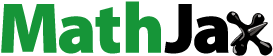
ABSTRACT
The blacklip rock oyster, Saccostrea lineage J, has the potential to support new aquaculture developments throughout its range in the tropical Indo-Pacific region, but lack of research investment in understanding basic biology has been a key failure point in the past. This study assessed Saccostrea lineage J spat growth and survival in downweller and upweller nursery systems, with the aim of establishing baseline biological information and evaluating spat performance to support commercialisation efforts. Downweller and upweller nursery systems were similarly effective at rearing spat, with mean dorsoventral measurement in both systems reaching a 5 mm deployment size at 59 days post hatch. Growth rates reported in this study of 0.19 and 0.20 mm day−1 in the downweller and upweller, respectively, are comparable to other hatchery-produced tropical and subtropical bivalve spat. Based on the results of this study, it is recommended that spat be held in nurseries for approximately two months to reach a 5 mm deployment size. The information obtained in this study is the first documentation of Saccostrea lineage J spat growth and survival, and the methods used provide a basis for commercial production of this species.
Introduction
Although rock oysters are fished and farmed throughout the tropics, there is a paucity of literature surrounding much of their basic biology (Nowland et al. Citation2019b; Willer and Aldridge Citation2020). Documented reasons for this include the low socio-economic status of most tropical countries, taxonomic confusion of culture species, lack of provision of adequate public sector resourcing and support services and limited private sector investment (Sachs Citation2001; SOTT Citation2017; Nowland et al. Citation2019b). Existing biological knowledge for most tropical rock oyster species is generally inadequate to support commercial-scale production, resulting in a bottleneck in industry progress. For example, the lack of essential information about suitable growout sites and conditions for the native mangrove oyster, Crassostrea rhizophorae (Guilding 1828), is impeding developments in Cuba (Mazón-Suástegui et al. Citation2017). Further, Kinch et al. (Citation2020) report on the opportunities and market potential of oyster aquaculture in Fiji, acknowledging that increasing productivity will require major investments in research, development and extension.
A lack of research investment in understanding basic biology has been a key failure point in past programs to develop the tropical blacklip rock oyster, Saccostrea lineage J, as a commercial aquaculture species. For example, several Pacific Island countries, including Palau and New Caledonia, attempted to farm Saccostrea lineage J in the 1980s (Coeroli et al. Citation1984; Glude Citation1984). Around the same time in Australia, a small farm was established on Magnetic Island and an experimental farm developed at Palm Island in Queensland (Bryson Citation1977; White Citation1991). All farms were eventually closed and an unreliable and insufficient supply of wild spat was recognised as the major bottleneck. Hatchery production of this species was first attempted by Coeroli et al. (Citation1984) with limited success. Later, Southgate and Lee (Citation1998) confirmed that Saccostrea lineage J were amenable to hatchery production. It was not until recently that significant time and resources were allocated to understanding the basic biology of Saccostrea lineage J, with the aim of developing reliable hatchery culture methods (Nowland et al. Citation2018a; Nowland et al. Citation2019c; Nowland and Hartley Citation2021).
Research has focused on closing the life cycle in the hatchery to support commercial spat production for growout (Nowland et al. Citation2018b; Nowland et al. Citation2019a). Despite a growing body of literature on Saccostrea lineage J, there are no existing publications on nursery or growout performance. Once larvae settle into spat, they are typically transferred to a nursery system until they have reached a dorsoventral measurement (DVM) of at least 5 mm, depending on farm requirements, before they are deployed into ocean-based farms (Gosling Citation2003). Maintaining spat in nursery systems is an important step in the production cycle, as early deployment onto farms can lead to high mortalities from sediment smothering and predation (Gosling Citation2003). Information on oyster spat performance in commercial nursery systems is essential for producers to make economic decisions about spat age and size at deployment. Therefore, this study assessed Saccostrea lineage J spat growth and survival in downweller and upweller nursery systems with the aim of establishing baseline biological information and evaluating spat performance to support commercialisation of this emerging aquaculture species.
Materials and methods
Spawning and larval culture
Larval production was conducted at the Northern Territory Government, Department of Industry, Tourism and Trades, Darwin Aquaculture Centre. Saccostrea lineage J broodstock oysters (n = 68) with anteroposterior and dorsoventral shell measurements of 67.87 ± 0.24 mm and 103.97 ± 0.36 mm, respectively, were collected from the intertidal zone at Groote Eylandt, Northern Territory, Australia (13°40′53.60″S, 136°36′41.40″E). Spawning and larval rearing followed hatchery protocols for Saccostrea lineage J described by Nowland and Hartley (Citation2021). When more than 35% of larvae reached the crawling pediveliger stage (17 days post hatch [dph]) and were able to be retained on a 220 µm nylon mesh screen, they were induced to settle using epinephrine bitartrate (Sigma-Aldrich, USA) as described by Nowland and Hartley (Citation2021). Spat were transferred to either a downweller or an upweller nursery system when they were large enough to be retained on a 500 µm nylon mesh screen.
Nursery systems
The downweller nursery system consisted of a 1400 L fibreglass raceway recirculating system and spray bar that can hold up to eight 50 cm diameter screens (A). A low voltage, 24 V submerged pump (REEFE Pumps, Australia) was used to circulate water through the system and the flow rate into each screen was 110 L h−1, governed by the spray nozzle size (jet spray full circle; POPE, Australia). The upweller system consisted of a 1400 L fibreglass raceway recirculating system with a gutter that can hold up to eight 50 cm diameter screens (B). Water was directed up through the table by two 230 V submerged pumps (U3KS special/2; Pentair, United Kingdom), and exited the screens via polyvinyl chloride (PVC) pipe into a drain. The flow rate through each screen was approximately 25 L min−1, which was high enough to promote screen cleaning but slow enough not to lift spat off the screen. A backup screen was placed over the PVC pipe outlet to prevent escapes.
Seawater was pumped via submerged pumps into the hatchery through a primary bank of 12 high-volume, deep-bed sand filters with approximately 98% efficiency for the removal of particles of >10 μm (GEBEL AQUASAFE, Australia). For larval rearing 36 ppt seawater was blended with carbon filtered de-chlorinated freshwater to adjust salinity to 25 ppt. In the nursery system, seawater temperature was ambient and salinity was gradually increased, by 1 ppt day−1, from the larval rearing salinity of 25 ppt to the ambient salinity of 36 ppt. A series of 50 cm diameter screens were maintained fitted with either 150 or 500 μm nylon mesh, with an initial stocking density of 10,000 spat screen−1 (5 spat per cm2). Spat on the screens and the systems were rinsed with fresh water daily. Three times a week spat were removed from the tank systems before the systems were thoroughly cleaned with 200 ppm chlorine solution. At this stage, spat are considered large enough to be left out of water during cleaning (typically 1 h).
Microalgae
Larval feeding began approximately 18 h post fertilisation (hpf), on the morning after spawning. D-stage larvae were fed a 2:1:1 mixture of Chaetoceros calcitrans (Takano 1968), Tisochrysis lutea (Bendif and Probert 2013), and Pavlova sp. (CS50, CSIRO catalog code). Chaetoceros muelleri (Lemmermann 1898) was gradually incorporated into the diet from days 8 to 16 until a feed ratio of 1:1:1:2 of C. calcitrans, T. lutea, Pavlova sp., and C. muelleri was established. The daily ration was 15,000 cells mL−1 from 1 to 5 dph, 20,000 cells mL−1 on 6 dph, 25,000 cells mL−1 from 7 to 9 dph and 30,000 cells mL−1 from 10 to 21 dph. Rations were split between morning and afternoon feeds. Food rations in the downweller settlement system continued according to the larval culture rations at 30,000 cells mL−1, as larvae/spat undergoing metamorphosis temporarily stabilise or reduce feeding.
Spat in the nursery system were fed a 2:2:5:1 mixture of T. lutea, Pavlova sp., C. muelleri and Tetraselmis chuii (Butcher 1959). Feeding rates started at 100,000 cells mL−1 d−1 at 31 dph and gradually increased to 800,000 cells mL−1 d−1 by 93 dph. Increases in food ration were non-linear as they were based on the rate of algal consumption. Residual algae cells were assessed and recorded daily using an AquaFluor handheld fluorometer (Turner Designs, USA). If the readings were below 40,000 cells mL−1, then algae food ration was increased. The required ration of each microalgae species was calculated on an equal dry-weight basis.
Data collection and seawater parameters
Data collection occurred from the time when spat were transferred from the settlement system into the nursery systems at 31 dph, until 92 dph when they reached ∼5 mm DVM and were translocated to a growout farm. Seawater parameters recorded daily included temperature, salinity, dissolved oxygen (DO) and pH. Grading was conducted weekly using a wet screen method, where spat were placed onto a desired grading screen size, rinsed with filtered sea water (FSW) and sieved gently (Nowland and Hartley Citation2021). The larger size grade was retained on the screen, whilst the smaller size grade passed through and was retained on a secondary back-up screen. Spat were stocked in the nursery systems on screens specific to their size grade. Spat growth was recorded three times a week by measuring the DVM; spat from all screens of each size grade, within each nursery system (downweller and upweller separately) were added to a tub and thoroughly mixed, and 10 spat were randomly selected and measured.
Each week following grading, biomass and survival of spat from each size grade in each system was assessed. Total volume was recorded by placing spat into a measuring cylinder with seawater and gently tapping the cylinder until the spat settled down to a packed volume. Total wet weight of all spat and wet weight of 100 spat was determined using electronic balances with precision of 0.1 and 0.001 g, respectively. Spat were placed into nylon mesh screens placed on paper towel for 5 min to remove as much water as possible, prior to weighting. These weights were used to estimate the total number of spat in each size grade and system. Survival was then estimated from the total number of spat remaining in the system:
(1)
(1)
Spat histology
Spat (n = 177) were sampled from both nursery systems and submitted for histological health assessment at 63 dph, as part of pre-movement health certification. Spat were fixed in 10% seawater formalin for 48 h and then processed and embedded in paraffin. Sections of 4 µm were made using standard histological techniques (Luna Citation1968; Raphael et al. Citation1976) and stained with haematoxylin and eosin for histological evaluation. Tissues examined generally included shell matrix, gill (ctenidia), gonad follicle, mantle, nerve ganglion, adductor muscle, labial palp, digestive gland, stomach, intestine, heart and nephridium. Sections were assessed microscopically and observations on spat gonadal differentiation and gametogenesis reported.
Statistical analyses
Data were tested for homogeneity of variance, and biomass and survival data were square root transformed. Data were then analysed with a one-way or a two-way analysis of variance (ANOVA) to determine whether any significant differences exist between nursery systems and spat age. Analyses were completed and plots generated using R with a significance level of α = 0.05 (R Core Team Citation2017).
Results
Seawater parameters
In the downweller and upweller nursery systems, temperature ranged from 22.6 to 31.4 °C (mean 26.6 ± 0.2 °C) and 24.6 to 31.4 °C (mean 28.8 ± 0.2 °C), respectively. Salinity was consistent between the two nursery systems and ranged from 29 to 35 ppt, with a mean of 32 ± 0.2 ppt. Percent DO ranged from 83.5 to 101.5% (mean 92.4 ± 0.9%) and 85.3 to 103% (mean 93.7 ± 0.9%) in the downweller and upweller systems, respectively, while pH ranged from 7.48 to 8.08 (mean 7.75 ± 0.02) and 7.60 to 8.15 (mean 8.01 ± 0.01) in the downweller and upweller systems, respectively. No significant differences (P > 0.05) were detected between the downweller and upweller nursery systems for salinity and DO. The downweller nursery system had significantly lower seawater temperature and pH than the upweller nursery system (F = 39.34; df 1/122; P < 0.05 and F = 156.2; df 1/122; P < 0.05, respectively).
Spat growth, survival and histology
Spat had an average growth rate in the downweller and upweller nursery systems of 0.19 ± 0.09 and 0.20 ± 0.10 mm day−1, respectively, and reached a mean DVM of 5 mm at ∼59 dph (). No significant differences were recorded in DVM or biomass between the nursery systems at each spat age ( and ). At deployment to growout farms (93 dph), 74.3% and 75.8% of spat had a DVM >5 mm in the downweller and upweller nursery systems, respectively. Throughout the nursery stage (31 to 93 dph) total spat biomass increased from 84.3 g to 1514.1 ± 201.2 g and 1362.3 ± 186.5 g, in the downweller and upweller nursery systems, respectively ().
Figure 2. Dorsoventral measurement (DVM; mm) of Saccostrea lineage J spat in a downweller and upweller nursery system, from 31 to 93 days post hatch (dph). Y-axis intercept line at 5 mm DVM indicates spat size suitable for deployment.
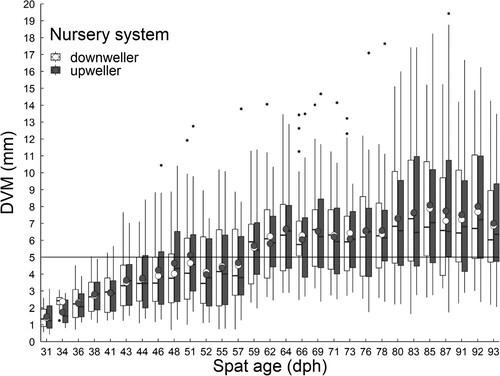
Figure 3. Total biomass (g) of Saccostrea lineage J spat in a downweller and upweller nursery system from 31 to 93 days post hatch (dph).
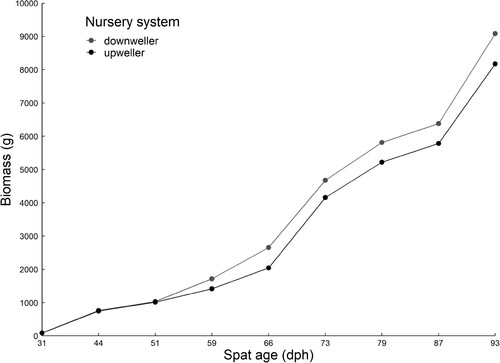
Mean spat weight and DVM for each grade size (nylon mesh size) is shown in and spat development at each grade size is shown photographically in . The volume of 1000 spat increased from 0.42 and 0.44 mL at a grade size of 0.5 mm, to 199.18 and 234.18 mL at a grade size of 9 mm, for the downweller and upweller nursery systems, respectively (). No significant differences were recorded in survival between the nursery systems at each spat age. Survival estimates ranged from 97 to 114% and 87 to 104%, in the downweller and upweller nursery systems, respectively. At 93 dph the total survival estimate from initial stocking was 97% and 92% in the downweller and upweller nursery systems, respectively.
Figure 4. Development photographs and mean dorsoventral shell measurement (DVM ± standard error, mm) of Saccostrea lineage J spat at grade sizes. A, 0.5 mm, 34 days post hatch (dph); B, 1 mm, 43 dph; C, 2 mm, 51 dph; D, 3 mm, 66 dph; E, 4 mm, 71 dph; F, 5 mm, 80 dph; and G, 9 mm, 90 dph.
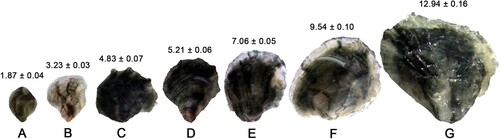
Table 1. Mean spat weight (g), spat dorsoventral measurement (DVM ± standard error (SE), mm) and volume of 1000 spat (mL) of Saccostrea lineage J spat from 31 to 93 days post hatch (dph), for each spat grade mesh size (mm) and nursery system.
Spat submitted for histological health assessment ranged in shell length from 1 to 7 mm and gonad follicles were identified in 19 spat sections; there was 1 female with vitellogenic oocytes, and there were 18 where gonad follicles were present but gender could not be determined (sex indeterminate).
Discussion
This study provides baseline biological information on hatchery-reared Saccostrea lineage J spat development. Downweller and upweller systems used in this study were similarly effective at rearing spat, with mean DVM in both systems reaching the 5 mm threshold size favoured for transfer to ocean-based growout systems at 59 dph. While growth rates reported in this study (0.19 and 0.20 mm day−1 in the downweller and upweller system, respectively) are broadly comparable to many other hatchery-produced tropical and subtropical bivalve spat, growth is highly variable among species, locations and individuals. Wang and Li (Citation2018) reported a maximum growth rate of 0.19 mm day−1 in the Iwagaki oyster, Crassostrea nippona (Seki 1934), while growth in the Cortez oyster, Crassostrea corteziensis (Hertlein 1951), ranges between 0.17 and 0.52 mm day−1 (Cáceres-Puig et al. Citation2007; Mazón-Suástegui et al. Citation2008) depending on diet and other environmental factors.
During larval settlement and early nursery stages oyster larvae are typically transferred into downweller systems. This is because downweller systems encourage settlement as larvae are pushed downwards onto screens (Shumway Citation2021). Most hatcheries transition spat into upweller systems as soon they are large enough to remain on the screen despite the upwards flow (Utting and Spencer Citation1991; Wallace et al. Citation2008). The supposed advantages of upweller systems include the removal of detritus material, higher stocking densities and easier maintenance (Shumway Citation2021), although few studies have directly compared downweller and upweller nursery systems. Pfeiffer and Rusch (Citation2001) conducted nursery comparison experiments on the northern quahog, Mercenaria mercenaria (Linnaeus 1758), under various culture systems related to flow type: stacked trays with downward flow, traditional upweller (i.e., low flow) and fluidised flow upweller (i.e., high flow). The fluidised flow conditions showed better growth than the downward flow and upward low flow rates (Pfeiffer and Rusch Citation2001).
The current study does not show significant differences in spat growth and survival between the downweller and upweller systems used. This may be due to the current study rearing spat to a deployment size of 5 mm. Acquafredda et al. (Citation2022) showed that for the Atlantic surf clam, Spisula solidissima (Dillwyn 1817), downweller and upweller nursery systems for small juveniles (size 0.4–2.7 mm) performed similarly, while the nursery upweller system for larger juveniles (size 1.1–18.0 mm) performed significantly better.
Growth and survival rates in farmed molluscs are influenced by a multitude of endogenous and exogenous factors, and performance during the early life history is often strongly and positively correlated with subsequent growth and thus growout time to attain market size (Videla et al. Citation1998; Collet et al. Citation1999). Developing protocols to optimise growth and survival in hatcheries is therefore a key priority in the development of new production species such as Saccostrea lineage J. Available strategies to increase growth and/or survival include optimising environmental conditions within hatcheries (Nell and Holliday Citation1988; Gjedrem and Rye Citation2018), triploidy (Wadsworth et al. Citation2019) and selective breeding programs (Hollenbeck and Johnston Citation2018). The presence of gonad follicles observed in 11% of the spat submitted for histological health assessment at 63 dph is of interest, and the first record for this species. This indicates short generation times, which are advantageous for possible future selective breeding. To maximise spat growth and hatchery yield for Saccostrea lineage J, the next steps should focus on optimising stocking densities, temperature, salinity and microalgae ration in nursery systems. The biological information presented in this study provides a baseline against which future progress may be measured.
Bivalves are often affected by high mortality rates during larval and early post-larval development in hatcheries (Dubert et al. Citation2017). In the current study, survival rates were high in both nursery systems (97% and 92% in the downweller and upweller nursery systems, respectively). While this highlights the amenability of Saccostrea lineage J hatchery production, survival is nonetheless likely to be negatively affected as the scale of hatchery production intensifies and stocking densities increase. Strategies to maintain high survival rates should remain a key priority of future research.
Our results indicate that both downweller and upweller nursery systems are suitable for rearing Saccostrea lineage J spat. Mean seawater temperatures were 2.2 °C cooler in the downweller system, due to the cooling effects of the spray bar on the water surface, and pH was also 0.26 units lower in the downweller system. This may be attributed to higher rates of algae settling on the screens in this system and subsequently higher levels of microbial activity. Given these differences, the optimal nursery system may vary among locations.
In conclusion, the methods used in this study support successful post-larval culture of Saccostrea lineage J and provide a basis for commercial production of this species. Based on the results of this study, it is recommended that spat be held in nurseries for approximately two months to reach a 5 mm deployment size. These results add to a growing body of evidence that Saccostrea lineage J is well suited to hatchery-based aquaculture production and has the potential to support new aquaculture developments throughout its range in the tropical Indo-Pacific region.
Acknowledgements
This study was conducted as part of the Cooperative Research Centre for Developing Northern Australia (CRCNA) project ‘Northern Australian Tropical Rock Oyster research and development’ for which the Northern Territory Government Department of Industry, Tourism and Trade is the commissioned organisation. The authors thank the Anindilyakwa Land Council of Groote Eylandt for the supply of broodstock oysters. We also recognise the significant support provided by the Darwin Aquaculture Centre staff; in particular, we thank Matthew Osborne, Layla Hadden, Mitchell Beagley, Shanika Jeewantha Arachchi, Cameron Hartley and Kitman Dyrting. We thank the reviewer and editor who made valuable contributions to this manuscript.
Disclosure statement
No potential conflict of interest was reported by the authors.
Additional information
Funding
References
- Acquafredda, M.P., Morris, N., Calvo, L., De Luca, M. & Munroe, D. (2022) Evaluating the efficacy of nursery gear types for cultivating Atlantic surfclams (Spisula solidissima). Aquaculture Reports 25, 101186. doi:10.1016/j.aqrep.2022.101186.
- Bryson, R.K. (1977) Tropical oyster farming a valuable new industry. Australian Fisheries 36, 2–6.
- Cáceres-Puig, J.I., Abasolo-Pacheco, F., Mazón-Suastegui, J.M., Maeda-Martínez, A.N. & Saucedo, P.E. (2007) Effect of temperature on growth and survival of Crassostrea corteziensis spat during late-nursery culturing at the hatchery. Aquaculture 272, 417–422. https://doi.org/10.1016/j.aquaculture.2007.06.030.
- Coeroli, M., De Gaillande, D. & Landret, J.P. (1984) Recent innovations in cultivation of molluscs in French Polynesia. Aquaculture 39, 45–67. https://doi.org/10.1016/0044-8486(84)90258-8.
- Collet, B., Boudry, P., Thebault, A., Heurtebise, S., Morand, B. & Gerard, A. (1999) Relationship between pre-and post-metamorphic growth in the Pacific oyster Crassostrea gigas (Thunberg). Aquaculture 175(3–4), 215–226.
- Dubert, J., Barja, J.L. & Romalde, J.L. (2017) New insights into pathogenic Vibrios affecting bivalves in hatcheries: present and future prospects. Frontiers in Microbiology 8(762), https://doi.org/10.3389/fmicb.2017.00762.
- Gjedrem, T. & Rye, M. (2018) Selection response in fish and shellfish: a review. Reviews in Aquaculture 10, 168–179. https://doi.org/10.1111/raq.12154.
- Glude, J.B. (1984) The applicability of recent innovations to mollusc culture in the western Pacific Islands. Aquaculture 39, 29–43. https://doi.org/10.1016/0044-8486(84)90257-6.
- Gosling, E. (2003) Bivalve Molluscs: Biology, Ecology and Culture. Fishing News Books, Oxford. https://doi.org/10.1002/9780470995532.
- Hollenbeck, C.M. & Johnston, I.A. (2018) Genomic tools and selective breeding in molluscs. Frontiers in Genetics 9(253), https://doi.org/10.3389/fgene.2018.00253.
- Kinch, J., Yabakiva, M., Waqainabete, P., Nalasi, U., Pickering, T., Ravunamoce, P. & Romeo, A. (2020) Exploring the market potential for Fiji’s Rewa River oysters. SPC Fisheries Newsletter 160, 48–54.
- Luna, L.G. (1968) Manual for Histological Staining Methods of the Armed Forces Institute of Pathology, third edition. McGraw-Hill Book Company, New York.
- Mazón-Suástegui, J.M., Rivero Suarez, S.E., Betanzos Vega, A., Saucedo, P.E., Rodriguez Jaramillo, C. & Acosta Salmon, H. (2017) Potential of sites in northern Cuba for developing an industry of the native mangrove oyster (Crassostrea rhizophorae). Latin American Journal of Aquatic Research 45, 218–222. https://doi.org/10.3856/vol45-issue1-fulltext-24.
- Mazón-Suástegui, J.M., Ruíz-Ruíz, K.M., Parres-Haro, A. & Saucedo, P.E. (2008) Combined effects of diet and stocking density on growth and biochemical composition of spat of the Cortez oyster Crassostrea corteziensis at the hatchery. Aquaculture 284, 98–105. https://doi.org/10.1016/j.aquaculture.2008.07.022.
- Nell, J.A. & Holliday, J.E. (1988) Effects of salinity on the growth and survival of Sydney rock oyster (Saccostrea commercialis) and Pacific oyster (Crussostrea gigas) larvae and spat. Aquaculture 68, 39–44. https://doi.org/10.1016/0044-8486(88)90289-X.
- Nowland, S. & Hartley, C. (2021) Hatchery protocol for the Blacklip Rock Oyster Saccostrea echinata (Quoy and Gaimard, 1835). In: M. Osborne, G. Schipp, W. O’Connor & P. Southgate (Eds.), Northern Territory Government, Australia, Fishery Report No. 123, pp. 1–30. Darwin. Northern Territory Government Australia.
- Nowland, S.J., O’Connor, W.A., Osborne, M.W.J. & Southgate, P.C. (2019b) Current status and potential of tropical rock oyster aquaculture. Reviews in Fisheries Science & Aquaculture 28(1), 57–70. https://doi.org/10.1080/23308249.2019.1670134.
- Nowland, S.J., O'Connor, W.A., Penny, S.S., Osborne, M.W.J. & Southgate, P.C. (2019a) Water temperature and salinity synergistically affect embryonic and larval development of the tropical black-lip rock oyster Saccostrea echinata. Aquaculture International 27(5), 1239–1250. https://doi.org/10.1007/s10499-019-00381-7.
- Nowland, S.J., O’Connor, W.A., Penny, S.S. & Southgate, P.C. (2019c) Monsoonally driven reproduction in the tropical black-lip rock oyster Saccostrea echinata (Quoy & Gimard, 1835) in northern Australia. Journal of Shellfish Research 38(1), 1–12. https://doi.org/10.2983/035.038.0100.
- Nowland, S.J., O'Connor, W.A. & Southgate, P.C. (2018a) Embryonic, larval, and early postlarval development of the tropical black-lip rock oyster Saccostrea echinata. Journal of Shellfish Research 37, 73–77. https://doi.org/10.2983/035.037.0100.
- Nowland, S.J., O'Connor, W.A. & Southgate, P.C. (2018b) Optimizing stocking density and microalgae ration improves the growth potential of tropical black-lip oyster, Saccostrea echinata, larvae. Journal of the World Aquaculture Society 50(4), 728–737. https://doi.org/10.1111/jwas.12581.
- Pfeiffer, T. & Rusch, K. (2001) Comparison of three culture methods for the intensive culture of northern quahog seed, Mercenaria mercenaria. Journal of the World Aquaculture Society 32, 11–20. doi:10.1111/j.1749-7345.2001.tb00917.x.
- Raphael, S.S., Culling, C.F.A., Inwood, M.J., Thomson, S. & Sergovich, F. (1976) Lynch’s Medical Laboratory Technology, third edition. W.B. Saunders, Philadelphia.
- R Core Team (2017) R: A language and environment for statistical computing. https://www.R-project.org/.
- Sachs, J.D. (2001) Tropical underdevelopment. NBER working paper series. National Bureau of Economic Research, Cambridge.
- Shumway, S. (2021) Molluscan Shellfish Aquaculture: A Practical Guide. 5 m Books Ltd. Great Easton, Essex, UK.
- SOTT (2017) State of the Tropics: Sustainable Infrastructure for the Tropics. James Cook University, Townsville.
- Southgate, P.C. & Lee, P.S. (1998) Hatchery rearing of the tropical blacklip oyster Saccostrea echinata (Quoy and Gaimard). Aquaculture 169, 275–281. https://doi.org/10.1016/S0044-8486(98)00382-2.
- Utting, S.D. & Spencer, B.E. (1991) The hatchery culture of bivalve mollusc larvae and juveniles. Laboratory Leaflet 68: Ministry of Agriculture, Fisheries and Food Directorate of Fisheries Research, Lowestoft.
- Videla, J.A., Chaparro, O.R., Thompson, R.J. & Concha, I.I. (1998) Role of biochemical energy reserves in the metamorphosis and early juvenile development of the oyster Ostrea chilensis. Marine Biology 132, 635–640. doi:10.1007/s002270050428.
- Wadsworth, P., Wilson, A.E. & Walton, W.C. (2019) A meta-analysis of growth rate in diploid and triploid oysters. Aquaculture 499, 9–16. https://doi.org/10.1016/j.aquaculture.2018.09.018.
- Wallace, R.K., Waters, P. & Rikard, F.S. (2008) Oyster Hatchery Techniques. Southern Regional Aqaculture Center, Stoneville, Mississippi, USA.
- Wang, T. & Li, Q. (2018) Effects of salinity and temperature on growth and survival of juvenile Iwagaki oyster Crassostrea nippona. Journal of Ocean University of China 17, 1–6. https://doi.org/10.1007/s11802-018-3536-4.
- White, M.J. (1991) Aspects of growth and reproduction of Saccostrea echinata the Indigenous blacklip oyster cultured at Magnetic Island Queensland, Unpublihed Honours thesis, James Cook University, Townsville.
- Willer, D.F. & Aldridge, D.C. (2020) Sustainable bivalve farming can deliver food security in the tropics. Nature Food 1, 384–388. https://doi.org/10.1038/s43016-020-0116-8.