ABSTRACT
Sepiolidae (Cephalopoda: Sepiolida) are growing in popularity as model organisms, not least because of their well-studied symbiotic relationship with light producing bacteria. Their easy maintenance and cultivation requirements in captivity have further facilitated their use in a wide range of developmental, anatomical, neurophysiological, behavioural and genetic studies, exhibiting promising opportunities for these cephalopods in research. Considering the rising interest in sepiolids, a detailed overview of their behavioural ecology is necessary to understand their evolution and conservation, as well as to aid establishment of good welfare practice when held in captivity. To date, not all aspects of the sepiolid ecology have been investigated in detail, and our current knowledge of their behavioural ecology is, for the most part, restricted to descriptions from less than 10 of the approximately 80 species, occasionally resulting in a generalisation of specific observations across species, genera, or even subfamilies. This review summarises current knowledge on sepiolid behavioural ecology and life history, including discussions on their habitat, life span, activity patterns, hunting and feeding behaviour, anti-predator behaviour, burying behaviour, and reproductive behaviour. Moreover, future directions as well as areas of interest for upcoming research studies are highlighted.
Introduction
Many coleoid cephalopods have been intensively studied in the past due to their dynamic behavioural repertoires (Hanlon and Messenger Citation2018). While this applies especially to groups of larger and commercially valuable species such as octopuses (Octopoda), squids (Oegopsida and Myopsida) and cuttlefish (Sepiidae), there are further cephalopod families that have also been an integral part of research for the last half century or even longer. This is no less true for the family Sepiolidae Leach, 1817, comprising small-sized cephalopods commonly referred to as bobtail squids due to their characteristic rounded (‘bobbed’) mantles (A). However, bobtail squids are not ‘true squids’ in a phylogenetic sense. Most recent studies suggest that these cephalopods are sister to a clade comprising cuttlefish as well as oegopsid and myopsid squids ( = true squids) (Anderson and Lindgren Citation2021) and will therefore be referred to as sepiolids or sepiolid cephalopods throughout this manuscript.
Figure 1. Sepiolid anatomy, using Sepiola atlantica as an example. A, lateral view; B, oral view. Abbreviations: chr —chromatophores; fun —funnel; LI (♂ hec) —left arm I (in male individuals: hectocotylus); LII —left arm II; LIII —left arm III; LIV —left arm IV; man —mantle; RI —right arm I; RII —right arm II; RIII —right arm III; RIV —right arm IV; suc —sucker; ten —tentacle. The 4 arm pairs consist of one arm from either side, e.g. LI and RI form the arm pair I, LII and RII form arm pair II, and so forth. Credits: A–B, Photos taken by Bas van der Sanden.
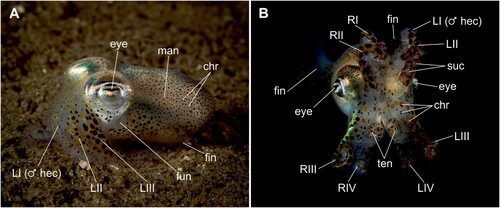
In total, the family Sepiolidae consists of three subfamilies (Sepiolinae Leach, 1817, Rossiinae Appellöf, 1898 and Heteroteuthinae Appellöf, 1898) including close to 80 valid species (Reid and Jereb Citation2005), with many of them only discovered and described in the last two decades (Kubodera and Okutani Citation2002; Young et al. Citation2007; Groenenberg et al. Citation2009; Kubodera et al. Citation2009; de Heij and Goud Citation2010; Kubodera and Okutani Citation2011; Bello Citation2013; Bello and Salman Citation2015; Sanchez et al. Citation2019; Fernández-Álvarez et al. 2021; Reid Citation2021; Sanchez et al. Citation2021; Lu and Okutani Citation2022). While members of the subfamily Sepiolinae generally inhabit tropical and temperate habitats, the subfamily Rossiinae is also associated with colder waters. These two subfamilies exhibit a benthic or nektobenthic lifestyle (Reid and Jereb Citation2005) with some species potentially undergoing vertical migrations of several tens of metres (Bello and Biagi Citation1995). In contrast, members of the third subfamily Heteroteuthinae are commonly found in oceanic and pelagic environments (Reid and Jereb Citation2005). Recently, it has been shown that all three subfamilies are monophyletic, and that the subfamily Sepiolinae, while being genetically divided into an Indo-Pacific and Atlantic-Mediterranean tribe, forms a sister group to the other two subfamilies (Sanchez et al. Citation2021).
Sepiolids are some of the smallest cephalopods and commonly have oval-shaped mantles with lengths between 10 and 80 mm (Reid and Jereb Citation2005). As for all decabrachian cephalopods, sepiolids possess a bilaterally symmetric arm crown with four pairs of prehensile arms as well as two extensible tentacles (B; Nödl et al. Citation2016). Although there are many morphological and anatomical similarities between the three subfamilies, the key features used to distinguish between them are the presence of a web joining the first three arm pairs and an extension of the anterior edge of the ventral mantle forming a ventral shield (indicating heteroteuthine species), and for non-heteroteuthine sepiolids that lack these two features, whether the dorsal edge of the mantle is free (indicating rossiine species) or fused with the head (indicating sepioline species) (Bello Citation1995; Reid and Jereb Citation2005). Within the subfamily Heteroteuthinae, different species can then be identified by their body shape, fin length and position, and width of the occipital band (Reid and Jereb Citation2005), whereas species of the subfamily Rossiinae can be distinguished by the presence of a light organ, the number and shape of their hectocotyli (modified reproductive arms), the number of rows of suckers on the arms, and the development of their anal flaps (Bello Citation1995; Reid and Jereb Citation2005). Species within the third subfamily Sepiolinae display a multitude of varying characteristics and can be identified by the presence and shape of a light organ, the morphology of the male hectocotylus, the shape of the female bursa copulatrix (copulatory pouch), the size, number and pattern of certain suckers across their arms and tentacle clubs, the development of the gladius, and the shape of the ventral mantle margin (Bello Citation1995; Reid and Jereb Citation2005; Bello Citation2020).
As a result of the closure of the life cycle in captivity for several sepiolid species and their comparatively easy maintenance and cultivation requirements (Boletzky and Hanlon Citation1983; Hanlon et al. Citation1997; Nabhitabhata et al. Citation2005; Jones and Richardson Citation2010; Sanchez et al. Citation2019), sepiolid cephalopods have been growing in popularity as model organisms for a range of biological studies. This is especially true for the Hawaiian bobtail squid Euprymna scolopes Berry, 1913 which has been used as a well-established model for host-microbe interactions due to its symbiosis with Vibrio bacteria (Ruby Citation1996; Lee et al. Citation2009b; Mandel and Dunn Citation2016; McAnulty and Nyholm Citation2017; Nyholm and McFall-Ngai Citation2021). In addition to the long-lasting interest into the symbiotic interactions of these cephalopods, in the last two decades sepiolids have also been investigated in terms of their embryonic development (Hartmann et al. Citation2003; Lee et al. Citation2009a, von Byern et al. Citation2016), anatomy and morphology (Kerbl et al. Citation2013; Wild et al. Citation2015; Nödl et al. Citation2016), neural plasticity (Howard et al. Citation2019; Bazarini and Crook Citation2020), associated learning behaviour (Zepeda et al. Citation2017; Seehafer et al. Citation2018), and ‘personality’ traits (Sinn and Moltschaniwskyj Citation2005; Sinn et al. Citation2006, Citation2008), promoting the promising opportunities for the use of these cephalopods in research.
Due to the rising interest in sepiolids as well as the recent sequencing of the nuclear genome of E. scolopes (making it the first example for all decabrachian cephalopods; Belcaid et al. Citation2019), further studies in all of the aforementioned biological research fields will probably be conducted in the near future, and it is conceivable that these cephalopods could be established as model organisms in those fields (Davison and Neiman Citation2021). Therefore, a better understanding of the ecology of sepiolids is not only beneficial as a reference when their behaviour is experimentally manipulated, but may also help to establish good welfare practices for captive individuals.
In this review, I shall bring together the knowledge on the behavioural ecology of sepiolids as well as highlight research aspects that should be addressed in future studies. Due to the nektonic lifestyle of the subfamily Heteroteuthinae together with the lack of observations in the wild for its species, this review mainly focusses on the two subfamilies Sepiolinae and Rossiinae which have frequently been held and studied in laboratory settings. However, information about Heteroteuthinae is presented where available.
Habitat preference
Subfamilies Sepiolinae and Rossiinae
It is widely reported that sepiolids of the subfamilies Rossiinae and Sepiolinae inhabit continental slopes, ranging from intertidal zones to depths of more than 1700 m. Species of the subfamily Sepiolinae commonly inhabit intertidal and neritic zones, with some species extending their depth range down to approximately 500 m, whereas Sepietta oweniana (d'Orbigny, 1841) is considered the most eurybathic sepioline with a depth range from 8 to over 1000 m (Reid and Jereb Citation2005). The bathymetric range of the rossiine subfamily is generally wider, with species occuring in habitats ranging from the neritic zone down to 900 m, whereas Neorossia caroli (Joubin, 1902) displays the widest depth range of the rossiines and all sepiolids, extending from 40 m down to 1744 m (Villanueva Citation1992; Reid and Jereb Citation2005).
Most species are commonly found on sandy or muddy substrates, often associated with seagrass beds, where they spend the daytime buried in the sediment (Reid and Jereb Citation2005). However, some sepiolid species can also be found at sites consisting almost entirely of hard substrate or coral gravel where they either shelter in crevices or bury themselves in small sand patches if available (Drerup et al. Citation2020, Citation2021). While detailed descriptions of the habitat types of sepiolids are scarce, Anderson (Citation1987) reported that Rossia pacifica Berry, 1911 was always found on muddy sand containing both fine sediments as well as organic material, but not on pure mud or bare sand.
For several sepiolid species, migration patterns have been observed. Both for Sepiola atlantica d'Orbigny, 1842 in the Irish Sea (Jones and Richardson Citation2012) as well as for Rossia macrosoma (Delle Chiaje, 1830) in West-Scottish waters (Drerup et al. Citation2021), a migration from deeper offshore waters to shallow inshore waters in summer, followed by a return in autumn, was observed, which is presumably linked to their reproductive behaviour and environmentally more favourable conditions due to increased water temperatures. On the contrary, Rodrigues et al. (Citation2011a) observed that S. atlantica migrated from shallow coastal waters to deeper zones in summer, presumably as the increased water temperature of these shallow coastal areas in the summer months forces this sepiolid species to cooler, deeper waters.
Both Anderson and Mather (Citation1996) and Drerup et al. (Citation2020) reported from field studies that adult sepiolids are commonly encountered close to the bottom, whereas their hatchlings have mainly been found in the water column. These findings coincide with observations from laboratory studies (Bergstrom and Summers Citation1983; Singley Citation1983; Summers and Colvin Citation1989; Hanlon et al. Citation1997; Nabhitabhata et al. Citation2005; Jones and Richardson Citation2010), suggesting that sepiolid hatchlings undergo an actively planktonic phase. Following the latter studies, the length of this planktonic phase is species dependent. Whereas hatchlings of S. atlantica were reported to spend the first six days entirely in the water column before adapting a benthic lifestyle (Jones and Richardson Citation2010), hatchlings of the genus Euprymna Steenstrup, 1887 only spent between 6–48 h constantly swimming before gradually resting longer on the bottom and adapting a fully benthic lifestyle after 30 days (Hanlon et al. Citation1997; Nabhitabhata et al. Citation2005). In contrast to adult sepiolids, little is known about their paralarvae. However, recent studies have shown that sepiolid paralarvae in the North-East Atlantic are not dispersed into the pelagic zone but retained in the neritic water column over the continental slope (Roura et al. Citation2016; Olmos-Pérez et al. Citation2018), probably by avoiding surface offshore currents (Roura et al. Citation2016).
Subfamily Heteroteuthinae
Unlike Rossiinae and Sepiolinae, members of the subfamily Heteroteuthinae are commonly considered pelagic with depth ranges down to almost 1600 m (Villanueva Citation1992; Reid and Jereb Citation2005). While paralarvae of Heteroteuthis dispar (Rüppell, 1844) have been reported from mesopelagic and bathypelagic zones between 1500 and 3000 m, adults commonly occur at depths between 200 and 300 m (Reid and Jereb Citation2005), although Roper (Citation1974) observed a migration of adult individuals to the surface at night. However, several observations obtained in the last half decade indicate that the lifestyle of heteroteuthines is, at least during parts of their life span, potentially less pelagic than long assumed. Not only do species of this sepiolid subfamily presumably spawn their eggs on the sea floor (see Spawning Behaviour section below), the high number of heteroteuthine sepiolids in the stomach contents of demersal predators (Bello Citation1997; Kousteni et al. Citation2018) indicate that these species must get close to the sea floor at some points in time. While the results of Kousteni et al. (Citation2018) additionally show a high abundance of H. dispar over the upper slope of the Mediterranean Sea, Bello (Citation1999) further reported that this species is found more frequently in stomachs of inshore- than offshore-caught albacore [Thunnus alalunga (Bonnaterre, 1788)] which additionally suggests a continental slope habitat. Moreover, observations for other heteroteuthine species also question the idea of an holopelagic life style for this sepiolid subfamily. According to Nesis (Citation1993), species of the genus Heteroteuthis Gray, 1849 are commonly found near seamounts and submarine ridges despite being widely distributed in the open ocean. Similarly, Okutani and Tsuchida (Citation2005) found several individuals of Heteroteuthis hawaiiensis (Berry, 1909) near a seamount with a bottom texture of coarse sand, and observations of Harman and Seki (Citation1990) further indicate a seamount-association for Iridoteuthis iris (Berry, 1909).
Life span
Subfamilies Sepiolinae and Rossiinae
As with the majority of cephalopods sepiolids are very short-lived, with captive Euprymna hyllebergi Nateewathana, 1997 showing one of the shortest life spans of less than four months (Nabhitabhata et al. Citation2005). Boletzky (Citation1983) suggested that Sepiola robusta Naef, 1912 can reach an age of 6–8 months in captivity. By analysing statoliths of captured individuals, Czudaj et al. (Citation2012) postulated a life span of up to six months for S. oweniana, which is significantly shorter than the estimate by Mangold and Froesch (Citation1977) of up to nine months for this species. Of the subfamily Sepiolinae, E. scolopes, Rondeletiola minor (Naef, 1912) and S. atlantica show some of the longest recorded life spans of up to 10 months (Singley Citation1983; Jones and Richardson Citation2010; Önsoy et al. Citation2013). Members of the subfamily Rossiinae which are generally associated with colder waters tend to live longer. For captive R. pacifica a life span of roughly 1.5 years was reported (Summers Citation1985; Summers and Colvin Citation1989), and Zumholz and Frandsen (Citation2006) suggested a life span of 3–4 years for Rossia moelleri Steenstrup, 1856. As several environmental factors, most notably the water temperature, can affect the growth and life span of cephalopods (Forsythe and Hanlon Citation1988; Wood and O'Dor Citation2000; Domingues et al. Citation2002; Arkhipkin et al. Citation2015), the data presented above should be treated with caution, especially when comparing life spans within the same species. However, the difference in life spans between the species mentioned above also reflects their reproduction seasonality. The species with life expectancies of less than 10 months all inhabit tropical and temperate waters that facilitate year-round reproduction along with higher growth rates and earlier maturation (Rodrigues et al. Citation2011b, Citation2012; Czudaj et al. Citation2012, Citation2013; Salman Citation2014). In contrast, those species displaying a longer life span (of more than a year) are associated with colder waters and presumably reproduce only in late summer and autumn (Brocco Citation1971; Zumholz and Frandsen Citation2006; Drerup et al. Citation2021).
Subfamily Heteroteuthinae
No studies have been published stating the life span for species of the subfamily Heteroteuthinae.
Hunting and feeding behaviour
Subfamilies Sepiolinae and Rossiinae
Sepiolids of the subfamilies Rossiinae and Sepiolinae commonly spend the daytime buried in the sediment and emerge at night to hunt (Boletzky et al. Citation1971; Shears Citation1988; Drerup et al. Citation2020), although occasional feeding activity during the day has been observed in captivity (Moynihan Citation1983; Shears Citation1988; Drerup et al. Citation2020). For laboratory-based experiments under natural light phases, Shears (Citation1988) stated that in a 24 h period, approximately 96 % of E. scolopes activities were observed at night. Additionally, Drerup et al. (Citation2020) reported that in a 24 h period captive individuals of Eumandya parva (Sasaki, 1914) spend 41.4 ± 1.2 % of the time buried in the sediment, 27.0 ± 15.9 % partly buried in the sediment, 22.6 ± 14.5 % resting on the sediment, and 9.0 ± 4.6 % swimming in the water column (with individuals swimming continuously for up to 170 min). Stomach content analyses by Shears (Citation1988) of E. scolopes caught from the wild, and long-term recordings of captive Eum. parva by Drerup et al. (Citation2020), have shown that most hunting activity takes place at the beginning of the night. Sepiolid prey choice is not yet fully understood but consists mostly of crustaceans (e.g. mysids, euphausiids, and decapods), although fish, polychaetes, echinoderms, and bivalves may also form part of their diet (Mercer Citation1968; Brocco Citation1971; Boletzky and Hanlon Citation1983; Orsi Relini and Massi Citation1988; Shears Citation1988; Golikov et al. Citation2019, Citation2020). Furthermore, occasional cannibalistic interactions have been reported for captive sepiolids (Bergstrom and Summers Citation1983; Singley Citation1983; Bergström Citation1985; Nabhitabhata et al. Citation2005).
Sepiolids are visual predators, and their hunting behaviour is usually evoked by either active (e.g. swimming or walking) or passive (e.g. floating) locomotion of prey (Hanlon et al. Citation1997; Drerup et al. Citation2020). Dead prey are generally neglected and will only be taken when artificially kept in motion (Boletzky et al. Citation1971; Yau and Boyle Citation1996). Prey capture follows a similar sequence to that described for cuttlefish of the genus Sepia Linnaeus, 1758, namely attention, positioning, and seizure (Messenger Citation1968). After noticing a potential prey item, sepiolids face the prey with their arms forming an elongated cone, whereby the tentacles are slightly protruding (A-B). The prey is then caught by a swift approach along with simultaneous forward-shooting of their tentacles (C-F; ; Boletzky et al. Citation1971; Brocco Citation1971; Moynihan Citation1983; Bergström Citation1985; Drerup et al. Citation2020, Citation2021). For Eum. parva, an average tentacular strike speed of 0.88 m s-1 was calculated (Drerup et al. Citation2020). This is significantly slower than in larger cephalopods such as the squids Lolliguncula brevis (Blainville, 1823) (1.23 m s-1; Jastrebsky et al. Citation2017) and Doryteuthis pealei (Lesueur, 1821) (2.3 m s-1; Kier and van Leeuwen Citation1997). After a successful capture, the prey is pulled back into the buccal area of the sepiolid and instantly grabbed by their arms (G-H; Choe Citation1966; Boletzky et al. Citation1971; Brocco Citation1971; Boletzky Citation1983; Moynihan Citation1983; Bergström Citation1985; Drerup et al. Citation2020, Citation2021). Tentacular retraction in sepiolids is performed in a straight line, which is in contrast to the coiling retraction described for cuttlefish (Boletzky Citation1983). Moynihan (Citation1983) stated that in order to avoid the long, less nutritious appendages of a shrimp’s head (e.g. the antennae), they were either broken off by the sepiolids using their arms, or the shrimps were eaten ‘tail-first’. While smaller shrimps are usually fully consumed, the exoskeletons of larger individuals are commonly ejected after the internal content has been consumed (Moynihan Citation1983; Drerup et al. Citation2020). While Eum. parva and R. macrosoma were observed to maintain their body colouration throughout the hunting sequence (Drerup et al. Citation2020, Citation2021), R. pacifica, S. oweniana and hatchlings of S. atlantica blanch while approaching prey and regain their dark colouration after the tentacular strike (Brocco Citation1971; Bergström Citation1985; Rodrigues et al. Citation2011c), whereas E. scolopes and Sepiola affinis Naef, 1912 exhibit a variety of colour patterns, especially during tentacle ejection and prey ingestion (Moynihan Citation1983; Mauris Citation1989).
Figure 2. Hunting behaviour in sepiolids. A, B, An individual of Eumandya parva approaching its prey (here: mysid shrimp) with its arms forming an elongated cone; C–F, Tentacular strike to capture the prey; G, H, Retraction of tentacles to pull the prey towards the mouth. Credits: A–H, Reprinted from Drerup et al. (Citation2020), with permission from Elsevier.
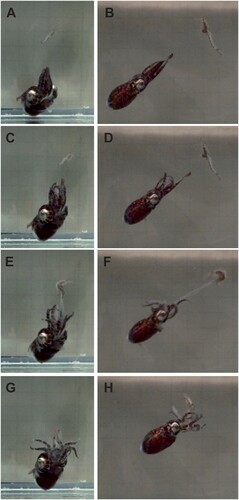
Table 1. Literature dedicated to different ecological aspects of sepiolids. Taxonomic authorities are given only for species not mentioned in the text.
Subfamily Heteroteuthinae
No studies have been published describing the hunting and feeding behaviour for species of the subfamily Heteroteuthinae.
Anti-predator behaviour
Subfamilies Sepiolinae and Rossiinae
The escape responses so far observed in the subfamilies Rossiinae and Sepiolinae are largely identical (). Anderson and Mather (Citation1996) reported that individuals of E. scolopes situated on sandy bottoms would either remain stationary and change to a deep red-brown colouration or bury themselves in the sand (A; Note: While the burying behaviour of sepioline and rossiine sepiolids is undoubtedly a type of anti-predator behaviour, it will be described in more detail in the next section, see ‘Burying behaviour’). On closer approach, E. scolopes then either swims roughly 2 m away, followed by another burying attempt, or jets into the water column to stay there motionless while again exhibiting a red-brown colouration. The latter behaviour was also observed when individuals of E. scolopes were approached in mid-water, although some individuals then either jetted to the bottom or rose to the surface and stayed there motionless while potentially resembling floating seaweed (Anderson and Mather Citation1996). Species occurring in habitats with hard substrates have also been observed to shelter in crevices or under coral fragments (B; Drerup et al. Citation2020, Citation2021).
Figure 3. Anti-predator behaviour in sepiolids. A, B, The stout bobtail squid Rossia macrosoma occupies habitats with both soft and hard substrate and can either bury itself in soft sediment or shelter in rock crevices to avoid predation; C, Individual of Eumandya parva ejecting a stretch of ink, a so-called ‘ink rope’, and holding on to it. The picture of a captive individual was taken with an infrared-sensitive camera, however this behaviour has also been observed in the wild (Drerup et al. Citation2020); D, Individual of E. parva wearing a layer of sediment (‘sand coat’) covering its dorsal mantle; E, Counterillumination behaviour of sepiolids. Left: Species devoid of a light organ cast a shadow below their body. Right: Counterillumination by matching ambient light in species with light organs. Credits: A, B, Photos taken by Mark Skea and reprinted from Drerup et al. (Citation2021) (CC BY); C, D, Reprinted from Drerup et al. (Citation2020), with permission from Elsevier; E, Redrawn from Stabb (Citation2006), with permission from John Wiley and Sons.
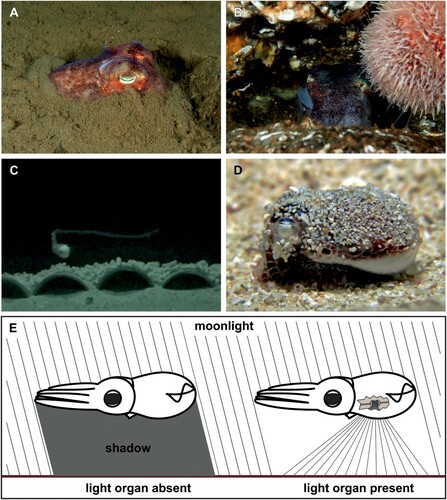
Another escape response reported for Eum. parva (Drerup et al. Citation2020), E. scolopes (Moynihan Citation1983; Anderson and Mather Citation1996; Seehafer et al. Citation2018) and R. pacifica (Shimek Citation1983) is inking, which is a common defence behaviour in many cephalopods (Derby Citation2014; Hanlon and Messenger Citation2018). These sepiolid species eject a series of ink blobs, similar in size, shape and colouration to themselves, which act as decoys (i.e. ‘pseudomorphs’) to confuse potential predators (Moynihan Citation1983; Shimek Citation1983; Anderson and Mather Citation1996; Seehafer et al. Citation2018; Drerup et al. Citation2020). Recently, a second type of inking behaviour was described for Eum. parva. This species was occasionally observed to eject stretches of ink, approximately 4–5 times the length of the sepiolid, and hold on to it motionless (C; Drerup et al. Citation2020). These so-called ‘ink ropes’ have only been documented for deep sea squids so far, where it has been suggested as a type of mimicry due to their resemblance to stinging siphonophores (Bush and Robison Citation2007). Eum. parva, however, seemingly ejects these ink ropes with a masquerade purpose in order to resemble floating seagrass leaves (Drerup et al. Citation2020).
Some species of the genera Eumandya Bello, Citation2020 (Drerup et al. Citation2020), Euprymna (Choe Citation1966; Singley Citation1982; Moynihan Citation1983; Singley Citation1983; Shears Citation1988; Norman and Lu Citation1997; Nabhitabhata et al. Citation2005) and Rossia Owen, 1835 (Drerup et al. Citation2021) are occasionally observed with their dorsal mantle covered in a sand grain layer when emerging from the sediment (D). This so-called ‘sand coat’ (sensu Singley Citation1982) is presumably used as crypsis and can be released as a unit, potentially to confuse predators while escaping (Singley Citation1982; Moynihan Citation1983; Shears Citation1988). It has long been assumed that sand coats are maintained by an antagonistic gland system in the dorsal epidermis of the sepiolids, consisting of two different secretions for either adhesion or release of sediment particles (Singley Citation1982; von Byern and Klepal Citation2006). However, a recent study by von Byern et al. (Citation2017) demonstrated that in E. scolopes the adhesion of sediment particles derives from the secretions of two gland types, whereas the sand coat is likely released mechanically by a large muscular network in the dorsal mantle of the sepiolids. Whether species of other genera use the same or a different mechanism to maintain their sand coats needs to be addressed in future studies.
Most sepiolids of the subfamily Sepiolinae, except species of the genera Inioteuthis Verrill, 1881 and Sepietta Naef, 1912 which have independently lost this feature in the course of evolution (Bello Citation2020; Sanchez et al. Citation2021), possess a typically bilobe-shaped light-emitting organ associated with their ink sac (Herring et al. Citation1981; McFall-Ngai and Montgomery Citation1990). In the subfamily Rossiinae, however, such a light organ is absent in all species but those of the genus Semirossia Steenstrup, 1887 (Boletzky Citation1970). The light emission of these organs corresponds to a mutualistic symbiosis with light-producing Vibrio bacteria (Fidopiastis et al. Citation1998; Stabb Citation2006). While the sepiolid provides the bacteria with nutrients and a safe environment, it uses the emitted luminescence of the bacteria, induced through quorum sensing (Verma and Miyashiro Citation2013), for counter-illumination (E), a means of cryptic defence behaviour (Young and Roper Citation1976). Here, sepiolids swimming in the water match the emitted luminescence from their light organs to the wavelength and intensity of the downwelling light to obscure their silhouette from predators below (Jones and Nishiguchi Citation2004; Stabb Citation2006). Moreover, Stabb and Millikan (Citation2009) further suggested that sepiolids might use their light emission to flash brightly and therefore startle or confuse predators once detected. However, further research is needed to verify this theory and also address whether light emission is used in other behavioural traits, such as intraspecific communication (Bello and Deickert Citation2021).
Subfamily Heteroteuthinae
Unlike the subfamilies Rossiinae and Sepiolinae, little is known about escape responses in the subfamily Heteroteuthinae. It appears, however, that species of the genus Heteroteuthis release luminous fluids with their ink to distract predators (Dilly and Herring Citation1978). All heteroteuthine sepiolids also possess a light organ. In contrast to the bilobed light organ associated with bacterial symbiosis in some of their sepioline and rossiine relatives, as described above, the luminescence of the round-shaped light organ in the subfamily Heteroteuthinae appears to have an autogenic origin (Dilly and Herring Citation1978; but see Leisman et al. Citation1980).
Burying behaviour
Subfamilies Sepiolinae and Rossiinae
Sepiolid cephalopods of the subfamilies Rossiinae [but see Brocco (Citation1971) as well as Boletzky and Boletzky (Citation1973)] and Sepiolinae bury themselves in the sand as a means of protection (). Burying behaviour is considered to be a relatively uncommon escape response in cephalopods, and similar behaviour has only been observed in the cuttlefish Sepia officinalis Linnaeus, 1758 (Mather Citation1986; Hanlon and Messenger Citation1988), bottletail squids (Drerup Citation2021), and some octopus species (Hanlon and Hixon Citation1980; Guerra et al. Citation2006; Hanlon et al. Citation2008, Citation2010). Burying is generally initiated after a sepiolid settles on the sediment (A, B). Anderson et al. (Citation2004) and Rodrigues et al. (Citation2010) reported an ‘alert posture’ in R. pacifica and S. atlantica, respectively, exhibited before the start of the burying procedure, which may benefit sepiolids in the event of a rapid escape (Rodrigues et al. Citation2010).
Figure 4. Burying behaviour in sepiolids. A, An individual of Sepiola intermedia shortly before resting on the sediment; B, Settling/resting posture before the start of burying; C, D, Phase 1 of the burying behaviour. After the formation of a depression in the sediment by tilting the body slightly forwards and ejecting a gentle forward-directed water jet, alternating backward- and forward-directed water jets are ejected to cover nearly the whole body with sediment; E, Phase 2 of the burying behaviour. A series of arm sweeps is conducted to gather sediment from its circular vicinity to cover the remaining body parts; C–E, During the burying procedure, the animal shifts from a dark to a pale body colouration; F, Fully buried individual. Credits: A–F, Photos taken by Christian Drerup.
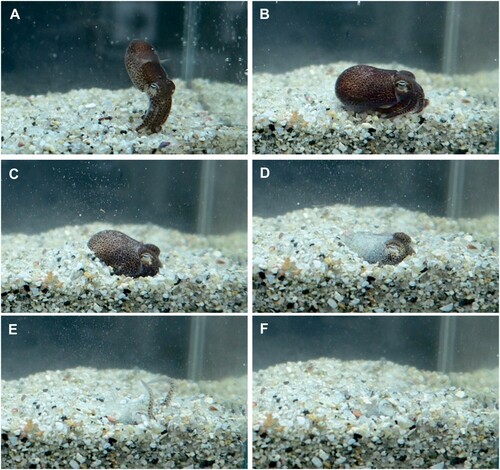
The sepiolid burying behaviour can be divided into two phases, as first described in detail for several species of the genera Sepietta and Sepiola Leach, 1817 by Boletzky and Boletzky (Citation1970). In the first phase, the sepiolid tilts its body slightly forward and creates a depression in the sediment by ejecting a gentle, forward-directed funnel jet. This depression serves as a hold for a stronger, backward-directed funnel jet to displace more sediment from below the animal’s body. By ejecting further alternating forward- and backward-directed funnel jets (A, C, D), the sepiolid immerses its body deeper in the sediment while the dispersed sediment particles descend from the water column and cover its dorsal mantle (Boletzky and Boletzky Citation1970). Fin movements may counteract the funnel jet thrust to maintain a steady position but do not contribute to the actual burying at any point (Boletzky and Boletzky Citation1970; Boletzky Citation1996). These observations have generally been confirmed for other species of the genus Sepiola (Rodrigues et al. Citation2010) and other sepioline genera such as Eumandya (Drerup et al. Citation2020) and Euprymna (Anderson et al. Citation2002; Nabhitabhata et al. Citation2005), as well as for rossiine species of the genus Rossia (Anderson et al. Citation2004; Drerup et al. Citation2021). However, E. scolopes appears to start its burying procedure with a backward- rather than forward-directed funnel jet (Anderson et al. Citation2002).
Subsequently, the second phase starts with the sepiolid stretching out a pair of arms above the sediment, followed by sweeping arm movements to gather sediment from the vicinity (E). This procedure is repeated several times until the animal is fully obscured by sediment (F; Boletzky and Boletzky Citation1970). Sweeping movements of both arms are always closely synchronous unless one arm is impeded by the presence of an obstacle such as a rock or an aquarium wall (Boletzky and Boletzky Citation1970; Drerup et al. Citation2020). While most sepiolid species were reported to use their dorsolateral arms (Anderson et al. Citation2002, Citation2004; Rodrigues et al. Citation2010; Drerup et al. Citation2020, Citation2021), E. hyllebergi performed the second phase of its burying behaviour with its ventrolateral arms (Nabhitabhata et al. Citation2005). Moreover, Moynihan (Citation1983) reported for E. scolopes the use of its tentacles for the sand sweeping movement during phase two. However, the author presumably mistook the tentacles for a pair of arms due to their thin appearance while being stretched out (Boletzky and Boletzky Citation1970). For buried individuals of the species E. hyllebergi and R. pacifica, an angling behaviour, whereby the animals protrude one arm vertically out of the sediment, was reported by Anderson et al. (Citation2004) and Nabhitabhata et al. (Citation2005). The exact purpose of this angling behaviour remains unknown. Throughout the burying activity, sepiolids have been observed to change their colouration from their typical dark appearance to a pale colouration that matches the sediment (C-E; Rodrigues et al. Citation2010; Drerup et al. Citation2020).
The burying duration depends on the sediment type (Boletzky and Boletzky Citation1970; Anderson et al. Citation2002, Citation2004; Drerup Citation2019), and for several Mediterranean species of the subfamily Sepiolinae it has been shown that medium-grained sand most facilitates their burying (Boletzky and Boletzky Citation1970; Drerup Citation2019). On sediment samples taken from the corresponding habitat of a sepiolid species the total burying lasts around 22–25 s (Rodrigues et al. Citation2010; Drerup et al. Citation2020). Other sediment types (e.g. very coarse sand) can prolong the burying significantly. This prolongation derives from a higher number of executed funnel jets and arm sweeps, as well as extended resting periods in between funnel jets, arm sweeps or both burying phases, and can result in a total burying duration of up to several minutes (Anderson et al. Citation2004). Whether the size of sepiolids contributes to the burying duration on a given sediment needs further attention in future studies. While Boletzky and Boletzky (Citation1970) stated for S. robusta that with increasing mantle length the duration of the first phase declines whereas the second phase extends, a correlation between the mantle length and any of the observed burying characteristics could not be identified for either Eum. parva (Drerup et al. Citation2020) or S. atlantica (Rodrigues et al. Citation2010).
Two further peculiarities were reported by Boletzky and Boletzky (Citation1970) regarding the burying of Sepiola and Sepietta species. These authors mentioned that the respiration of buried individuals is not accomplished by mantle expansions and contractions, as it is normally for unburied individuals and other cephalopods (Bone et al. Citation1994). In contrast, buried sepiolids perform their respiration with the aid of the funnel collar which acts as a diaphragm pushing water back and forth, in through the mantle slits and out through the funnel (Boletzky and Boletzky Citation1970). The latter finding was confirmed by Anderson et al. (Citation2002, Citation2004) who observed small bursts of water coming from one side of fully buried sepiolids, resulting in fine, roughly 2 mm wide holes in the sediment.
As a second peculiarity, Boletzky and Boletzky (Citation1970) documented that the eyeballs of Sepiola spp. and Sepietta spp. are rotated vertically when buried, resulting in the pupils facing upwards. This is supported by the observations of Anderson (Citation1997) and Anderson et al. (Citation2002, Citation2004) showing that completely buried individuals of both E. scolopes and R. pacifica were still able to perceive visual stimuli through the sediment. This was concluded from the observation of diffuse jets of ink ejected by buried individuals, evoked by sudden movements outside the tank.
Subfamily Heteroteuthinae
Due to the pelagic lifestyle of the subfamily Heteroteuthinae no burying behaviour has ever been reported for these species.
Mating behaviour
The reproductive biology of sepiolid cephalopods is the most extensively studied feature of their ecology. However, only a small part of this study focuses on their reproductive behaviour (courtship, mating and spawning), while most publications cover aspects such as maturity stages, fecundity, or mating/spawning seasons of sepiolids ().
Table 2. Literature dedicated to reproductive biology and egg characteristics of sepiolids. Taxonomic authorities are given only for species not mentioned in the text or .
Subfamily Sepiolinae
The observations and descriptions of the mating behaviour in sepiolids mostly derive from species of the subfamily Sepiolinae (A-C) which have recently been summarised in detail by Bello and Deickert (Citation2021). Readers interested in sepioline mating behaviour should refer to this review, as here only two additional aspects will be highlighted. Firstly, Bello and Deickert (Citation2021) have stated that sepiolids of the subfamily Sepiolinae are characterised by the hectocotylisation of the left arm of the first arm pair in males (see also Bello Citation2020), and the location of the bursa copulatrix in the left side of the female’s mantle cavity, resulting in an alignment of both sexual organs during copulation. However, a recent publication by Lu and Okutani (Citation2022) describes a new species (Dextrasepiola taenia Lu & Okutani, Citation2022) in which both the hectocotylus and the bursa copulatrix are located on the right side of this sepiolid. While the alignment of both organs still exists in this species, the findings by Lu and Okutani (Citation2022) contradict the previous consensus that all sepioline species are characterised by a left-sided hectocotylus and bursa copulatrix.
Figure 5. Mating in sepiolids. A–C, Mating in sepioline species, using pairs of Sepiola atlantica as an example. Copulation usually occurs: A, B on the sediment, but occasionally C, pairs are also observed slowly swimming; D–G, Mating in rossiine sepiolids, here shown with individuals of Rossia macrosoma; D, Mating of two individuals; E–G, Due to the lack of a bursa copulatrix in female rossiine sepiolids, males implant their spermatophores (E) on the left ventral side close to the oviduct opening or (F–G) on the head or outer surface of the female’s mantle; G, Close-up of the dashed area in F. Credits: A, Photo taken by Mirjam van der Sanden. B, C, Photos taken by Bas van der Sanden. D–G, Photos taken by Mark Skea and reprinted from Drerup et al. (Citation2021) (CC BY).
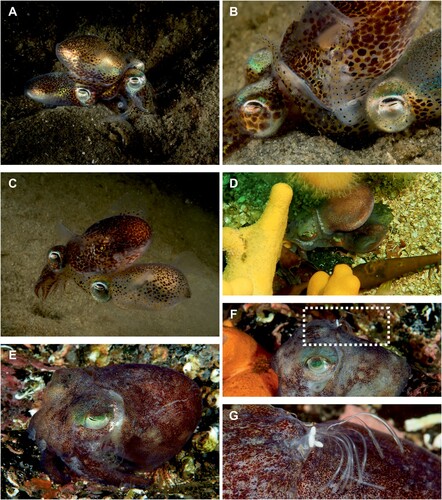
The second aspect worth highlighting is the range of detailed studies on the mating behaviour in Euprymna tasmanica (Pfeffer, 1884), revealing a better insight into the copulatory process and its implications for this species and, potentially, sepiolid cephalopods in general. Here, Squires et al. (Citation2013) reported for male individuals of this species a ‘pumping behaviour’ (increased mantle contractions) during copulation, which may be used to shoot jets of water into the female’s mantle cavity to either dislodge spermatophores or flush out accessory seminal fluids from previous mates. Although this behaviour has also been documented in some cuttlefish species such as Sepia apama Gray, 1849 (Hall and Hanlon Citation2002), Sepia esculenta Hoyle, 1885 (Wada et al. Citation2005) and S. officinalis (Hanlon et al. Citation1999), it has not yet been confirmed for other sepiolid species. Due to the energetic pumping behaviour of male E. tasmanica, and a potentially reduced or inhibited circulation of oxygenated blood in female conspecifics due to the tight physical restriction during copulation, mating events in this species are energetically costly. Franklin et al. (Citation2012) have shown that mating greatly reduces post-copulatory swimming endurance, and that individuals need up to 30 min to recover fully. Hence, both the long-lasting mating behaviour of up to 3 h as well as the post-copulatory recovery period greatly increases the predation risk in E. tasmanica. However, a laboratory study by Franklin et al. (Citation2014) has shown that the presence of a predator effects neither the likelihood nor the duration of mating.
Moreover, E. tasmanica can be considered as polyandric, meaning that females mate with multiple males (Squires et al. Citation2012, Citation2015; Franklin and Stuart-Fox Citation2017). Overall, multiple mating reduces the longevity in female E. tasmanica, probably due to copulatory-related injuries, seminal toxins, pathogens or increased metabolic costs (Franklin and Stuart-Fox Citation2017). However, females that mate with multiple males produce eggs more rapidly and have larger hatchlings, providing strong fitness benefits for reproduction (Squires et al. Citation2012).
Subfamily Rossiinae
There are limited observations on mating for species of the subfamily Rossiinae (Racovitza Citation1894 fide Hanlon and Messenger Citation2018; Mangold-Wirz Citation1963; Brocco Citation1971; Drerup et al. Citation2021). Similar to species of the subfamily Sepiolinae, no courtship behaviour has been observed, although in some cases the sepiolids were already in the copulatory position when first observed (Brocco Citation1971; Drerup et al. Citation2021). Therefore, no detailed description about the male approach and initial male grasp have yet been documented. Mating seemingly also occurs in the ‘male-parallel’ position (D; Brocco Citation1971; Drerup et al. Citation2021). Unlike sepioline species, however, most species of the subfamily Rossinae do not possess a bursa copulatrix (but see Reid Citation1991), and here males implant spermatangia on the head or outer surface of the female’s mantle edge (E–G), particularily on the left ventral side close to the oviduct opening (E); Laptikhovsky et al. Citation2008; Hoving et al. Citation2009; Cuccu et al. Citation2011; Akalin et al. Citation2016; Drerup et al. Citation2021). So far, no mating durations have been documented.
Subfamily Heteroteuthinae
No mating behaviour has ever been observed for heteroteuthine sepiolids. However, Orsi Relini (Citation1995) and Hoving et al. (Citation2008) reported for captured individuals of H. dispar sucker scars on the head and mantle of both males and female individuals. According to the authors, these scars suggest a similar mating position as observed in sepiolids of the subfamilies Rossiinae and Sepiolinae, but further research is needed to confirm this hypothesis. Furthermore, female H. dispar possess a specialised internal seminal receptacle at the posterior end of the visceral mass in the coelom that facilitates successful sperm transfer during mating (Hoving et al. Citation2008), whereas sperm transfer in Stoloteuthis leucoptera (Verrill, 1878) resembles that of rossiine sepiolids, with male St. leucoptera implanting spermatophores onto the female’s head and mantle tissue (Villanueva and Sánchez Citation1993).
Spawning behaviour
Subfamilies Sepiolinae and Rossiinae
Spawning of Sepiolinae and Rossiinae generally occurs at night (Boletzky et al. Citation1971; Arnold et al. Citation1972; Boletzky Citation1983; Singley Citation1983; Jones and Richardson Citation2010; Rodrigues et al. Citation2011b; Drerup et al. Citation2020) or in the early morning (Boletzky et al. Citation1971; Boletzky Citation1983; Hanlon et al. Citation1997; Nabhitabhata et al. Citation2005). However, occasional spawning during daytime has also been observed both in the wild (Drerup et al. Citation2021) as well as in laboratory settings (Boletzky et al. Citation1971; Boletzky Citation1983). The spawning period varies among species and may take up to 4 months in E. tasmanica (Squires et al. Citation2013). Before laying their eggs, sepiolids may investigate given substrata for attachment of their eggs by touching it with their arms (Nabhitabhata et al. Citation2005). After finding a suitable substrate, some species were observed to adopt a vertical ‘sitting’ position while laying their eggs (Boletzky et al. Citation1971), however, depending on the chosen substrate this might vary. Eggs are laid individually by the female clinging to the substrate and attaching one egg at a time onto it (A–C; Drerup et al. Citation2021). Fertilisation probably takes place while spawning, when eggs pass along the bursa copulatrix (Rodrigues et al. Citation2011b), and for E. tasmanica it has been shown that egg clutches can have multiple paternity due to stored sperm of different males deriving from multiple mating events (Squires et al. Citation2014).
Figure 6. Spawning behaviour in sepiolids, here displayed by an individual of Rossia macrosoma. A–C, Spawning sequence. A, Female resting in front of an older egg batch after attaching one of her eggs to the old batch (marked with an arrow). B, The female displays her spawning behaviour by clinging to the substrate and spawning another egg. C, After successfully deploying one egg (marked with an arrow), the female rests for a moment then continues spawning individual eggs as shown above. D, Batch of freshly spawned eggs attached to a batch of older eggs, with some already hatched. Credits: A–D, Photos taken by Mark Skea and reprinted from Drerup et al. (Citation2021) (CC BY).
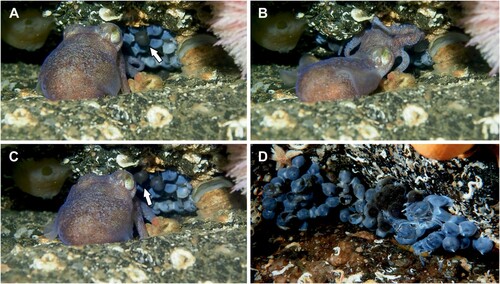
Egg deposition is often cryptic, and eggs are frequently attached to the underside of hard objects such as corals, rocks, empty mollusc shells or man-made objects (; Boletzky and Boletzky Citation1973; Boletzky Citation1983; Singley Citation1983; Summers and Colvin Citation1989; Anderson and Shimek Citation1994; Hanlon et al. Citation1997; Deickert and Bello Citation2005; Nabhitabhata et al. Citation2005; Cuccu et al. Citation2007, Citation2010; Drerup et al. Citation2020, Citation2021), or in laboratory settings to exposed sites such as tank walls (Boletzky et al. Citation1971; Arnold et al. Citation1972; Bergstrom and Summers Citation1983; Boletzky Citation1983; Singley Citation1983; Summers and Colvin Citation1989; Hanlon et al. Citation1997; Norman and Lu Citation1997; Nabhitabhata et al. Citation2005; Jones and Richardson Citation2010; Rodrigues et al. Citation2011b) or on the sediment (Boletzky et al. Citation1971; Rodrigues et al. Citation2011b). Observations from the wild have further shown that eggs may also be attached to or into other living organisms, such as seagrass, sea squirts, sponges, or colonies of bryozoans (Jecklin Citation1934; Cuenot Citation1936 fide Okutani and Sasaki Citation2007; Aldrich and Lu Citation1968; Mercer Citation1968; Boletzky Citation1983; Deickert and Bello Citation2005; Okutani and Sasaki Citation2007).
Sepiolids attach their droplet-shaped eggs either together in one (Boletzky Citation1983; Anderson and Shimek Citation1994; Nabhitabhata et al. Citation2005; Jones and Richardson Citation2010; Rodrigues et al. Citation2011b; Sanchez et al. Citation2019), or several, multi-layered piles (Boletzky and Boletzky Citation1973; Summers and Colvin Citation1989; Anderson and Shimek Citation1994; Nabhitabhata et al. Citation2005; Laptikhovsky et al. Citation2008; Rodrigues et al. Citation2011b; Drerup et al. Citation2021). Often, the outer jelly capsule then becomes leathery or turns opaque and rigid ((D); Choe Citation1966; Arnold et al. Citation1972; Boletzky and Boletzky Citation1973; Summers and Colvin Citation1989; Anderson and Shimek Citation1994; Nabhitabhata et al. Citation2005; Rodrigues et al. Citation2011b; Drerup et al. Citation2021). In contrast, Eum. parva and E. scolopes were observed to deploy eggs individually but in close proximity to each other, whereby each egg is covered in a sand grain layer (Hanlon et al. Citation1997; Sanchez et al. Citation2019; Drerup et al. Citation2020). Occasionally, eggs are deposited on the top of others previously laid by another female (; Deickert and Bello Citation2005; Laptikhovsky et al. Citation2008; Rodrigues et al. Citation2011b; Drerup et al. Citation2021), and observations from the wild have shown polyspecific clutches, containing eggs of different sepiolid species (Deickert and Bello Citation2005).
Sepioline and rossiine sepiolids undergo multiple, monocyclic spawning events but their spawning strategies (sensu Rocha et al. Citation2001) vary between multiple (Bello and Deickert Citation2003; Deickert Citation2009), continuous (Cuccu et al. Citation2007; Laptikhovsky et al. Citation2008, Czudaj et al. Citation2013; Önsoy et al. Citation2013; Salman Citation2014), and intermittent terminal spawning (Gabel-Deickert Citation1995, Rodrigues et al. Citation2011b). Both the number of eggs per individual spawning event, as well as the total number of eggs laid by a female, differ among species; however, the number of eggs per batch seems to decrease over time, with the first usually containing the most eggs (Gabel-Deickert Citation1995; Steer et al. Citation2004; Rodrigues et al. Citation2011b; Squires et al. Citation2013). Among the two subfamilies Rossiinae and Sepiolinae, egg size varies significantly, with those of Rossiinae usually being bigger (up to 18.0 mm; Mangold et al. Citation1998) than those of Sepiolinae (1.4–4.8 mm; Laptikhovsky et al. Citation2008). Gabel-Deickert (Citation1995) highlighted the possibility that these interspecies differences in egg size might indicate a fitness advantage for larger hatchlings with respect to finding prey and avoiding predation, as hatchlings from larger eggs start their life at a size that hatchlings from smaller eggs would reach only after several weeks. As for all decabrachian cephalopods (Boletzky and Villanueva Citation2014; with the potential exception of deep-sea squid, see Seibel et al. Citation2005; Bush et al. Citation2012), no maternal care of the egg capsules has been observed (Nabhitabhata et al. Citation2005; Rodrigues et al. Citation2011b; Drerup et al. Citation2020) and captive females usually die shortly (from hours up to a few days) after their last observed spawning event (Boletzky et al. Citation1971; Brocco Citation1971; Bergstrom and Summers Citation1983; Boletzky Citation1983; Singley Citation1983; Summers Citation1985; Gabel-Deickert Citation1995; Nabhitabhata et al. Citation2005; Jones and Richardson Citation2010; Rodrigues et al. Citation2011b).
Subfamily Heteroteuthinae
There is little information about the spawning behaviour of species of the subfamily Heteroteuthinae. However, as mature females of H. dispar have been found close to the bottom (Orsi Relini Citation1995) and a single egg with an embryo of this species has been obtained from the ocean floor (Boletzky Citation1978 fide Hoving et al. Citation2008), it is likely that heteroteuthine sepiolids spawn their egg masses on the sea bed. An observation of several individuals of H. hawaiiensis close to the bottom supports this theory (Okutani and Tsuchida Citation2005).
Hatching behaviour
Subfamilies Sepiolinae and Rossiinae
Embryonic development, along with the time of hatching, varies between species and relies greatly on the water temperature. For species inhabiting tropical waters, an embryonic phase of 12–18 days at 27 °C (324–486 degree-days, see Wood and O'Dor Citation2000) for E. hyllebergi (Nabhitabhata et al. Citation2005) and 26 days at approximately 21 °C (546 degree-days) for Eum. parva (Drerup et al. Citation2020) has been documented. For temperate water species, Rodrigues et al. (Citation2011c) reported for S. atlantica eggs incubated at 13 °C hatching after roughly 61 days (793 degree-days), whereas for those incubated at 18 °C hatching occurred after approximately 22 days (396 degree-days). Similarly, Boletzky (Citation1983) stated for Sepiola sp. (incorrectly reported as S. robusta, see Bello and Deickert Citation2021) an embryonic development of more than two months at temperatures below 15 °C (> 900 degree-days) and about one month at slightly above 20 °C (∼ 600 degree-days). For species of the subfamily Rossiinae that are generally associated with colder waters, embryonic development takes longer, e.g. hatching of R. pacifica eggs reared between 6 °C to 12 °C started after 4.5 months (810–1620 degree-days; Summers and Colvin Citation1989). Hatching mainly takes place during the night or early morning (Summers Citation1985; Rodrigues et al. Citation2011c) and is achieved by forceful mantle contractions and pressing the mantle end with its terminal spine against the egg case (Arnold et al. Citation1972; Boletzky and Boletzky Citation1973, von Byern et al. Citation2016). Moreover, Summers (Citation1985) found that hatching might be linked to the lunar cycle, as the highest hatching activity for R. pacifica was observed in the nights around a new moon, presumably as a strategy to minimise vulnerability to visually-guided predators.
Subfamily Heteroteuthinae
No studies have been published describing the hatching behaviour of species of the subfamily Heteroteuthinae.
Conclusions and further directions
While the behavioural ecology of pelagic heteroteuthine sepiolids remains for the most part unclear, species of the other two subfamilies Rossiinae and Sepiolinae have been studied both in the wild and in the laboratory over the last decades. Many aspects of the behaviour of the latter two subfamilies appear to be consistent across species; however, slight variations or even novel behaviours were recently observed in certain genera or even on a species-level. However, most work on sepiolid behaviour has been conducted on only a few of the roughly 80 extant species, and the majority of the observations presented in this review are based on studies involving Eum. parva, E. hyllebergi, E. scolopes, R. pacifica, R. macrosoma, and S. atlantica ( and ). Further descriptive studies on the behaviour of other less-investigated sepiolid species are therefore necessary to evaluate how consistent the behaviours presented in this review are across all sepioline and rossiine genera, or contrastingly, how exclusive some behaviours are for certain genera or even species. This accompanies the need for a deeper understanding of the habitat requirements of sepiolids, as certain behavioural traits might have evolved through the exposure to specific environmental stimuli. Moreover, a better comprehension of their habitat preferences will likewise allow better welfare practices when keeping sepiolids in captivity for research purposes.
Our knowledge about the behavioural ecology of sepiolids derives, for the greater part, from laboratory-based studies, whereas observations from the field are rare. Several behavioural features observed on captive sepiolids were found to be largely consistent between different species and genera, indicating that those observations display the natural sepiolid behaviour. However, studies on other organisms have shown that observations made on captive individuals cannot always predict the natural behaviour of wild individuals (e.g. Fisher et al. Citation2015). Therefore, a strong emphasis should also be put on obtaining ecological observations of wild sepiolids to increase our understanding of their behaviour. A promising opportunity to receive observations of cephalopod behaviour from the wild could be through citizen science (Dickinson et al. Citation2010), a usually cost-effective and valuable tool which has recently been used to describe some ecological features of the sepiolid R. macrosoma (Drerup et al. Citation2021) as well as other cephalopods (Ringvold and Taite Citation2018; Drerup and Cooke Citation2021; Laptikhovsky et al. Citation2021; Ringvold et al. Citation2021; Laptikhovsky et al. Citation2022; Freitas et al. Citation2022).
Furthermore, some sepiolid species display behavioural traits such as emitting ventrally directed light, the ability to adhere a sand coat layer to their dorsal mantle, or the use of ink as part of a masquerade purpose that should be addressed in more detail in future studies. For example, the symbiosis between E. scolopes and Vibrio bacteria has been studied in great detail in terms of their host-symbiont interaction (with different aspects of it recently reviewed in Mandel and Dunn Citation2016; McFall-Ngai et al. Citation2021; Nyholm and McFall-Ngai Citation2021; Visick et al. Citation2021). However, the behavioural use of the emitted light needs more detailed research to investigate other potential uses apart from counterillumination, e.g. intraspecific communication as postulated by Stabb and Millikan (Citation2009) as well as Bello and Deickert (Citation2021). By understanding the (potentially different) behavioural aspects relying on light emission in sepiolids, it might also be of interest to investigate variation in the lifestyle, behaviour, and activity of the closely related sepioline genera Sepiola and Sepietta, as species of the latter subfamily lack light organs and therefore may differ in their behavioural responses to those species able to emit light.
In summary, the behavioural ecology of sepiolid cephalopods seems to be relatively consistent amongst species of the two benthic subfamilies Rossiinae and Sepiolinae. However, slight variations in both their morphology and behavioural ecology have been addressed so far, and future research is needed to verify the extent of these variants across further sepiolid species, combining observations on both wild as well as captive individuals.
Acknowledgements
The author would like to thank Dr. Antonio V. Sykes and Dr. Gavan M. Cooke for their comments on an earlier version of this manuscript, as well as Helena C. Brown, Dr. James Herbert-Read, and the two anonymous reviewers for their constructive comments on the latest version of this manuscript. Additionally, the author would like to thank Bas and Mirjam van der Sanden for providing some of the photographs displayed in this manuscript.
Disclosure statement
No potential conflict of interest was reported by the author(s).
References
- Akalin, M. & Salman, A. (2018) Spermatangia implantation in the subfamily Sepiolinae (Cephalopoda: Sepiolidae). Vie et Milieu 68, 119–125.
- Akalin, M., Salman, A. & Önsoy, B. (2016) On the spermatophore implantation in bobtail squid Rossia macrosoma (Cephalopoda: Mollusca). Journal of the Black Sea/Mediterranean Environment 22, 250–258.
- Aldrich, F.A. & Lu, C. (1968) Report on the larva, eggs, and egg mass of Rossia sp. (Decapoda, Cephalopoda) from Bonavista Bay, Newfoundland. Canadian Journal of Zoology 46, 369–371.
- Anderson, F.E. & Lindgren, A.R. (2021) Phylogenomic analyses recover a clade of large-bodied decapodiform cephalopods. Molecular Phylogenetics and Evolution 156, 107038.
- Anderson, R.C. (1987) Field aspects of the sepiolid squid Rossia pacifica Berry, 1911. Western Society of Malacologists - Annual Report 20, 30–32.
- Anderson, R.C. (1997) Low tide and the burying behavior of Euprymna scolopes (Cephalopoda: Sepiolidae). Western Society of Malacologists - Annual Report 29, 12–15.
- Anderson, R.C. & Mather, J.A. (1996) Escape responses of Euprymna scolopes Berry, 1911 (Cephalopoda: Sepiolidae). Journal of Molluscan Studies 62, 543–545.
- Anderson, R.C. & Shimek, R. (1994) Field observations of Rossia pacifica (Berry, 1911) egg masses. The Veliger 37, 117–119.
- Anderson, R.C., Mather, J. & Steele, C. (2002) The burying behavior of the sepiolid squid Euprymna scolopes Berry, 1913 (Cephalopoda, Sepiolidae). Western Society of Malacologists - Annual Report 33, 1–7.
- Anderson, R.C., Mather, J. & Steele, C. (2004) Burying and associated behaviors of Rossia pacifica (Cephalopoda: Sepiolidae). Vie et Milieu 54, 13–20.
- Arkhipkin, A., Argüelles, J., Shcherbich, Z. & Yamashiro, C. (2015) Ambient temperature influences adult size and life span in jumbo squid (Dosidicus gigas). Canadian Journal of Fisheries and Aquatic Sciences 72, 400–409.
- Arnold, J., Singley, C.T. & Williams-Arnold, L. (1972) Embryonic development and post-hatching survival of the sepiolid squid Euprymna scolopes under laboratory conditions. Veliger 14, 361–364.
- Bazarini, S.N. & Crook, R.J. (2020) Environmental estrogen exposure disrupts sensory processing and nociceptive plasticity in the cephalopod Euprymna scolopes. Journal of Experimental Biology 223, jeb218008.
- Belcaid, M., Casaburi, G., McAnulty, S.J., Schmidbaur, H., Suria, A.M., Moriano-Gutierrez, S., Pankey, M.S., Oakley, T.H., Kremer, N., Koch, E.J., Collins, A.J., Nguyen, H., Lek, S., Goncharenko-Foster, I., Minx, P., Sodergren, E., Weinstock, G., Rokhsar, D.S., McFall-Ngai, M., Simakov, O., Foster, J.S. & Nyholm, S.V. (2019) Symbiotic organs shaped by distinct modes of genome evolution in cephalopods. Proceedings of the National Academy of Sciences 201817322.
- Bello, G. (1995) A key for the identification of the Mediterranean sepiolids (Mollusca: Cephalopoda). Bulletin de l'Institut océanographique de Monaco 16, 41–56.
- Bello, G. (1997) Cephalopods from the stomach contents of demersal chondrichthyans caught in the Adriatic Sea. Vie et Milieu 47, 221–227.
- Bello, G. (1999) Cephalopods in the diet of albacore, Thunnus alalunga, from the Adriatic Sea. Journal of Molluscan Studies 65, 233–240.
- Bello, G. (2013) Description of a new sepioline species, Sepiola bursadhaesa n. sp. (Cephalopoda: Sepiolidae), from the Catalan Sea, with remarks and identification key for the Sepiola atlantica group. Scientia Marina 77, 489–499.
- Bello, G. (2020) Evolution of the hectocotylus in Sepiolinae (Cephalopoda: Sepiolidae) and description of four new genera. European Journal of Taxonomy 655, 1–53.
- Bello, G. & Biagi, V. (1995) How benthic are sepiolids? Bulletin de l'Institut océanographique de Monaco 16, 57–61.
- Bello, G. & Deickert, A. (2003) Multiple spawning and spawning batch size in Sepietta oweniana (Cephalopoda: Sepiolidae). Cahiers de Biologie Marine 44, 307–314.
- Bello, G. & Deickert, A. (2021) Observations on mating in Mediterranean Sepiola and Sepietta species and review of mating behaviour in Sepiolinae (Cephalopoda: Sepiolidae). Swiss Journal of Palaeontology 140, 22.
- Bello, G. & Salman, A. (2015) Description of a new sepioline species, Sepiola boletzkyi sp. nov. (Cephalopoda: Sepiolidae), from the Aegean Sea. European Journal of Taxonomy 144, 1–12.
- Bergstrom, B. & Summers, W. (1983) Sepietta oweniana. In: Boyle, P.R. (Ed.), Cephalopod Life Cycles, Vol. 1. Species Accounts. Academic Press, London, pp. 75–91.
- Bergström, B.I. (1985) Aspects of natural foraging by Sepietta oweniana (Mollusca, Cephalopoda). Ophelia 24, 65–74.
- Boletzky, S.v. (1970) Biological results of the University of Miami deep-sea expeditions. 54. On the presence of light organs in Semirossia Steenstrup, 1887 (Mollusca: Cephalopoda). Bulletin of Marine Science 20, 374–388.
- Boletzky, S.v. (1978) Premières données sur le développement embryonnaire du Sépiolide pelagique Heteroteuthis (Mollusca, Cephalopoda). Haliotis 9, 81–84.
- Boletzky, S.v. (1983) Sepiola robusta. In: Boyle, P.R. (Ed.), Cephalopod Life Cycles, Vol. 1. Species Accounts. Academic Press, London, pp. 53–67.
- Boletzky, S.v. (1996) Cephalopods burying in soft substrata: agents of bioturbation? Marine Ecology 17, 77–86.
- Boletzky, S.v. & Boletzky, M.V.v. (1893) Das Eingraben in Sand bei Sepiola und Sepietta (Mollusca, Cephalopoda). Revue Suisse de Zoologie 77, 536–548.
- Boletzky, S.v. & Boletzky, M.V.v. (1973) Observations on the embryonic and early post-embryonic development of Rossia macrosoma (Mollusca, Cephalopoda). Helgoländer wissenschaftliche Meeresuntersuchungen 25, 135–161.
- Boletzky, S.v. & Hanlon, R.T. (1983) A review of the laboratory maintenance, rearing and culture of cephalopod molluscs. Memoirs of the National Museum of Victoria 44, 147–187.
- Boletzky, S.v. & Villanueva, R. (2014) Cephalopod Biology. In: Iglesias, J., Fuentes, L., Villanueva, R. (Eds), Cephalopod Culture. Springer, Dordrecht, pp. 3–16.
- Boletzky, S.v., Boletzky, M.V.v., Frösch, D. & Gätzi, V. (1971) Laboratory rearing of Sepiolinae (Mollusca: Cephalopoda). Marine Biology 8, 82–87.
- Bone, Q., Brown, E. & Travers, G. (1994) On the respiratory flow in the cuttlefish Sepia officinalis. Journal of Experimental Biology 194, 153–165.
- Brocco, S. (1971) Aspects of the biology of the sepiolid squid Rossia pacifica Berry. M.Sc. thesis, University of Victoria.
- Bush, S.L. & Robison, B.H. (2007) Ink utilization by mesopelagic squid. Marine Biology 152, 485–494.
- Bush, S.L., Hoving, H.J.T., Huffard, C.L., Robison, B.H. & Zeidberg, L.D. (2012) Brooding and sperm storage by the deep-sea squid Bathyteuthis berryi (Cephalopoda: Decapodiformes). Journal of the Marine Biological Association of the United Kingdom 92, 1629–1636.
- Choe, S. (1966) On the eggs, rearing, habits of the fry, and growth of some Cephalopoda. Bulletin of Marine Science 16, 330–348.
- Claes, M.F. & Dunlap, P.V. (2000) Aposymbiotic culture of the sepiolid squidEuprymna scolopes: Role of the symbiotic bacterium Vibrio fischeri in host animal growth, development, and light organ morphogenesis. The Journal of Experimental Zoology 286, 280–296.
- Cuccu, D., Mereu, M., Cannas, R., Follesa, M.C., Cau, A. & Jereb, P. (2007) Egg clutch, sperm reservoirs and fecundity of Neorossia caroli (Cephalopoda: Sepiolidae) from the southern Sardinian sea (western Mediterranean). Journal of the Marine Biological Association of the United Kingdom 87, 971–976.
- Cuccu, D., Mereu, M., Cannas, R., Marcias, S., Cau, A. & Jereb, P. (2010) An unusual finding of Sepietta oweniana (Cephalopoda: Sepiolidae) egg clutch. Scientia Marina 74, 555–560.
- Cuccu, D., Mereu, M., Masala, P., Cau, A. & Jereb, P. (2011) Male reproductive system in Neorossia caroli (Joubin 1902) (Cephalopoda: Sepiolidae) from Sardinian waters (western Mediterranean Sea) with particular reference to sexual products. Invertebrate Reproduction & Development 55, 16–21.
- Cuenot, L. (1936) Commensalisme des pontes de Cephalopods avec des Éponges et des Cnidaires. Bulletin des Station Biologique de Arcachon 1936, 37–40.
- Czudaj, S., Pereira, J., Moreno, A., Costa, A.M., Saint-Paul, U. & Rosa, R. (2012) Distribution, abundance, reproduction and ageing of the common bobtail squid Sepietta oweniana (Sepiolidae, Cephalopoda) from the Portuguese Coast. Marine Biology Research 8, 74–86.
- Czudaj, S., Pereira, J., Moreno, A., Saint-Paul, U. & Rosa, R. (2013) Distribution and reproductive biology of the lentil bobtail squid, Rondeletiola minor (Cephalopoda: Sepiolidae) from the Portuguese Atlantic Coast. Marine Biology Research 9, 802–808.
- Davison, A. & Neiman, M. (2021) Mobilizing molluscan models and genomes in biology. Philosophical Transactions of the Royal Society B: Biological Sciences 376, 20200163.
- de Heij, A. & Goud, J. (2010) Sepiola tridens spec. nov., an overlooked species (Cephalopoda, Sepiolidae) living in the North Sea and north-eastern Atlantic Ocean. Basteria 74, 51–62.
- Deickert, A. (2009) Reproductive mode in the genus Sepietta (Cephalopoda: Sepiolidae). Bolletino Malacologico 45, 87–94.
- Deickert, A. & Bello, G. (2005) Egg masses of Sepietta owenian (Cephalopoda: Sepiolidae) collected in the Catalan Sea. Scientia Marina 69, 205–209.
- Derby, C. (2014) Cephalopod ink: production, chemistry, functions and applications. Marine Drugs 12, 2700–2730.
- Dickinson, J.L., Zuckerberg, B. & Bonter, D.N. (2010) Citizen science as an ecological research tool: challenges and benefits. Annual Review of Ecology, Evolution, and Systematics 41, 149–172.
- Dilly, P. & Herring, P.J. (1978) The light organ and ink sac of Heteroteuthis dispar (Mollusca: Cephalopoda). Journal of Zoology 186, 47–59.
- Domingues, P.M., Sykes, A. & Andrade, J.P. (2002) The effects of temperature in the life cycle of two consecutive generations of the cuttlefish Sepia officinalis (Linnaeus, 1758), cultured in the Algarve (South Portugal). Aquaculture International 10, 207–220.
- Drerup, C. (2019) Habitat preference and behavioural ecology of bobtail squids (Sepiolidae). M.Sc. thesis, University of Algarve.
- Drerup, C. (2021) First detailed description of the burying behaviour of a bottletail squid, Sepiadarium kochii Steenstrup, 1881. Molluscan Research 41, 87–91.
- Drerup, C. & Cooke, G.M. (2021) Shoaling behaviour in the European cuttlefish Sepia officinalis. Ethology 127, 1101–1108.
- Drerup, C., Sykes, A.V. & Cooke, G.M. (2020) Behavioural aspects of the spotty bobtail squid Euprymna parva (Cephalopoda: Sepiolidae). Journal of Experimental Marine Biology and Ecology 530-531, 151442.
- Drerup, C., Jackson, A., Rickard, C., Skea, M. & Cooke, G.M. (2021) Field observations on the behavioural ecology of the stout bobtail squid Rossia macrosoma (Cephalopoda: Sepiolidae) from Scottish waters. Marine Biodiversity 51, 57.
- Fernández–Álvarez, FÁ, Sánchez, P. & Villanueva, R. (2021) Morphological and molecular assessments of bobtail squids (Cephalopoda: Sepiolidae) reveal a hidden history of biodiversity. Frontiers in Marine Science 7, 632261.
- Fidopiastis, P.M., Boletzky, S.v. & Ruby, E.G. (1998) A new niche for Vibrio logei, the predominant light organ symbiont of squids in the genus Sepiola. Journal of Bacteriology 180, 59–64.
- Fisher, D.N., James, A., Rodríguez-Muñoz, R. & Tregenza, T. (2015) Behaviour in captivity predicts some aspects of natural behaviour, but not others, in a wild cricket population. Proceedings of the Royal Society B: Biological Sciences 282, 20150708.
- Forsythe, J.W. & Hanlon, R.T. (1988) Effect of temperature on laboratory growth, reproduction and life span of Octopus bimaculoides. Marine Biology 98, 369–379.
- Franklin, A. & Stuart-Fox, D. (2017) Single and multiple mating reduces longevity of female dumpling squid (Euprymna tasmanica). Journal of Evolutionary Biology 30, 977–984.
- Franklin, A.M., Squires, Z.E. & Stuart-Fox, D. (2012) The energetic cost of mating in a promiscuous cephalopod. Biology Letters 8, 754–756.
- Franklin, A.M., Squires, Z.E. & Stuart-Fox, D. (2014) Does predation risk affect mating behavior? An experimental test in dumpling squid (Euprymna tasmanica). PLoS One 9, e115027.
- Freitas, T.B.N., Leite, T.S., de Ramos, B., di Cosmo, A. & Proietti, M.C. (2022) In an octopus's garden in the shade: Underwater image analysis of litter use by benthic octopuses. Marine Pollution Bulletin 175, 113339.
- Gabel-Deickert, A. (1995) Reproductive patterns in Sepiola affinis and other Sepiolidae (Mollusca, Cephalopoda). Bulletin de l'Institut océanographique de Monaco 16, 73–83.
- Golikov, A.V., Ceia, F.R., Sabirov, R.M., Batalin, G.A., Blicher, M.E., Gareev, B.I., Gudmundsson, G., Jørgensen, L.L., Mingazov, G.Z., Zakharov, D.V. & Xavier, J.C. (2020) Diet and life history reduce interspecific and intraspecific competition among three sympatric Arctic cephalopods. Scientific Reports 10, 21506.
- Golikov, A.V., Ceia, F.R., Sabirov, R.M., Belyaev, A.N., Blicher, M.E., Arboe, N.H., Zakharov, D.V. & Xavier, J.C. (2019) Food spectrum and trophic position of an Arctic cephalopod, Rossia palpebrosa (Sepiolida), inferred by stomach contents and stable isotope (δ13C and δ15N) analyses. Marine Ecology Progress Series 632, 131–144.
- Goud, J., de Heij, A. & Hiemstra, A.-F. (2019) Cephalopods in the North Sea. Vita Malacologica 18, 34–57.
- Groenenberg, D.S., Goud, J., De Heij, A. & Gittenberger, E. (2009) Molecular phylogeny of north sea Sepiolinae (Cephalopoda: Sepiolidae) reveals an overlooked Sepiola species. Journal of Molluscan Studies 75, 361–369.
- Guerra, A., Rocha, F., González, ÁF & González, J.L. (2006) First observation of sand-covering by the lesser octopus Eledone cirrhosa. Iberus 24, 27–31.
- Hall, K. & Hanlon, R. (2002) Principal features of the mating system of a large spawning aggregation of the giant Australian cuttlefish Sepia apama (Mollusca: Cephalopoda). Marine Biology 140, 533–545.
- Hanlon, R.T. & Hixon, R.F. (1980) Body patterning and field observations of Octopus burryi Voss, 1950. Bulletin of Marine Science 30, 749–755.
- Hanlon, R.T. & Messenger, J.B. (1988) Adaptive coloration in young cuttlefish (Sepia officinalis L. the morphology and development of body patterns and their relation to behaviour. Philosophical Transactions of the Royal Society of London B: Biological Sciences 320, 437–487.
- Hanlon, R.T. & Messenger, J.B. (2018) Cephalopod Behaviour. Cambridge University Press, Cambridge.
- Hanlon, R.T., Ament, S.A. & Gabr, H. (1999) Behavioral aspects of sperm competition in cuttlefish, Sepia officinalis (Sepioidea: Cephalopoda). Marine Biology 134, 719–728.
- Hanlon, R.T., Claes, M.F., Ashcraft, S.E. & Dunlap, P.V. (1997) Laboratory culture of the sepiolid squid Euprymna scolopes: A model system for bacteria-animal symbiosis. The Biological Bulletin 192, 364–374.
- Hanlon, R.T., Conroy, L.-A. & Forsythe, J.W. (2008) Mimicry and foraging behaviour of two tropical sand-flat octopus species off North Sulawesi, Indonesia. Biological Journal of the Linnean Society 93, 23–38.
- Hanlon, R.T., Watson, A.C. & Barbosa, A. (2010) A “mimic octopus” in the Atlantic: Flatfish mimicry and camouflage by Macrotritopus defilippi. The Biological Bulletin 218, 15–24.
- Harman, R.F. & Seki, M.P. (1990) Iridoteuthis iris (Cephalopoda: Sepiolidae): new records from the Central North Pacific and first description of the adults. Pacific Science 44, 171–179.
- Hartmann, B., Lee, P.N., Kang, Y.Y., Tomarev, S., de Couet, H.G. & Callaerts, P. (2003) Pax6 in the sepiolid squid Euprymna scolopes: evidence for a role in eye, sensory organ and brain development. Mechanisms of Development 120, 177–183.
- Herring, P.J., Clarke, M.R., Boletzky, S.v. & Ryan, K.P. (1981) The light organs of Sepiola atlantica and Spirula spirula (Mollusca: Cephalopoda): Bacterial and intrinsic systems in the order Sepioidea. Journal of the Marine Biological Association of the United Kingdom 61, 901–916.
- Hoving, H.J.T., Laptikhovsky, V., Piatkowski, U. & Önsoy, B. (2008) Reproduction in Heteroteuthis dispar (Rüppell, 1844) (Mollusca: Cephalopoda): a sepiolid reproductive adaptation to an oceanic lifestyle. Marine Biology 154, 219–230.
- Hoving, H.J.T., Nauwelaerts, S., Van Genne, B., Stamhuis, E.J. & Zumholz, K. (2009) Spermatophore implantation in Rossia moelleri Steenstrup, 1856 (Sepiolidae; Cephalopoda). Journal of Experimental Marine Biology and Ecology 372, 75–81.
- Howard, R.B., Lopes, L.N., Lardie, C.R., Perez, P.P. & Crook, R.J. (2019) Early-life injury produces lifelong neural hyperexcitability, cognitive deficit and altered defensive behaviour in the squid Euprymna scolopes. Philosophical Transactions of the Royal Society B: Biological Sciences 374, 20190281.
- Jastrebsky, R.A., Bartol, I.K. & Krueger, P.S. (2017) Turning performance of brief squid Lolliguncula brevis during attacks on shrimp and fish. The Journal of Experimental Biology 220, 908–919.
- Jecklin, L. (1934) Beitrag zur Kenntnis der Laichgallerten und der Biologie der Embryonen decapoder Cephalopoden. Revue Suisse de Zoologie 41, 595–673.
- Jones, B.W. & Nishiguchi, M.K. (2004) Counterillumination in the Hawaiian bobtail squid, Euprymna scolopes Berry (Mollusca: Cephalopoda). Marine Biology 144, 1151–1155.
- Jones, N.J.E. & Richardson, C.A. (2010) Laboratory culture, growth, and the life cycle of the little cuttlefish Sepiola atlantica (Cephalopoda: Sepiolidae). Journal of Shellfish Research 29, 241–246.
- Jones, N.J.E. & Richardson, C.A. (2012) Distribution and reproductive biology of the little cuttlefish Sepiola atlantica (Cephalopoda: Sepiolidae) around Anglesey, North Wales. Helgoland Marine Research 66, 233–242.
- Kerbl, A., Handschuh, S., Nödl, M.-T., Metscher, B., Walzl, M. & Wanninger, A. (2013) Micro-CT in cephalopod research: Investigating the internal anatomy of a sepiolid squid using a non-destructive technique with special focus on the ganglionic system. Journal of Experimental Marine Biology and Ecology 447, 140–148.
- Kier, W. & van Leeuwen, J.L. (1997) A kinematic analysis of tentacle extension in the squid Loligo pealei. Journal of Experimental Biology 200, 41–53.
- Kousteni, V., Karachle, P., Megalofonou, P. & Lefkaditou, E. (2018) Cephalopod prey of two demersal sharks caught in the Aegean Sea (eastern Mediterranean). Journal of the Marine Biological Association of the United Kingdom 98, 81–88.
- Kubodera, T. & Okutani, T. (2002) A new species of bobtail squid, Euprymna megaspadicea, from Okinawa, Japan. Venus (Journal of the Malacological Society of Japan) 61, 159–168.
- Kubodera, T. & Okutani, T. (2011) New additions of luminous bobtail squids to the Japanese cephalopod fauna (Sepiolida: Sepiolidae: Heteroteuthinae). Venus (Journal of the Malacological Society of Japan) 69, 145–161.
- Kubodera, T., Okutani, T. & Kosuge, T. (2009) A new bobtail squid ex-pisce from Yonaguni Island, Southwestern Japan (Cephalopoda: Sepiolidae). Venus (Journal of the Malacological Society of Japan) 67, 173–179.
- Laptikhovsky, V., Allcock, A.L., Barnwall, L., Barrett, C., Cooke, G., Drerup, C., Firmin, C., Lozach, S., MacLeod, E., Oesterwind, D., Petroni, M., Robin, J.-P., Sheerin, E., Power, A.-M. & Pierce, G.J. (2022) Spatial and temporal variability of spawning and nursery grounds of Loligo forbesii and Loligo vulgaris squids in ecoregions of Celtic Seas and Greater North Sea. ICES Journal of Marine Science (published corrected proof, doi:10.1093/icesjms/fsac128).
- Laptikhovsky, V., Cooke, G., Barrett, C., Lozach, S., MacLeod, E., Oesterwind, D., Sheerin, E., Petroni, M., Barnwall, L., Robin, J.-P., Allcock, L. & Power, A.M. (2021) Identification of benthic egg masses and spawning grounds in commercial squid in the English Channel and Celtic Sea: Loligo vulgaris vs L. forbesii. Fisheries Research 241, 106004.
- Laptikhovsky, V., Nigmatullin, C.M., Hoving, H., Onsoy, B., Salman, A., Zumholz, K. & Shevtsov, G. (2008) Reproductive strategies in female polar and deep-sea bobtail squid genera Rossia and Neorossia (Cephalopoda: Sepiolidae). Polar Biology 31, 1499–1507.
- Lee, P.N., Callaerts, P. & de Couet, H.G. (2009a) The embryonic development of the hawaiian bobtail squid (Euprymna scolopes). Cold Spring Harbor Protocols 4, 1426–1435.
- Lee, P.N., McFall-Ngai, M.J., Callaerts, P. & de Couet, H.G. (2009b) The Hawaiian bobtail squid (Euprymna scolopes): a model to study the molecular basis of eukaryote-prokaryote mutualism and the development and evolution of morphological novelties in cephalopods. Cold Spring Harbor Protocols 11, 1–9.
- Lefkaditou, E. & Kaspiris, P. (1998) Distribution and reproductive biology of Sepietta neglecta (Naef, 1916) (Cephalopoda: Sepioidea) in the North Aegean Sea (Eastern Mediterranean). Veliger 41, 239–242.
- Leisman, G., Cohn, D.H. & Nealson, K.H. (1980) Bacterial origin of luminescence in marine animals. Science 208, 1271–1273.
- Levy, F. (1912) Observations sur les Sepioles des côtes de France. Archives de Zoologie Experimentale et Générale series 5(9), 54–59.
- Lu, C.C. & Okutani, T. (2022) Two new genera and species of sepioline squids (Cephalopoda: Sepiolidae) from Australia. Memoirs of Museum Victoria 81, 1–23.
- Mandel, M.J. & Dunn, A.K. (2016) Impact and influence of the natural Vibrio-squid symbiosis in understanding bacterial-animal interactions. Frontiers in Microbiology 7, 1982.
- Mangold-Wirz, K. (1963) Biologie des céphalopodes bentiques et nectoniques de la Mer Catalan. Vie et Milieu 13, 1–285.
- Mangold, K. & Froesch, D. (1977) A reconsideration of factors associated with sexual maturation. Symposia of the Zoological Society of London 38, 541–555.
- Mangold, K., Young, R. & Smith, C. (1998) Research note: In search of Rossia pacifica diegensis S.S. Berry, 1912. American Malacological Bulletin 14, 185–187.
- Mather, J.A. (1986) Sand digging in Sepia officinalis: Assessment of a cephalopod mollusc's "fixed" behavior pattern. Journal of Comparative Psychology 100, 315–320.
- Mauris, E. (1989) Colour patterns and body postures related to prey capture in Sepiola affinis (Mollusca: Cephalopoda). Marine Behaviour and Physiology 14, 189–200.
- McAnulty, S.J. & Nyholm, S.V. (2017) The role of hemocytes in the Hawaiian bobtail squid, Euprymna scolopes: a model organism for studying beneficial host-microbe interactions. Frontiers in Microbiology 7, 2013.
- McFall-Ngai, M. & Montgomery, M.K. (1990) The anatomy and morphology of the adult bacterial light organ of Euprymna scolopes Berry (Cephalopoda:Sepiolidae). The Biological Bulletin 179, 332–339.
- McFall-Ngai, M., Ruby, E. & Gilbert, J.A. (2021) Getting the message out: the many modes of host-symbiont communication during early-stage establishment of the squid-Vibrio partnership. mSystems 6(5), e00867–00821.
- Mercer, M.C. (1968) Systematics and biology of the sepiolid squids of the genus Rossia Owen, 1835 in Canadian waters with a preliminary review of the genus. M.Sc. thesis, Memorial University of Newfoundland.
- Messenger, J.B. (1968) The visual attack of the cuttlefish, Sepia officinalis. Animal Behaviour 16, 342–357.
- Moynihan, M. (1983) Notes on the behavior of Euprymna scolopes (Cephalopoda: Sepiolidae). Behaviour 85, 25–41.
- Nabhitabhata, J., Nilaphat, P., Promboon, P. & Jaroongpattananon, C. (2005) Life cycle of cultured bobtail squid, Euprymna hyllebergi Nateewathana, 1997. Phuket Marine Biological Center Research Bulletin 66, 351–365.
- Nesis, K.N. (1993) Cephalopods of seamounts and submarine ridges. In: Okutani, T., O'Dor, R.K. & Kubodera, T. (Eds), The Recent Advances in Cephalopod Fishery Biology. Tokai University Press, Tokyo, pp. 365–373.
- Nödl, M.-T., Kerbl, A., Walzl, M.G., Müller, G.B. & de Couet, H.G. (2016) The cephalopod arm crown: appendage formation and differentiation in the Hawaiian bobtail squid Euprymna scolopes. Frontiers in Zoology 13, 44.
- Norman, M. & Lu, C. (1997) Redescription of The southern dumpling Squid euprymna tasmanica and a revision of the genus Euprymna (Cephalopoda: Sepiolidae). Journal of the Marine Biological Association of the United Kingdom 77, 1109–1137.
- Nyholm, S.V. & McFall-Ngai, M.J. (2021) A lasting symbiosis: how the Hawaiian bobtail squid finds and keeps its bioluminescent bacterial partner. Nature Reviews Microbiology 19, 666–679.
- Okutani, T. & Tsuchida, S. (2005) Occurrence and living habit of bathyal bobtail squid, Heteroteuthis hawaiiensis (Cephalopoda: Sepiolidae) from off the Ogasawara Islands, Japan. Venus (Journal of the Malacological Society of Japan) 63, 125–133.
- Okutani, T. & Sasaki, T. (2007) Eggs of Rossia mollicella (Cephalopoda: Sepiolidae) deposited in a deep-sea sponge. Journal of Molluscan Studies 73, 287–289.
- Olmos-Pérez, L., Roura, Á, Pierce, G.J. & González, ÁF (2018) Sepiolid paralarval diversity in a regional upwelling area of the NE Atlantic. Hydrobiologia 808, 57–70.
- Önsoy, B., Ceylan, B. & Salman, A. (2013) Reproductive biology of the lentil bobtail squid, Rondeletiola minor(Cephalopoda: Sepiolidae) from the eastern Mediterranean. Journal of the Marine Biological Association of the United Kingdom 93, 851–854.
- Önsoy, B., Laptikhovsky, V. & Salman, A. (2008) Reproductive biology of the Patagonian bobtail squid, Semirossia patagonica (Sepiolidae: Rossiinae) in the south-west Atlantic. Journal of the Marine Biological Association of the United Kingdom 88, 1019–1023.
- Orsi Relini, L. (1995) Notes on midwater collections of Heteroteuthis dispar (Cephalopoda, Sepiolidae). Bulletin de l'Institut océanographique de Monaco 16, 63–72.
- Orsi Relini, L. & Massi, D. (1988) Feeding of Sepietta oweniana (d’Orbigny 1839) along the slope of the Ligurian Sea: a preliminary note. Rapport Commission International Mer Méditerranée 31, 255.
- Racovitza, E.G. (1894) Sur l'accouplement de quelques Céphalopodes, Sepiola rondeletii (Leach), Rossia macrosoma (d. Ch.) et Octopus vulgaris (Lam.). Gauthier-Villars et Fils, Paris.
- Reid, A. (1991) Taxonomic review of the Australian Rossiinae (Cephalopoda: Sepiolidae), with a description of a new species, Neorossia leptodons, and redescription of N. caroli (Joubin, 1902). Bulletin of Marine Science 49, 748–831.
- Reid, A.L. (2021) Two new species of Iridoteuthis (Cephalopoda: Sepiolidae: Heteroteuthinae) from the southwest Pacific, with a redescription of Stoloteuthis maoria (Dell, 1959). Zootaxa 5005, 503–537.
- Reid, A. & Jereb, P. (2005) Family Sepiolidae. In: Jereb, P. & Roper, C.F. (Eds), Cephalopods of the World. an Annotated and Illustrated Catalogue of Cephalopod Species Known to Date. Volume 1. Chambered Nautiluses and Sepioids (Nautilidae, Sepiidae, Sepiadariidae, Idiosepiidae and Spirulidae). FAO, Rome, pp. 153–212.
- Ringvold, H. & Taite, M. (2018) Using citizen science to obtain data on large, floating gelatinous spheres from NE Atlantic, attributed to egg mass of ommastrephid squid (Oegopsida, Cephalopoda, Mollusca). Marine Biology Research 14, 672–681.
- Ringvold, H., Taite, M., Allcock, A.L., Vecchione, M., Péan, M., Sandulli, R., Johnsen, G., Fjellheim, A., Bakke, S., Sannæs, H., Synnes, A.-E.W., Coronel, J., Hansen, M., Olejar, P.G., Eliassen, G., Eliassen, A. & Klungland, K. (2021) In situ recordings of large gelatinous spheres from NE Atlantic, and the first genetic confirmation of egg mass of Illex coindetii (Vérany, 1839) (Cephalopoda, Mollusca). Scientific Reports 11, 7168.
- Rocha, F., Guerra, Á & González, ÁF (2001) A review of reproductive strategies in cephalopods. Biological Reviews of the Cambridge Philosophical Society 76, 291–304.
- Rodrigues, M., Garci, M.E., Guerra, Á & Troncoso, J.S. (2009) Mating behavior of the Atlantic bobtail squid Sepiola atlantica (Cephalopoda: Sepiolidae). Vie et Milieu 59, 271–275.
- Rodrigues, M., Garci, M.E., Troncoso, J.S. & Guerra, A. (2010) Burying behaviour in the bobtail squid Sepiola atlantica (Cephalopoda: Sepiolidae). Italian Journal of Zoology 77, 247–251.
- Rodrigues, M., Garci, M.E., Troncoso, J.S. & Guerra, A. (2011a) Seasonal abundance of the Atlantic bobtail squid Sepiola atlantica in Galician waters (NE Atlantic). Marine Biology Research 7, 812–819.
- Rodrigues, M., Garcí, M.E., Troncoso, J.S. & Guerra, Á (2011b) Spawning strategy in Atlantic bobtail squid Sepiola atlantica (Cephalopoda: Sepiolidae). Helgoland Marine Research 65, 43–49.
- Rodrigues, M., Guerra, Á & Troncoso, J.S. (2011c) The embryonic phase and its implication in the hatchling size and condition of Atlantic bobtail squid Sepiola atlantica. Helgoland Marine Research 65, 211–216.
- Rodrigues, M., Guerra, A. & Troncoso, J.S. (2012) Reproduction of the Atlantic bobtail squid Sepiola atlantica (Cephalopoda: Sepiolidae) in northwest Spain. Invertebrate Biology 131, 30–39.
- Roper, C.F. (1974) Vertical and seasonal distribution of pelagic cephalopods in the Mediterranean Sea. Preliminary report. Bulletin of the American Malacological Union, 27–30.
- Rosa, R., Pereira, J., Costa, P. & Nunes, M. (2006) Distribution, abundance, biology and biochemistry of the stout bobtail squid Rossia macrosoma from the Portuguese coast and. Marine Biology Research 2, 14–23.
- Roura, Á, Antón Álvarez-Salgado, X., González, ÁF, Gregori, M., Rosón, G., Otero, J. & Guerra, Á (2016) Life strategies of cephalopod paralarvae in a coastal upwelling system (NW Iberian Peninsula): insights from zooplankton community and spatio-temporal analyses. Fisheries Oceanography 25, 241–258.
- Ruby, E.G. (1996) Lessons from a cooperative, bacterial-animal association: the Vibrio fischeri–Euprymna scolopes light organ symbiosis. Annual Review of Microbiology 50, 591–624.
- Salman, A. (1998) Reproductive biology of Sepietta oweniana (Pfeffer, 1908) (Sepiolidae: Cephalopoda) in the Aegean Sea. Scientia Marina 62, 379–383.
- Salman, A. (2010) Reproductive biology of Neorossia caroli (Cephalopoda: Sepiolidae) in the Aegean Sea. Scientia Marina 75, 9–15.
- Salman, A. (2014) Reproductive biology of Sepiola intermedia (Sepiolidae: Cephalopoda) in the Aegean Sea, Eastern Mediterranean. Turkish Journal of Fisheries and Aquatic Sciences 14, 777–784.
- Salman, A. & Katagan, T. (1996) A preliminary study on reproduction biology of Rondeletiola minor (Naef, 1912) (Sepiolidae: Cephalopoda) in the Aegean Sea. E Ü Su Ürünleri dergisi 13, 403–408.
- Salman, A. & Önsoy, B. (2004) Analysis of fecundity of some bobtail squid of the genus Sepiola (Cephalopoda: Sepiolida) in the Aegean Sea (eastern Mediterranean). Journal of the Marine Biological Association of the United Kingdom 84, 781–782.
- Salman, A. & Önsoy, B. (2010) Reproductive biology of the bobtail squid Rossia macrosoma (Cephalopoda: Sepiolidea) from the eastern Mediterranean. Turkish Journal of Fisheries and Aquatic Sciences 10, 81–86.
- Sanchez, G., Fernández-Álvarez, FÁ, Taite, M., Sugimoto, C., Jolly, J., Simakov, O., Marlétaz, F., Allcock, L. & Rokhsar, D.S. (2021) Phylogenomics illuminates the evolution of bobtail and bottletail squid (order Sepiolida). Communications Biology 4, 819.
- Sanchez, G., Jolly, J., Reid, A., Sugimoto, C., Azama, C., Marlétaz, F., Simakov, O. & Rokhsar, D.S. (2019) New bobtail squid (Sepiolidae: Sepiolinae) from the Ryukyu islands revealed by molecular and morphological analysis. Communications Biology 2, 465.
- Seehafer, K., Brophy, S., Tom, S.R. & Crook, R.J. (2018) Ontogenetic and experience-dependent changes in defensive behavior in captive-bred Hawaiian bobtail squid, Euprymna scolopes. Frontiers in Physiology 9, 299.
- Seibel, B., Robison, B. & Haddock, S. (2005) Post-spawning egg care by a squid. Nature 438, 929.
- Shears, J. (1988) The use of a sand-coat in relation to feeding and diel activity in the sepiolid squid Euprymna scolopes. Malacologia 29, 121–133.
- Shimek, R. (1983) Escape behavior of Rossia pacifica Berry, 1911. American Malacological Bulletin 2, 91–92.
- Singley, C. (1982) Histochemistry and fine-structure of the ectodermal epithelium of the sepiolid squid Euprymna scolopes. Malacologia 23, 177–192.
- Singley, C. (1983) Euprymna scolopes. In: Boyle, P.R. (Ed), Cephalopod Life Cycles, Vol. I: Species Accounts. Academic Press, London, pp. 69–74.
- Sinn, D.L. & Moltschaniwskyj, N.A. (2005) Personality traits in dumpling squid (Euprymna tasmanica): context-specific traits and their correlation with biological characteristics. Journal of Comparative Psychology 119, 99–110.
- Sinn, D.L., Gosling, S.D. & Moltschaniwskyj, N.A. (2008) Development of shy/bold behaviour in squid: context-specific phenotypes associated with developmental plasticity. Animal Behaviour 75, 433–442.
- Sinn, D.L., Apiolaza, L.A. & Moltschaniwskyj, N.A. (2006) Heritability and fitness-related consequences of squid personality traits. Journal of Evolutionary Biology 19, 1437–1447.
- Squires, Z.E., Norman, M.D. & Stuart-Fox, D. (2013) Mating behaviour and general spawning patterns of the southern dumpling squid Euprymna tasmanica (Sepiolidae): a laboratory study. Journal of Molluscan Studies 79, 263–269.
- Squires, Z.E., Wong, B.B., Norman, M.D. & Stuart-Fox, D. (2012) Multiple fitness benefits of polyandry in a cephalopod. PLoS One 7, e37074.
- Squires, Z.E., Wong, B.B., Norman, M.D. & Stuart-Fox, D. (2014) Multiple paternity but no evidence of biased sperm use in female dumpling squid Euprymna tasmanica. Marine Ecology Progress Series 511, 93–103.
- Squires, Z.E., Wong, B.B., Norman, M.D. & Stuart-Fox, D. (2015) Last male sperm precedence in a polygamous squid. Biological Journal of the Linnean Society 116, 277–287.
- Stabb, E.V. (2006) The Vibrio fischeri–Euprymna scolopes light organ symbiosis. In: Thompson, F.L., Austin, B. & Swings, J. (Eds), The Biology of Vibrios. American Society of Microbiology, Washington, DC, pp. 204–218.
- Stabb, E.V. & Millikan, D.S. (2009) Is the Vibrio fischeri–Euprymna scolopes symbiosis a defensive mutualism? In: White Jr, J.F. & Torres, M.S. (Eds), Defensive Mutualism in Microbial Symbiosis. CRC Press, Boca Raton, pp. 85–98.
- Steer, M.A., Moltschaniwskyj, N.A., Nichols, D.S. & Miller, M. (2004) The role of temperature and maternal ration in embryo survival: using the dumpling squid Euprymna tasmanica as a model. Journal of Experimental Marine Biology and Ecology 307, 73–89.
- Summers, W.C. (1985) Ecological implications of life stage timing determined from the cultivation of Rossia pacifica (Mollusca: Cephalopoda). Vie et Milieu 35, 249–254.
- Summers, W.C. & Colvin, L. (1989) On the cultivation of Rossia pacifica (Berry, 1911). Journal of Cephalopod Biology 1, 21–32.
- Thorson, G. (1946) Reproduction and larval development of Danish marine bottom invertebrates. Meddelelser Fra Kommissionen for Danmarks Fiskeri - og Havundersogelser, Series: Plankton 4, 1–523.
- Verma, S.C. & Miyashiro, T. (2013) Quorum sensing in the squid-Vibrio symbiosis. International Journal of Molecular Sciences 14, 16386–16401.
- Villanueva, R. (1992) Deep-sea cephalopods of the north-western Mediterranean: indications of up-slope ontogenetic migration in two bathybenthic species. Journal of Zoology 227, 267–276.
- Villanueva, R. & Sánchez, P. (1993) Cephalopods of the Benguela Current off Namibia: new additions and considerations on the genus Lycoteuthis. Journal of Natural History 27, 15–46.
- Visick, K.L., Stabb, E.V. & Ruby, E.G. (2021) A lasting symbiosis: how Vibrio fischeri finds a squid partner and persists within its natural host. Nature Reviews Microbiology 19, 654–665.
- von Byern, J. & Klepal, W. (2006) Adhesive mechanisms in cephalopods: a review. Biofouling 22, 329–338.
- von Byern, J., Kerbl, A., Nödl, M.T., Bello, G., Staedler, Y., Schönenberger, J. & Cyran, N. (2016) Spine formation as a hatching tool in Euprymna scolopes (Mollusca, Cephalopoda, Sepiolidae). Malacologica 59, 231–238.
- von Byern, J., Cyran, N., Klepal, W., Nödl, M.T. & Klinger, L. (2017) Characterization of the adhesive dermal secretion of Euprymna scolopes Berry, 1913 (Cephalopoda). Zoology 120, 73–82.
- Wada, T., Takegaki, T., Mori, T. & Natsukari, Y. (2005) Sperm displacement behavior of the cuttlefish Sepia esculenta (Cephalopoda: Sepiidae). Journal of Ethology 23, 85–92.
- Wild, E., Wollesen, T., Haszprunar, G. & Heß, M. (2015) Comparative 3D microanatomy and histology of the eyes and central nervous systems in coleoid cephalopod hatchlings. Organisms Diversity & Evolution 15, 37–64.
- Wood, J.B. & O'Dor, R.K. (2000) Do larger cephalopods live longer? Effects of temperature and phylogeny on interspecific comparisons of age and size at maturity. Marine Biology 136, 91–99.
- Yau, C. & Boyle, P. (1996) Ecology of Sepiola atlantica (Mollusca: Cephalopoda) in the shallow sublittoral zone. Journal of the Marine Biological Association of the United Kingdom 76, 733–748.
- Young, R.E. & Roper, C.F.E. (1976) Bioluminescent countershading in midwater animals: evidence from living squid. Science 191, 1046–1048.
- Young, R.E., Vecchione, M. & Roper, C.F.E. (2007) A new genus and three new species of decapodiform cephalopods (Mollusca: Cephalopoda). Reviews in Fish Biology and Fisheries 17, 353–365.
- Zepeda, E.A., Veline, R.J. & Crook, R.J. (2017) Rapid associative learning and stable long-term memory in the squid Euprymna scolopes. The Biological Bulletin 232, 212–218.
- Zumholz, K. & Frandsen, R.P. (2006) New information on the life history of cephalopods off west Greenland. Polar Biology 29, 169–178.