemical fertilizers and other artificially produced chemical agents find long-term and large-scale application for enhancement of crop growth and soil quality, which has threatened the soil ecological balance [Citation1–3]. The rhizosphere is a critical area for interaction betwen plant roots and soil microorganisms [Citation4, Citation5]. Rhizosphere soils have higher metabolite contents and higher microbial biodiversity (a diverse and robust microbial community), helping to maintain soil fertility and plant health [Citation6, Citation7]. Plant growth-promoting rhizobacteria (PGPR) can improve plant growth or help plants overcome biotic or abiotic stress [Citation8]. PGPRs can fix nitrogen [Citation9], dissolve phosphorus [Citation10], release potassium [Citation11], produce plant hormones [Citation12], secrete ACC (1-aminocyclopropane-1-carboxylate) deaminase or secrete antibiotics [Citation13], thereby promoting plant growth. Two substances that have a great influence on plants are IAA and siderophores. IAA is the earliest plant growth hormone, which can regulate stem growth and root differentiation. Siderophores are a low-molecular weight organic compounds produced by microorganisms. Thanks to its high affinity for Fe3+, siderophores can be absorbed from insoluble minerals and released to plants for utilization. Currently, most high-yielding siderophores microorganisms are bacteria, such as Bacillus subtilis, Herbaspirillum sp. [Citation14], Klebsiella pneumoniae [Citation15], etc. Due to their great importance in enhancing and maintaining soil nutrients in soil ecosystems, PGPR strains have received a great deal of attention in the agricultural sector. PGPR strains are widely used as biofertilizers or biological inoculants [Citation16]. They improve soil quality and significantly increase crop productivity while reducing the use of synthetic fertilizers and agrochemicals, enabling sustainable and environmentally friendly farming practices [Citation17].
For example, various strains of bacteria with growth-promoting abilities were present in the rhizosphere of wild quinoa [Citation18]. These bacteria were observed to elongate both the plant stem and root, increasing up to 1.5 times in plant height. Additionally, the yield of the plants was enhanced and there was a change in their physiological shape, including an increase in transpiration rate. Another study [Citation19] also confirmed the promotion effect of PGPR inoculation on chlorophyll content and antioxidant activity of plants. Rhizosphere growth-promoting bacteria, especially Bacillus sp., have inhibitory effects on various plant pathogens, including inducing plants to produce antibacterial substances and competing with pathogens for growth [Citation20, Citation21]. The strains isolated from the rhizosphere of plants in different environments have significant differences in functions. For example, most of the strains isolated from the rhizosphere of pepper plants in water-scarce areas have various functions such as drought resistance, salt tolerance and heat resistance [Citation22]. Strains isolated near the rhizosphere of native plants growing on iron minerals that could tolerate high concentrations of arsenic pollution and promote plant growth in arsenic-contaminated soil [Citation23]. All the above studies have proved that rhizosphere growth-promoting bacteria play an important role in promoting plant growth and increasing crop yield.
In addition, the evidence to date also indicates that the recruitment and functional evolution of rhizosphere microorganisms are closely related to plant genotypes [Citation24] and root exudates [Citation25]. Bourak et al. [Citation26] have also observed that PGPR can be beneficial to shaping a robust plant’s phenotype. Thus, it is of great significance to isolate rhizosphere growth-promoting bacteria from plants with strong resistance. Continued research in those underexplored and unexplored plant rhizosphere environments may lead to the discovery of multiple important new PGPR strains.
Dicranopteris dichotoma (Thunb.) Bernh. is a pioneer and ancient species of the fern genus Dicranopteris of the family Gleicheniaceae (Filicopsida), which is a widespread species in tropical and subtropical regions in East Asia and identified as an indicator plant of acid red soil habitat [Citation27]. The underground part (underground stems and adventitious roots) may grow buried in soil or close to the surface, which makes it play an important role in the ecological restoration of degraded ecosystems [Citation28]. The study also showed that the increase in soil organic matter and nutrient content during community succession of D. dichotoma may be related to the diversity of rhizosphere soil bacteria and the increase in the abundance of certain bacteria genera such as Actinomyces sp. and Proteobacteria sp. [Citation29]. However, few studies have investigated the plant growth-promoting microorganisms associated with D. dichotoma and their contribution to effectively assist host plant growth and enhance resistance to nutrient deficiency stress. In the present study, the rhizosphere microorganisms were isolated from the naturally growing D. dichotoma in red soil environment of Jiangxi province, southern China. Then, the isolates were identified based on sequence phylogenetic reconstruction analysis and their plant growth promoting characteristics were also determined and assessed. Our studies indicated that the robust and multitrait plant growth-promoting rhizosphere microorganisms isolated from the pioneer plant D. dichotoma have potential application values as a biofertilizer.
Materials and methods
Samples collection and isolation of rhizobacteria
Soil samples were collected from the rhizosphere of D. dichotoma growing in the Yiyang City, Jiangxi Province in China in April 2020. The plant’s roots were excised with rhizosphere soil by digging out intact root systems and were carefully taken in plastic zip bags stored at 4 °C. The surface organic matter was removed and brought to the laboratory, and was processed within 24-48 h. Rhizosphere bacteria were isolated from the soil sample tightly adhered to the root by serial dilution plating on Luria-Bertani (LB) agar plates, the plates of each sample were incubated at 37 °C ± 1 °C till the appearance of bacterial colonies. After the appearance of robust colonies, individual colonies were picked up and streaked on fresh LB plates for further purification. This was repeated several times, after which purified colonies were obtained. The selected colonies were further cultured in LB broth and preserved with 65% glycerol at −80 °C for further analysis.
Plant growth promotion characteristics of isolated rhizobacteria in vitro
Evaluation of phosphate solubilization capability of rhizobacterial isolates
The phosphate-solubilizing ability of the rhizosphere bacteria was preliminary screened by inoculating the isolates on Pikovskaya medium plates [Citation30] containing (g/L): glucose 10, (NH4)2SO4 0.5, NaCl 0.3, MgSO4·7H2O 0.3, KCl 0.3, FeSO4·7H2O 0.03, MnSO4·H2O 0.03, Ca3PO4 5 and agar 18, with incubation at 30 °C ± 2 °C for 7 d. The presence of visible clear halos around the bacteria colonies was used to assess phosphorus solubilization. Phosphate solubilization index (PSI) was calculated using the formula according to Singh et al. [Citation31]. PSI = total diameter (colony + halo)/diameter of colony.
Phosphorus standard curve: The standard phosphorus solution (KH2PO4, Solarbio, Cat#BYP1020) was diluted to 0, 0.2, 0.4, 0.6, 0.8, 1.0 mg/L phosphorus solutions. The content of phosphorus was determined by the Mo-Sb anti spectrophotometric method: 5 mL phosphorus solutions of different concentrations were added into 50 mL bottles and 25 mL deionized water was added. Then, 1-2 drops of 2,4-dinitrophenol were dropped until the solution was slightly yellow, and a drop of 0.1M H2SO4 was added until the solution was clear and transparent. Then, 5 mL of Molybdenum antimony anti chromogenic agent were added and the volume was brought up to 50 mL [The molybdenum antimony anti chromogenic agent was prepared as follows: 0.5 g of C8H8KO12Sb was dissolved in 100 mL of water to prepare liquid reagent A, 10 g of (NH4)6Mo7O24·4H2O was dissolved in 300 mL of water at 60 °C and cooled to prepare liquid reagent B; 126 mL of H2SO4 were slowly poured into 400 mL water to prepare liquid reagent C, then reagent C was slowly poured into reagent B, stirred well and poured into reagent A. After cooling, the volume was brought up to 1 L with deionized water to obtain liquid reagent D, and was stored in a brown bottle at room temperature. Then, 1.5 mL of L-ascorbic acid was dissolved in 100 mL of reagent D to form the molybdenum antimony anti chromogenic agent]. After color development at room temperature for two hours, the absorption values were measured at 700 nm (OD700) with the Molybdenum antimony anti chromogenic agent as the blank control, and the standard phosphorus concentration curve was drawn.
The strains that exhibited phosphate-solubilizing halo were selected and inoculated into LB liquid medium (60 mL medium per 250-mL flask) and were cultured in a shaker incubator at 180 rpm for 12h. The cell density was adjusted to OD600 of 0.6, and 200 μL of culture was inoculated in 60 mL Pikovskaya medium for culture for 7d in shaker incubator at 220 rpm. The content of soluble phosphorus (Mo-Sb anti spectrophotometric method) and pH value (SevenDirect SD20 pH meter) in culture supernatant were measured daily; the measurements were repeated three times to take the average value.
Screening for indole acetic acid production
The indole-3-acetic acid (IAA) production by rhizosphere bacteria was screened and measured using a colorimetric assay based on the method of Glickmann and Dessaux [Citation32]. Briefly, a loopful of inoculum was removed from the stock and inoculated into 250 mL flask containing 60 mL King’B liquid medium (containing 2.0% (w/v) peptone, 0.15% (w/v) K2HPO4, 0.15% (w/v) MgSO4·7H2O, 1.5% (v/v) glycerol) supplemented with 0.05% L-tryptophan (w/v), which was incubated in an incubator shaker at 200 rpm and 28 °C ± 2 °C for 3 d. The resulting broth sample was centrifuged for 10 min at 10,000 rpm at room temperature and the cell-free supernatant was collected and mixed with 1 mL of PC reagent (containing 1.2% (w/v) FeCl3 and 7.9 mol/L H2SO4). The reaction mixture was incubated at 30 °C for 30 min before determining the absorbance at 530 nm using a spectrophotometer (METASH X-6). The IAA standard solutions with concentrations ranging from 10 to 100 μg/mL were used to construct a calibration curve.
Siderophores production
The ability of rhizosphere bacteria isolates to produce siderophores was screened and measured using MKB medium blue plates (containing 0.2% (w/v) casamino acid, 1.5% (v/v) glycerol, 0.25% (w/v) K2HPO4, 0.25% (w/v) MgSO4·7H2O) supplemented with chrome azurol sulphonate (CAS) solution based on the method of Schwyn and Neilands [Citation33]. The plates were incubated at 30 °C for 2 d, and the presence of a bright zone with a yellowish color halo surrounding the colonies in the dark blue medium indicated siderophore production.
To determine the siderophores yield of D. dichotoma rhizosphere bacteria, the strains were inoculated in MKB medium and were grown at 28 °C and 150 rpm for 48 h. The resulting broth sample was centrifuged, and 1 mL of the cell-free supernatant was collected and mixed with the same amount of CAS reagent. After incubation at room temperature in the dark for 60 min, the optical densities were measured at 630 nm with a spectrophotometer. The siderophore unit (SU) was calculated using the formula [Citation34]: SU = [(absorbance of control broth) - (absorbance of sample)/(absorbance of control broth)] 100%.
Test for cellulase enzyme activity
The ability of rhizosphere bacteria isolates to secrete extracellular cellulase enzymes was identified according to the method of James et al. [Citation35] by using carboxymethylcellulose (CMC) agar plates containing (g/L): CMC-Na 10, K2HPO4 1, NaNO3 1, CaCl2 0.02, MgSO4·7H2O 0.2, FeCl3·6H2O 0.05 and agar 18, which were incubated at 28 °C for 3 d. The presence of visible clear halos around the colonies was considered to show cellulase activity after addition of 1% congo red solution for 1h and overlaid with 1 mol/L NaCl for elution 30 min. Extracellular enzymes index (EI) was calculated using the formula: EI = total diameter (colony + halo)/diameter of the colony.
Molecular identification of the rhizobacterial strains
Four rhizobacterial strains of interest were selected based on bioactivity tests. The four strains transferred to LB medium and were incubated at 37 °C for 24 h at 200 rpm. The total genomic DNA was extracted using the Bacterial DNA Kit (Omega Bio-Tek, D3350) according to the manufacturer’s instructions. The primers 27 F (5′-AGAGTTTGATCACTGGCTAG-3′) and 1492 R (5′-CGGCTTACCTTGTTACGACTT-3′) were used for amplification of the 16S rDNA gene using polymerase chain reaction (PCR) [Citation36]. Each reaction (50 µL) consisted of ddH2O 19 μL, 2 × Bio Taq PCR Mix (Taq DNA Polymerase, Mg2+, dNTPs) 25 μL (Bichem, BKM-PCR), DNA template 2 μL, 27 F and 1492 R primers are 2 μL each. The reactions were performed with an initial denaturation at 94 °C for 10 min, 35 cycles consisting of 94 °C for 30 s, 55 °C for 30 s, and 72 °C for 1 min, and a final extension at 72 °C for 10 min using a thermal cycler (ETC811). The PCR amplification products were then checked by gel electrophoresis and the successful PCR products were sent to the Sangon Biotech (Shanghai) Co., Ltd. (https://www.sangon.com) for purification and sequencing. The resulting rhizobacterial 16S rRNA gene sequences were matched against the nucleotide database using the Basic Local Alignment Search Tool (BLAST) in the National Center for Biotechnology Information (NCBI) database. The rhizobacterial strains and their relevant sequences derived from GenBank were aligned with the ClustalW method implemented in MEGA software (version 7.0). The phylogenetic tree was constructed using the neighbor-joining (NJ) method with 1,000 bootstrapped replications to estimate evolutionary distances. The 16S rRNA gene sequences of the PGPR isolates were deposited in NCBI GenBank and obtained accession numbers (see below).
Bacterial culture preparation, seed sterilization, and bacteria inoculation into seedlings
For culture preparation, the obtained multitrait PGPR strains were selected on the basis of siderophore production, IAA production, cellulase production and phosphorus solubilization. The strains of interest were cultured in 250 mL flasks containing 80 mL LB medium on an incubator shaker at 200 rpm and 37 °C for 36 h. The broth containing the strain was harvested by centrifugation at 8000 rpm at 4 °C (High speed table refrigerated centrifuge, CENCE, TGL-16M) for 10 min to separate bacterial cells. The cell pellets were washed twice with sterile distilled water and resuspended in sterile water to obtain the required rhizobacterial density of 106 cells/mL.
Then, a pot experiment was conducted to examine the germination promotion effect of rhizobacteria isolates on Vigna radiata (V. radiata) seeds (Lvbao mung bean king). Surface sterilization of the V. radiata seeds was carried out as described by Thakur et al. [Citation37] with minor modification by washing in 75% ethanol for 2 min, 0.1% mercuric chloride (v/v) for 5 min, respectively. The seed samples were then rinsed 3-4 times in sterile distilled water and dipped in the above rhizobacterial suspension broth for 12 h, then, the seeds were placed on Petri plates containing layers of moist filter paper. The seeds were then allowed to germinate at 30 °C. The appearance of a 2-5 mm radicle was considered an initiation of seed germination.
The germinated seeds were cultured for 6 days until they grew to the two-leaf stage, and the seedlings with the same growth stage were transplanted into peat soil for pot experiments to study the promotion effect of rhizobacterium isolates on the growth parameters of V. radiata seedlings. The size of the pots was 7 × 7 cm in the upper diameter, 5 × 5 cm in the lower diameter, height of 7.3 cm; with 100 g of soil per pot, and one plant per pot. The V. radiata plants were inoculated with 1.5 mL per plant of PGPR bacterial resuspension (at a cell density of 106 cells/mL) once every 7 days near the roots, and they were watered every 2 days. The plants underwent four times of inoculation, and the physiological indices were measured 2 days after the final inoculation. The plants were grown in a greenhouse at 28 °C with 16 h daytime and 8 h nighttime under 40% humidity. For the control treatment (CK) plastic pots were mock inoculated with sterile distilled water, and three pots were the three replicates for each above-described experimental condition. Representative plants were photographed and corresponding indices such as plant height, fresh plant weight, dry weight (electric blast drying oven TAISITE WGL-230B, 80 °C for 18h), fresh root weight, dry root weight, leaf number and root length, were measured and weighed at 36 days post-treatment, respectively.
Statistical analysis
Analysis of variance (ANOVA) (p < 0.05) was applied to evaluate the differences of strain inoculation on V. radiata growth. All the statistical procedures were performed using SPSS v. 27 and Origin v.2022 software.
Results
Isolation of rhizobacterial isolates and estimation with several PGP traits
A total of 41 isolates were isolated and purified from the rhizosphere of D. dichotoma. Then, the isolates were selected for further evaluation of plant growth-promoting traits such as phosphate solubilization capability, siderophores and IAA production, cellulase activity. Our findings highlight that the rhizosphere of D. dichotoma harbors a rich and fascinating PGPR community. Out of 41 rhizobacterial isolates, 21 rhizobacterial isolates were found positive for more than one plant-promoting trait, which includes solubilization of phosphate, production of IAA, siderophore and cellulase (). A total of 17 rhizobacterial isolates formed visible clear phosphate solubilization halos around the bacterial colonies in Pikovskaya medium plates, with the phosphate solubilization index (PSI) in the range of 1.11 to 2.19. The largest phosphate solubilization zone was shown by isolate MQB11 () followed by MQB8, and the PSI of six rhizobacterial isolates (MQB7、MQB 8、MQB10、MQB11、MQB12、MQB13) showed more than 1.5.
Figure 1. Plant growth promotion characteristics of isolated rhizobacteria in vitro. (A) Isolate samples showing cellulase production; (B) Isolate samples showing siderophore production; (C) Isolate samples showing phosphate solubilization. A clear halo around the bacterial colony indicates positive results; (D) In vitro colorimetric determination of IAA and indole compounds in bacterial supernatants. A pink color indicates positive reaction; (E) Phosphorus standard curve; (F) Phosphorus soluble content and pH value of four strains; (G) Determination of siderophore in bacterial supernatant. The pink color of the solution indicates that the siderophore content is higher, and the blue color is the opposite.
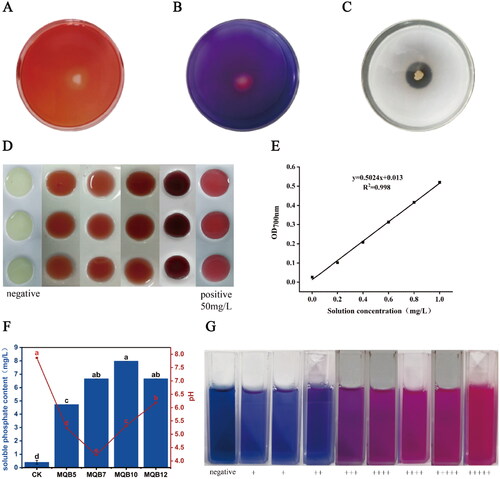
Table 1. Results of plant growth promotion activity test of bacterial isolates.
Four strains MQB5, MQB7, MQB10 and MQB12 were selected to determine the phosphorus soluble content of the strain according to the results of the physiological activity of the strains. The OD700 value of the bacterial supernatant was determined by the Mo-Sb colorimetric method, and the soluble phosphorus content was calculated on the basis of the phosphorus standard curve (). The results showed that MQB10 was the strain with the highest soluble phosphorus content (), reaching 7.98 mg/L, followed by MQB12, MQB7, and MQB5, with soluble phosphorus content of 6.66 mg/L, 6.65 mg/L and 4.74 mg/L, respectively. The soluble phosphorus content of the uninoculated control group was 0.39 mg/L. The soluble phosphorus content was significantly increased by 20.23 times, 16.89 times, 16.88 times and 12 times compared with the control, respectively. At the same time, the change in the pH value of the supernatant also indicated that the inoculation of phosphate-solubilizing bacteria (PSB), reduced the pH of the supernatant compared with the control.
The IAA production was determined in vitro and the pink color in rhizosphere bacterial culture supernatant indicated that the isolate had the capacity for IAA production (). In the quantitative analysis of IAA production, the results indicated that 21 of the 41 isolates could produce IAA. Among these isolates, MQB1 and MQB2, MQB3, MQB6, MQB7, MQB12 and MQB8 showed IAA production above 40 mg/L after inoculation for 96 h. The maximum IAA production was observed in isolate MQB7 with 134.46 mg/L.
The development of orange-yellow halos surrounding the rhizosphere bacterial colonies verified and identified siderophore production (). Our results indicated that 16 of the 41 isolates showed production of orange halos, indicating siderophore production () and among these isolates, MQB2, MQB1 and MQB4 showed strong siderophore production (SU of 97.1%, 95.9%, 87.4%), while isolates MQB5 and MQB12 showed moderate siderophore production (SU of 67.9%, 60.7%) and isolates MQB7 and MQB8 demonstrated mild siderophore production (SU of 7.8%, 9.5%).
To investigate the ability of rhizosphere bacteria isolates to secrete extracellular enzymes, CMC agar plates were used, and the results revealed that only 4 of the 41 isolates (MQB5, MQB7, MQB10, and MQB12) showed the production of halos indicating cellulase production (). Among these isolates, MQB5, MQB7, MQB10 and MQB12 had an extracellular enzyme index (EI) in the range of 1.58 to 2.67. Based on the above PGP functional verification, all 4 strains showed good biological activity. Therefore, the isolates with various PGPR functions were selected for molecular biological identification and plant pot growth promotion experiments.
Molecular identification of selected PGPR isolates
In order to identify the four strains of multifunctional PGPR, we sequenced the 16S rRNA genes of these four strains, and compared them with the 16S rDNA sequences of reference strains in the NCBI database to construct a phylogenetic tree. shows the taxonomic assignation of the strains based on BLAST analysis of 16S rRNA gene sequences. Our results revealed that the rhizobacterial isolates MQB5, MQB12, MQB7 and MQB10 showed high level of sequence identity with Bacillus pumilus, Bacillus aryabhattai, Pantoea agglomerans and Bacillus velezensis, respectively. The maximum identities for each isolate exceeded 99% with an E-value of 0. Furthermore, the neighbor-joining phylogenetic analysis originates from the multiple alignment of 16S rRNA gene sequence of PGPR strains with those of other closely related strains existing in the GenBank database. The phylogenetic tree also revealed a high homology and formed clades with their respective strain 16S rRNA gene sequence (). Therefore, based on 16S rDNA gene sequence phylogenetic analysis, the isolates MQB5, MQB12, MQB7, MQB10 were identified and named as B. aryabhattai MQB12, B. pumilus MQB5, B. velezensis MQB10 and P. agglomerans MQB7, respectively.
Figure 2. Neighbor-joining phylogenetic analysis originating from the multiple alignments of 16S rRNA gene sequence of PGPR strains with those of other closely related strains existing in the GenBank database.
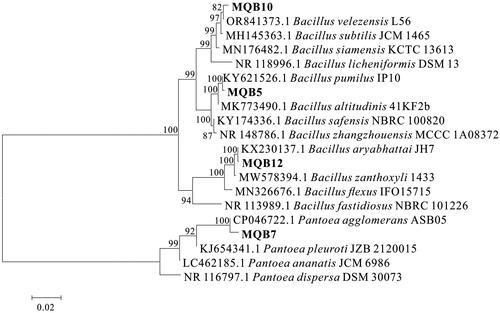
Table 2. Molecular identification of rhizobacteria based on 16S rRNA gene sequences.
Effects of selected PGPR isolates on the growth of V. radiata
We evaluated the growth-promoting effects of the four PGPR strains on V. radiata in a pot experiment, in which these strains were inoculated in the rhizosphere soil every 7 days for a total of 4 times. The results revealed that the V. radiata seeds treated with PGPR isolate B. aryabhattai MQB12 showed significantly enhanced root and shoot growth compared to that of other isolates and non-inoculated control CK (). In general, the inoculation of isolates did not produce any harmful effect on V. radiata. Thirty days after the inoculation, both fresh weight and dry weight in underground and aerial parts of seedlings were significantly increased, and fresh weight () and dry weight () in the aerial parts were 1522 mg and 244 mg individually, i.e. 4.3 and 7.6 times that of the control group, respectively. The dry weight () and fresh weight () of the underground part were 5 times and 10 times that of the control group, respectively, and the fresh weight of the root reached 921.9 mg. After MQB12 inoculation, the stem length () also changed significantly, reaching 23 cm, which was about 14 cm higher than that of the control group and 2.7 times higher. The application of the multiple traits isolate B. aryabhattai MQB12 significantly promoted the growth and development of V. radiata roots and shoots in the greenhouse.
Figure 3. Growth-promotion effects of the obtained strains MQB5、7、10、12 on V. radiata seedlings after cocultivation for 30 days (A), CK was irrigated with sterile water. The effects of PGPR strains MQB5, 7, 10, and MQB12 on the V. radiata seedlings shoot fresh weight (B), shoot dry weight (C), root fresh weight (D), root dry weight (E), stem length (F), first-order lateral root (G), leaf number (H), root length (I). Different letters (a-c) inducate statistically significant differences between each treatment vs the control (CK) group (p < 0.05), and the significance was determined via ANOVA.
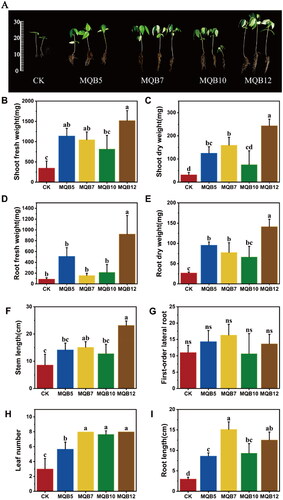
In addition to MQB12, the other three strains also showed a growth promotion effect on V. radiata, and the biomass of each plant part was increased by at least 2 times. The inoculation of each of the four strains significantly increased the dry and fresh weight, leaf number (), stem length and root length () of the test materials, but there was no significant change in the first-order lateral root number () of the plants. However, from the perspective of plant root development, the root tissue of V. radiata plants in the inoculation group was more dense than that in the control group. We speculate that the PGPR strain might enhance the absorption and utilization of nutrients in soil and promote the growth of plants by promoting the formation of secondary lateral roots of V. radiata.
Discussion
PGPR species have powerful biological functions and are of key importance due to their direct or indirect role in plant growth, development, health, nutrition and ability to stabilize the rhizosphere structure in various soil type conditions. In the present study, 41 rhizobacterial strains were successfully isolated from the rhizosphere of D. dichotoma, of which 21 strains had promising biological functions. Among these isolates, B. aryabhattai MQB12, B. pumilus MQB5, B. velezensis MQB10 and P. agglomerans MQB7 showed robust and multiple plant growth-promoting traits such as phosphate solubilization capability, siderophores, IAA and extracellular cellulase production.
PSBs are a major group of plant growth-promoting bacteria. Currently, known PSBs include Enterobacteria sp., Bacillus sp. [Citation38], Pantoea sp. [Citation39], Pseudomonas sp. and Burkholderia sp. [Citation40], among which Bacillus sp. and Pseudomonas sp. have been widely used as growth promoting bacteria. PSBs dissolve mineral phosphorus mainly by promoting the decomposition of insoluble phosphorus into nanoparticles and further producing organic acids [Citation41]. In this study, the isolated strains all had a certain phosphate-dissolving ability and the phosphate solubilization index in the medium with tricalcium phosphate as the substrate was 1.11–2.19. Among them, the phosphate-dissolving capacity of MQB5, MQB7, MQB10 and MQB12 strains was increased 12-20.23 times () compared with the control group, indicating that all four strains could dissolve phosphate well.
Yu et al. [Citation42] isolated three Pseudomonas strains from the soil, with a maximum phosphorus solubility of 283 mg/L, and at the same time produced 19.33 g/L 2-keto gluconic acid within 2 days. It was speculated that different strains of different species have different phosphorus solubility due to their physiological characteristics and the content of organic acids produced. In this study, in the liquid medium with tricalcium phosphate as the only phosphorus source, the process of PSB absolutizing inorganic phosphorus was consistent with the process of pH reduction (). This is in agreement with the study of Tchakounté [Citation43]; that is, PSB can dissolve inorganic minerals by producing organic acids, and the change of pH is positively correlated with the increase of dissolved phosphorus content. Due to the strong performance of PSB, inoculation in soil will increase the content of available phosphorus in soil and the abundance of beneficial bacteria in soil [Citation44] for a certain period to promote plant growth, which can be specifically manifested as an increase in crop biomass and yield [Citation45]. PSBs have been used as important growth-promoting bacteria in modern agriculture.
IAA is an important plant hormone in plant root elongation and embryonic development; it participates in the interaction between plants and microorganisms. Microorganisms regulate plant root morphogenesis by recognizing auxin signals [Citation46]. Low auxin concentration is beneficial to plant development, but high IAA will inhibit plant growth. Currently isolated growth-promoting bacteria, such as Enterobacter cloacae, Klebsiella pneumonia and Bacillus velezensis, all have certain ability to produce IAA, with a yield of about 36-76 mg/L [Citation47, Citation48]. In this study, there were nine strains with IAA yield higher than 10 mg/L, and the highest IAA yield was 134.46 mg/L. Part of the promoting effect of growth-promoting bacteria on plant growth comes from IAA. Han et al. [Citation49] explored the promoting mechanism of filamentous fungus Clonostachys rosea on tomato growth: promoting root elongation by synthesizing IAA. Therefore, IAA produced by growth-promoting bacteria has a positive promoting effect on plant growth. In addition, some of the strains we isolated also had siderophores activity, with orange-yellow halos visible on CAS plates, and siderophores production ranging from SU of 32% to 93%. The content of siderophores secreted by fungi is relatively low [Citation51], about 50%. In our study, six strains were high-yielding siderophores, and the yield was above 80%, with the highest being 97.1%. Siderophores-producing bacteria have certain inhibitory effects on pathogenic bacteria. Chandwani et al. [Citation52] screened a strain of Bacillus subtilis, and its siderophores-bearing extract had a good inhibitory effect on Ralstonia solanacearum. The experiments of Li et al. [Citation53] also showed that Bacillus velezensis with siderophores activity could be used as a biological control agent to inhibit Botrytis cinerea and promote the growth of tomato seedlings. In addition, siderophores can also indirectly promote plant growth by improving soil heavy metal pollution through interaction with rhizosphere microorganisms [Citation54].
In addition, growth-promoting bacteria can also degrade cellulose waste residues in soil by producing cellulose enzymes [Citation55], providing nitrogen and carbon sources for host plants to improve soil nutrients and maintain C and N balance. We screened four strains with cellulolytic activity. In previous studies, fungal strains had good cellulolytic activity because they had developed mycelium, but in this experiment, the cellulose transparent circle index of MQB5 and MQB10 was greater than 2, indicating strong cellulase production activity. Exploring the physiological activity of growth-promoting bacteria and verifying their positive effects on the growth of cash crops can provide a theoretical basis for the practical application of growth-promoting bacteria. Physiological characteristics of plants such as plant height, root length and biomass are important indicators reflecting plant growth and development. Our study showed that four growth-promoting strains MQB5, 7, 10 and 12 all significantly increased plant height, aboveground and underground biomass, leaf number, root length and lateral root number of V. radiata plants. On the one hand, these changes may be due to the production of metabolic products such as plant hormones [Citation56] and siderophores support, which promote root elongation and lateral root development. On the other hand, the strain produced a variety of organic acids, dissolved insoluble minerals into organic ions, and increased the absorption and utilization of nutrient elements by plants [Citation40]. Morais et al. [Citation57] showed that inoculation with growth-promoting bacteria could accelerate the ripening of strawberries, change fruit morphology, and increase fruit yield, quality and relative water content of plants. In this study, the fresh weight and dry weight of plants after inoculation were significantly higher than those of the control group (CK), which was consistent with the results of Bakaeva et al. [Citation58]. Singh et al. [Citation59] summarized the growth-promoting bacteria of Pseudomonas, proved the excellent role of Pseudomonas strains in plant growth promotion, also demonstrated the important contribution of PGPR inoculation to the intel oxidase system of plants. In this study, MQB12 did not show the best PGPR activity but had the best growth-promoting effect, which was significantly different from the other three strains. It was speculated that on the one hand, MQB12 also produced other highly active metabolites conducive to plant growth, and on the other hand, the strength of the growth-promoting effect also depended on the adaptability of the strain to the environment.
The health and nutrition of microbiota in soil ecological niches can directly or indirectly affect plant growth and development, which can directly or indirectly show a non-negligible impact on plant growth and development and stabilize the rhizosphere structure in various soil type conditions. Rhizosphere growth-promoting microorganisms can regulate plant health and nutrition by dissolving soil metal elements and producing plant hormones and secondary metabolites [Citation60], which is crucial for plant growth. Therefore, it is of practical importance to study and explore robust rhizosphere growth-promoting microorganisms.
Conclusions
In our study, we isolated 41 strains from the rhizosphere of D. dichotoma and found 21 strains that have one more than one PGP traits, including phosphate solubilization, siderophores, IAA and extracellular cellulase production. We chose the four most potent multifunctional stains to act on V. radiata for detecting the effects of plant growth promotion. The results of the pot experiment showed that all four isolates have excellent PGP function and increase biomass and length of stem and root, lateral root and leaf number. Our study verified that the rhizosphere soil of D. dichotoma has complex microorganisms and they have robust plant growth promoting functions.
Authors contributions
RZ conducted the experimental design and wrote the article under the guidance of YW and WFH. FH gave guidance to the pro-life and pot experiments of rhizosphere growth-promoting bacteria. YFZ, ZQH and HNX completed the main experimental content, and LFX, JCW and JH analyzed the experimental data. All authors have read and approved the final manuscript.
Acknowledgements
Shanghai Invitrogen Company Ltd. is acknowledged for purification and 16S rRNA sequencing.
Disclosure statement
No potential conflict of interest was reported by the author(s).
Availability of data
The data that support the findings reported in this study are available from the corresponding author [YW] upon reasonable request.
Additional information
Funding
References
- Li Y, Kong F, Li S, et al. Insights into the driving factors of vertical distribution of antibiotic resistance genes in long-term fertilized soils. J Hazard Mater. 2023;456:1. doi: 10.1016/j.jhazmat.2023.131706.
- Wang Z, Geng Y, Liang T. Optimization of reduced chemical fertilizer use in tea gardens based on the assessment of related environmental and economic benefits. Sci Total Environ. 2020;713:136439. doi: 10.1016/j.scitotenv.2019.136439.
- Molina-Santiago C, Matilla MA. Chemical fertilization: A short-term solution for plant productivity? Microb Biotechnol. 2020;13(5):1311–14. doi: 10.1111/1751-7915.13515.
- Ling N, Wang T, Kuzyakov Y. Rhizosphere bacteriome structure and functions. Nat Commun. 2022;13(1):836. doi: 10.1038/s41467-022-28448-9.
- Mommer L, Kirkegaard J, Van Ruijven J. Root–Root interactions: Towards a rhizosphere framework. Trends Plant Sci. 2016;21(3):209–217. doi: 10.1016/j.tplants.2016.01.009.
- Orozco-Mosqueda M, Fadiji AE, Babalola OO, et al. Rhizobiome engineering: Unveiling complex rhizosphere interactions to enhance plant growth and health. Microbiol Res. 2022;263:127137. doi: 10.1016/j.micres.2022.127137.
- Li J, Zhou B, Li T, et al. Isolation of rhizobacteria from the Cenchrus fungigraminus rhizosphere and characterization of their nitrogen-fixing performance and potential role in plant growth promotion. Plant Soil. 2023;489(1–2):405–421. doi: 10.1007/s11104-023-06028-0.
- Wang S, Chen S, Wang B, et al. Screening of endophytic fungi from Cremastra appendiculata and their potential for plant growth promotion and biological control. Folia Microbiol (Praha). 2023;68(1):121–133. doi: 10.1007/s12223-022-00995-0.
- Shameem M R, Sonali J MI, Kumar PS, et al. Rhizobium mayense sp. Nov., an efficient plant growth-promoting nitrogen-fixing bacteria isolated from rhizosphere soil. Environ Res. 2023;220:115200. doi: 10.1016/j.envres.2022.115200.
- He T, Xu Z-J, Wang J-F, et al. Improving cadmium accumulation by Solanum nigrum L. via regulating rhizobacterial community and metabolic function with phosphate-solubilizing bacteria colonization. Chemosphere. 2022;287(Pt 2):132209. doi: 10.1016/j.chemosphere.2021.132209.
- Nawaz A, Mubeen F, Imran A, et al. Contribution of potassium solubilizing bacteria in improved potassium assimilation and cytosolic K/Na ratio in rice (Oryza sativa L.) under saline-sodic conditions. Front Microbiol. 2023;14:1196024. doi: 10.3389/fmicb.2023.1196024.
- Gang S, Saraf M, Waite CJ, et al. Mutualism between Klebsiella SGM 81 and Dianthus caryophyllus in modulating root plasticity and rhizospheric bacterial density. Plant Soil. 2018;424(1):273–288. doi: 10.1007/s11104-017-3440-5.
- Bach E, Seger G, Fernandes G, et al. Evaluation of biological control and rhizosphere competence of plant growth promoting bacteria. Appl Soil Ecol. 2016;99:141–149. doi: 10.1016/j.apsoil.2015.11.002.
- Timofeeva AM, Galyamova MR, Sedykh SE. Bacterial siderophores: Classification, biosynthesis, perspectives of use in agriculture. Plants (Basel). 2022;11(22):3065. doi: 10.3390/plants11223065.
- Zhang W, Zhang Y, Wang X, et al. Siderophores in clinical isolates of Klebsiella pneumoniae promote ciprofloxacin resistance by inhibiting the oxidative stress. Biochem Biophys Res Commun. 2017;491(3):855–861. doi: 10.1016/j.bbrc.2017.04.108.
- Yang W, Gong T, Wang J, et al. Effects of compound microbial fertilizer on soil characteristics and yield of wheat (Triticum aestivum L.). J Soil Sci Plant Nutr. 2020;20(4):2740–2748. doi: 10.1007/s42729-020-00340-9.
- Valle Expósito CD, López JÁ, Liu J, et al. Development of a cold-active microbial compound biofertilizer on the improvement for rice (Oryza sativa L.) tolerance at low-temperature. Rhizosphere. 2022;24:100586. doi: 10.1016/j.rhisph.2022.100586.
- Rafique E, Mumtaz MZ, Ullah I, et al. Potential of mineral-solubilizing bacteria for physiology and growth promotion of Chenopodium quinoa Willd. Front Plant Sci. 2022;13:1004833. doi: 10.3389/fpls.2022.1004833.
- Rawat P, Shankhdhar D, Shankhdhar S. Synergistic impact of phosphate solubilizing bacteria and phosphorus rates on growth, antioxidative defense system, and yield characteristics of upland rice (Oryza sativa L.). J Plant Growth Regul. 2022;41(6):2449–2461. doi: 10.1007/s00344-021-10458-4.
- Mengstie GY, Awlachew ZT, Degefa AM. Selected rhizobacteria strains as potential growth promoters and biocontrol agents against chocolate spot disease in faba bean grown in pots. Biotechnol Biotechnological Equipment. 2023;37(1):2297885. doi: 10.1080/13102818.2023.2297885.
- Shafi J, Tian H, Ji M. Bacillus species as versatile weapons for plant pathogens: A review. Biotechnol Biotechnological Equipment. 2017;31(3):446–459. doi: 10.1080/13102818.2017.1286950.
- Admassie M, Woldehawariat Y, Alemu T, et al. The role of plant growth-promoting bacteria in alleviating drought stress on pepper plants. Agric Water Manage. 2022;272:107831. doi: 10.1016/j.agwat.2022.107831.
- Felestrino ÉB, Vieira IT, Caneschi WL, et al. Biotechnological potential of plant growth-promoting bacteria from the roots and rhizospheres of endemic plants in ironstone vegetation in southeastern Brazil. World J Microbiol Biotechnol. 2018;34(10):156. doi: 10.1007/s11274-018-2538-0.
- Qu Z, Li Y-h, Xu W-h, et al. Different genotypes regulate the microbial community structure in the soybean rhizosphere. J Integr Agriculture. 2023;22(2):585–597. doi: 10.1016/j.jia.2022.08.010.
- Hu L, Robert CAM, Cadot S, et al. Root exudate metabolites drive plant-soil feedbacks on growth and defense by shaping the rhizosphere microbiota. Nat Commun. 2018;9(1):2738. doi: 10.1038/s41467-018-05122-7.
- Bourak K, Sare AR, Allaoui A, et al. Impact of two phosphorus fertilizer formulations on wheat physiology, rhizosphere, and rhizoplane microbiota. Int J Mol Sci. 2023;24(12):9879. doi: 10.3390/ijms24129879.
- Yang L, Huang Y, Lima LV, et al. Rethinking the ecosystem functions of Dicranopteris, a widespread genus of ferns. Front Plant Sci. 2020;11:581513. doi: 10.3389/fpls.2020.581513.
- Zhang H, Xie J-S, Lyu M-K. Effect of Dicranopteris dichotoma on spectroscopic characteristic of dissolved organic matter in red soil erosion area. Chinese J Plant Ecol. 2017;41(8):862–871. doi: 10.17521/cjpe.2016.0363.
- Li Q-y, Zhu C-L, Yu J-B, et al. Response of soil bacteria of Dicranopteris dichotoma populations to vegetation restoration in red soil region of China. J Soil Sci Plant Nutr. 2023;23(1):456–468. doi: 10.1007/s42729-022-01058-6.
- Pikovskaya RI. Mobilization of phosphorus in soil in connection with the vital activity of some microbial species. Microbiology. 1948;17:362–370.
- Singh TB, Sahai V, Goyal D, et al. Identification, characterization and evaluation of multifaceted traits of plant growth promoting rhizobacteria from soil for sustainable approach to agriculture. Curr Microbiol. 2020;77(11):3633–3642. doi: 10.1007/s00284-020-02165-2.
- Glickmann E, Dessaux Y. A critical examination of the specificity of the Salkowski reagent for indolic compounds produced by phytopathogenic bacteria. Appl Environ Microbiol. 1995;61(2):793–796. doi: 10.1128/aem.61.2.793-796.1995.
- Schwyn B, Neilands JB. Universal chemical assay for the detection and determination of siderophores. Anal Biochem. 1987;160(1):47–56. doi: 10.1016/0003-2697(87)90612-9.
- Wang Y, Huang W, Ali SW, et al. Isolation, identification, and characterization of an efficient siderophore producing bacterium from heavy metal contaminated soil. Curr Microbiol. 2022;79(8):227. doi: 10.1007/s00284-022-02922-5.
- James N, Umesh M, Sarojini S, et al. Unravelling the potential plant growth activity of halotolerant Bacillus licheniformis NJ04 isolated from soil and its possible use as a green bioinoculant on Solanum lycopersicum L. Environ Res. 2023;216(Pt 2):114620. doi: 10.1016/j.envres.2022.114620.
- Wakarera PW, Ojola P, Njeru EM. Characterization and diversity of native Azotobacter spp. isolated from semi-arid agroecosystems of Eastern Kenya. Biol Lett. 2022;18(3):20210612. doi: 10.1098/rsbl.2021.0612.
- Thakur R, Srivastava S, Yadav S. Multitrait Pseudomonas sp. isolated from the rhizosphere of Bergenia ciliata acts as a growth-promoting bioinoculant for plants. Front Sustain Food Syst. 2023;7:1097587. doi: 10.3389/fsufs.2023.1097587.
- Wang Z, Zhang H, Liu L, et al. Screening of phosphate-solubilizing bacteria and their abilities of phosphorus solubilization and wheat growth promotion. BMC Microbiol. 2022;22(1):296. doi: 10.1186/s12866-022-02715-7.
- Li L, Chen R, Zuo Z, et al. Evaluation and improvement of phosphate solubilization by an isolated bacterium Pantoea agglomerans ZB. World J Microbiol Biotechnol. 2020;36(2):27. doi: 10.1007/s11274-019-2744-4.
- Chen J, Zhao G, Wei Y, et al. Isolation and screening of multifunctional phosphate solubilizing bacteria and its growth-promoting effect on Chinese fir seedlings. Sci Rep. 2021;11(1):9081. doi: 10.1038/s41598-021-88635-4.
- Estrada-Bonilla GA, Lopes CM, Durrer A, et al. Effect of phosphate-solubilizing bacteria on phosphorus dynamics and the bacterial community during composting of sugarcane industry waste. Syst Appl Microbiol. 2017;40(5):308–313. doi: 10.1016/j.syapm.2017.05.003.
- Yu H, Wu X, Zhang G, et al. Identification of the phosphorus-solubilizing bacteria strain JP233 and its effects on soil phosphorus leaching loss and crop growth. Front Microbiol. 2022;13:892533. doi: 10.3389/fmicb.2022.892533.
- Tchakounté GVT, Berger B, Patz S, et al. Selected rhizosphere bacteria help tomato plants cope with combined phosphorus and salt stresses. Microorganisms. 2020;8(11):1844. doi: 10.3390/microorganisms8111844.
- Saadouli I, Mosbah A, Ferjani R, et al. The impact of the inoculation of phosphate-solubilizing bacteria Pantoea agglomerans on phosphorus availability and bacterial community dynamics of a semi-arid soil. Microorganisms. 2021;9(8):1661. doi: 10.3390/microorganisms9081661.
- Bilal S, Hazafa A, Ashraf I, et al. Comparative effect of inoculation of phosphorus-solubilizing bacteria and phosphorus as sustainable fertilizer on yield and quality of mung bean (Vigna radiata L.). Plants (Basel). 2021;10(10):2079. doi: 10.3390/plants10102079.
- Finkel OM, Salas-González I, Castrillo G, et al. A single bacterial genus maintains root growth in a complex microbiome. Nature. 2020;587(7832):103–108. doi: 10.1038/s41586-020-2778-7.
- Yankey R, Omoor INA, Karanja JK, et al. Metabolic properties, gene functions, and biosafety analysis reveal the action of three rhizospheric plant growth-promoting bacteria of Jujuncao (Pennisetum giganteum). Environ Sci Pollut Res Int. 2022;29(25):38435–38449. doi: 10.1007/s11356-021-17854-z.
- Cheng S, Jiang JW, Tan LT, et al. Plant growth-promoting ability of Mycorrhizal fusarium strain KB-3 enhanced by its IAA producing endohyphal bacterium, Klebsiella aerogenes. Front Microbiol. 2022;13:855399. doi: 10.3389/fmicb.2022.855399.
- Han Z, Ghanizadeh H, Zhang H, et al. Clonostachys rosea promotes root growth in tomato by secreting auxin produced through the tryptamine pathway. J Fungi (Basel). 2022;8(11):1166. doi: 10.3390/jof8111166.
- Lobo LLB, da Silva M, Carvalho RF, et al. The negative effect of coinoculation of plant growth-promoting bacteria is not related to Indole-3-acetic acid synthesis. J Plant Growth Regul. 2023;42(4):2317–2326. doi: 10.1007/s00344-022-10706-1.
- Chowdappa S, Jagannath S, Konappa N, et al. Detection and characterization of antibacterial siderophores secreted by endophytic fungi from Cymbidium aloifolium. Biomolecules. 2020;10(10):1412. doi: 10.3390/biom10101412.
- Chandwani S, Dewala S, Chavan SM, et al. Complete genome sequencing of Bacillus subtilis (CWTS 5), a siderophore-producing bacterium triggers antagonistic potential against Ralstonia solanacearum. J Appl Microbiol. 2023;134(4):lxad066. doi: 10.1093/jambio/lxad066.
- Li Z, Li J, Yu M, et al. Bacillus velezensis FX-6 suppresses the infection of Botrytis cinerea and increases the biomass of tomato plants. PLoS One. 2023;18(6):e0286971. doi: 10.1371/journal.pone.0286971.
- Grobelak A, Hiller J. Bacterial siderophores promote plant growth: Screening of catechol and hydroxamate siderophores. Int J Phytoremediation. 2017;19(9):825–833. doi: 10.1080/15226514.2017.1290581.
- Behera BC, Sethi BK, Mishra RR, et al. Microbial cellulases – diversity & biotechnology with reference to mangrove environment: A review. J Genet Eng Biotechnol. 2017;15(1):197–210. doi: 10.1016/j.jgeb.2016.12.001.
- Karcz W, Burdach Z. A comparison of the effects of IAA and 4-Cl-IAA on growth, proton secretion and membrane potential in maize coleoptile segments. J Exp Bot. 2002;53(371):1089–1098. doi: 10.1093/jexbot/53.371.1089.
- Morais MC, Mucha Â, Ferreira H, et al. Comparative study of plant growth-promoting bacteria on the physiology, growth and fruit quality of strawberry. J Sci Food Agric. 2019;99(12):5341–5349. doi: 10.1002/jsfa.9773.
- Bakaeva M, Chetverikov S, Timergalin M, et al. PGP-Bacterium Pseudomonas protegens improves bread wheat growth and mitigates herbicide and drought stress. Plants (Basel). 2022;11(23):3289. doi: 10.3390/plants11233289.
- Singh P, Singh RK, Zhou Y, et al. Unlocking the strength of plant growth promoting Pseudomonas in improving crop productivity in normal and challenging environments: A review. J Plant Interact. 2022;17(1):220–238. doi: 10.1080/17429145.2022.2029963.
- Singh A, Yadav VK, Chundawat RS, et al. Enhancing plant growth promoting rhizobacterial activities through consortium exposure: A review. Front Bioeng Biotechnol. 2023;11:1099999. doi: 10.3389/fbioe.2023.1099999.