ABSTRACT
Shiitake mushroom (Lentinula edodes) is one of the most commonly consumed mushrooms and an important forest product in Japan. In outdoor log cultivation of shiitake, colonization of logs by wild fungi is an important factor affecting mushroom yield. In this study, we investigated the fungal communities in logs used for shiitake cultivation in three management conditions (two artificial laying yards and a forest floor) via isolation and molecular identification of individual species and examined their association with shiitake production. The isolation frequency of shiitake mycelia explained the mushroom yield, demonstrating that mycelial occupancy of logs is a useful indicator of mushroom yield. The occurrence pattern of other fungal species explained some of the variations in shiitake production, and white-rot fungi especially were found to be associated with mushroom yield. Although management conditions during the spawn run did not influence the richness and composition of fungi in logs, the isolation frequency of wild fungi differed among the treatments.
Introduction
The shiitake mushroom, Lentinula edodes (Berk.) Pegler, is one of the most commonly consumed mushrooms and an important forest product in Japan (Takabatake Citation2015). In 2018, shiitake production was 88,832 tonnes, accounting for 19% of total mushroom production in Japan (Forestry Agency Citation2019). Lentinula edodes is a white-rot fungus belonging to the Basidiomycetes, growing in dead wood of broad-leaved trees in nature. Commercially, shiitake mushrooms are cultivated on logs placed on the forest floor, as well as indoors on synthetic sawdust substrates. Although indoor sawdust cultivation has become the main method for production of shiitake mushrooms in recent years (75% of total production), traditional log-cultivated shiitake mushrooms remain a significant part of overall shiitake production (Takabatake Citation2015; Forestry Agency Citation2019). In Japan, oak logs (Quercus serrata or Q. acutissima) are usually used for shiitake cultivation. Generally, logs that are 10–15 cm in diameter are selected and cut to 1 m in length in late fall and winter, and shiitake spawn is inoculated early the following spring. After inoculation, the logs are stacked horizontally to facilitate shiitake mycelial growth throughout the log over several months (spawn run). After mycelia have fully colonized the logs, they are raised to lean them against a tree or fence to promote fruiting body production, and mushrooms are usually harvested over several years. Because some or all steps of shiitake log cultivation are performed outdoors, mushroom production can be influenced by natural conditions, including climatic and biological factors (Tokimoto Citation2010).
Colonization of logs by wild fungi is an important factor affecting the success of outdoor shiitake production. To date, more than 50 species of fungi have been reported as pests of shiitake log cultivation (Niisato Citation1980; Furukawa and Nobuchi Citation1986). These fungi can be roughly divided into two types in terms of their relationship with the shiitake mycelia growing within the logs: resource competitors and parasites. Wood-decay fungi of a wide range of taxa compete with shiitake for resources. Their mycelia prevent shiitake mycelia from spreading through the wood substrate by exclusively occupying a certain volume of the log. This may reduce shiitake mushroom formation by limiting the resources available to the shiitake mycelium. In particular, white-rot fungi are potentially strong competitors, because they consume similar resources (including lignin, cellulose, and hemicellulose) to L. edodes, which is also a white-rot fungus. The other pests of shiitake production are parasitic fungi. They attack or parasitize the mycelium of L. edodes and cause damage. For example, the antagonistic activity of Trichoderma spp., the most common pest of shiitake cultivation, is well studied under experimental conditions (Tokimoto Citation1985). Both types of wild fungi can negatively affect shiitake production.
The logs used for shiitake cultivation are usually inhabited by a large number of wild fungal species, which affects shiitake production. However, most previous studies have focused on the one-on-one response of shiitake mycelia (or production) to specific pest species under experimental conditions (e.g., Tokimoto Citation1985; Tsunoda et al. Citation1996, Citation1999; Tsunoda and Taniguchi Citation2015). Thus, the effect of many co-occurring wild fungal species on shiitake yield under actual log cultivation conditions is still poorly investigated. To understand the comprehensive effect of fungal community on shiitake production, quantitative assessment of fungal communities and the relative importance of each fungal species for shiitake production are required. To evaluate the fungal communities in logs, systematic sampling and accurate identification are needed. Isolation and molecular identification of fungal species using DNA barcoding is an effective method for this purpose (Seifert Citation2009), but few studies on shiitake cultivation have used such an approach. In addition, management conditions are important for colonization of logs by wild fungi because these fungi differ in their environmental preferences. In particular, logs are laid in several types of laying yards during the spawn run, such as artificial laying yards (flat ground covered with sunshade) or the forest floor. Type of laying yard is an important factor in colonization by wild fungi, because the environment (source of wild fungi) and microclimate conditions would differ between yards and yard types (Idei Citation1981; Tokimoto Citation2010).
In this study, we investigate fungal communities in logs used for shiitake cultivation via isolation and molecular identification of individual fungal species. The association of fungal communities with shiitake production is also examined. We demonstrate that mycelial occupation of logs is a good indicator of shiitake production and identify fungi associated with shiitake production. We also address the effects of management conditions on the fungal communities present in logs, which affect shiitake production.
Materials and methods
Study system and yield assessment
Shiitake mushrooms were cultivated on 21 logs of Q. acutissima (length 100 cm, diameter 7.9–13.0 cm) at the Mushroom Research Group of Oita Prefectural Agriculture, Forestry and Fisheries Research Center in Oita Prefecture, Japan (32°59′15″N, 131°35′24″E). The mean annual temperature as measured at the nearest meteorological station (Inukai) is 15.0°C, with a January mean minimum of −1.1°C and an August mean maximum of 32.2°C. Mean annual precipitation is 1725.5 mm (Japan Meteorological Agency Citation2019). The logs were inoculated with fungal spawn of shiitake (variety ‘Sekko H3ʹ) at 32 sites per log on 2 March 2018. After inoculation, logs were stacked in an artificial laying yard (on flat ground, under a sunshade) until 30 July, and then seven logs were placed at each of three outdoor laying yards: (i) the original laying yard, (ii) another artificial laying yard, and (iii) the forest floor. The two artificial laying yards have a similar structure; logs were placed on the ground, which is covered with gravel, and the canopy was covered with a sunshade net (Daio-furara, Daio Kasei co., Japan). The forest laying yard was located in a plantation of Japanese cedar (Cryptomeria japonica). The three yards were more than 40 m distant from each other. On 15 October, all logs were taken to the indoor cultivation facility and leaned against horizontal rails. To induce fruiting, logs were soaked in water for 4 h every month, a total of seven times, until March 2019. Shiitake mushrooms were harvested when their caps were 70–90% open. Harvested mushrooms were dried at 40°C to 58°C and their dry weights were measured.
Fungal isolation
In April 2019, logs were moved to the Forestry and Forest Products Research Institute in Ibaraki Prefecture, Japan (36°00′31″N, 140°07′44″E). Each log was covered by Tetoron mesh (mesh size <0.5 mm; Toray Industries, Inc., Tokyo, Japan) to keep insects out and leaned against a chain-link fence in the shade. Cross-sections (3 cm thick) were cut from each log at three equally spaced locations on each log from May to June. Wood chip samples (approximately 1 mm3) were taken from at least five fixed points (the center and four midpoints between the center and the bark) on each disc using a sterilized dissecting knife; three chips were collected from each point (n = 45 chips per log) as replicates. Additional samples were collected from any areas distinguished by pigmentation or zone lines presumably resulting from different fungal colonies. For all discs, the three chips per sampling point were individually placed on potato dextrose agar (PDA), a basidiomycete-selective medium, amended with streptomycin (300 ppm) and benomyl (5 ppm) in Petri dishes to isolate shiitake and other wood-decaying basidiomycetes. In addition, wood chips from one disc of each log (five fixed and some additional points per log) were incubated on PDA medium without benomyl to isolate all fungi including fast-growing ascomycetes. In total, we cut wood chips from 456 points (315 fixed points and 141 additional points) on 63 discs and made 1839 isolates (1368 on basidiomycete-selective media and 474 on non-benomyl media). Macroscopic features of colonies, such as growth pattern and color, were examined for preliminary identification of fungal species. When isolates from the same replicate presented identical features, we selected only one of the isolates for pure culture. Finally, 432 samples were selected for pure culture and subsequent DNA barcoding.
Molecular identification
Fungal isolates for DNA barcoding were transferred onto sterilized cellophane overlaid on PDA in new Petri dishes and cultured for 1–2 weeks. Mycelia grown on cellophanes were collected and genomic DNA was extracted using a PrepMAN Ultra Sample Preparation Reagent kit (Applied Biosystems, Foster City, CA, USA) following the manufacturer’s instructions. Internal transcribed spacer (ITS) and large subunit ribosomal RNA gene (LSU) of nuclear DNA sequences were amplified by polymerase chain reaction (PCR). The primers used were as follows: for ITS, ITS1 F (5′-CTT GGT CAT TTA GAG GAA GTA A-3′) (Gardes and Bruns Citation1993) and ITS4 (5′-TCC TCC GCT TAT TGA TAT GC-3′) (White et al. Citation1990); for LSU, LR0 R (5′-ACC CGC TGA ACT TAA GC-3′) and LR5 (5′-TCC TGA GGG AAA CTT CG-3′) (R. Vilgalys website: https://sites.duke.edu/vilgalyslab/rdna_primers_for_fungi/). PCR amplification was performed in 25-μL reactions using GoTaq Master Mix (Promega, Madison WI, USA) with the following conditions: initial denaturation at 94°C for 4 min, 35 cycles at 94°C for 30 sec, 55°C for 30 sec, and 72°C for 90 sec, and a final extension at 72°C for 7 min. PCR products were purified using ExoSAP-IT (Applied Biosystems) and sequencing reactions were performed by using Big Dye Terminator v3.1 (Applied Biosystems) with the above primers. Sequencing was performed on a DNA sequencer (ABI 3130xl, Applied Biosystems). Chromatograms of the obtained sequence were visually checked using MEGA7 (Kumar et al. Citation2016) and aligned using ClustalW (Larkin et al. Citation2007). We manually trimmed primer sequence and low-quality terminal fragments. Heterozygous sites were coded as IUPAC degenerate nucleotide symbols. We first sequenced ITS for 432 samples (all samples for DNA analysis) and grouped the sequences into 46 OTUs based on 99.5% identity. Then, LSU of selected samples (one per OTU) were additionally sequenced. Representative sequences of ITS and LSU for each OTU were deposited in the DNA Data Bank of Japan (DDBJ; accession numbers: LC520139–LC520229). Taxonomic assignment of OTUs was performed based on ITS and LSU sequences using the Claident v0.2.2019.05.10 software (Tanabe and Toju Citation2013). Claident retrieves neighborhood sequences based on the QCauto method (Tanabe and Toju Citation2013) using BLAST search algorithms and assigns the lowest taxonomic level common to the obtained sequences based on the lowest common ancestor algorithm (Huson et al. Citation2007). The “fungi_ITS_genus” and “eukaryote_LSU_genus” databases (downloaded from the Claident website on 27 September 2019) were used as reference databases for the BLAST search. We set the maximum acceptable proportion of opposer sequences (i.e., those with different taxonomic information to the assigned taxonomy) in neighborhood sequences at 10% and the minimum ratio of supporter/opposer sequences at 9 in the lowest common ancestor step. Fungi were identified to the lowest taxonomic level possible based on ITS and LSU sequences.
Statistical analyses
All statistical analyses were performed using R version 3.6.1 (R Core Team Citation2019). The isolation frequency was measured as the number of detections from 15 fixed points (5 points per disc) for each log. Data from additional points (i.e., sampling points except for fixed points) were not included in subsequent statistical analyses. The relationship between mushroom yield and the isolation frequency of shiitake mycelium (detected on benomyl-amended media) was analyzed by using a generalized linear model (GLM). The effect of species richness of non-shiitake fungi on shiitake yield was also analyzed by GLM. For both analyses, log link function and gamma error structure were used. Log volumes were incorporated as an offset term.
To identify fungal species strongly associated with shiitake mushroom yield, we performed a random forest analysis (Breiman Citation2001) using the “randomForest” package (Liaw and Wiener Citation2002) in R. Random forest is a machine learning algorithm that combines thousands of decision trees to identify the relationships between response and explanatory variables, automatically including non-linear relationships and interactions among them. We set mushroom yield as a response variable and occurrence of each fungal species as predictor variables. Analyses were conducted separately for datasets of fungal occurrence on benomyl-amended media and non-benomyl media. Fungal species with a very small number of occurrences (≤1) were removed from the data prior to the analysis. In total, six and five fungal species were included in analyses for benomyl-amended and non-benomyl media, respectively. The importance of each variable was determined using the importance function in the “randomForest” package, which calculates the percent increase in the mean squared error after permutation of each variable using out-of-bag samples. The proportion of variation explained by the model was calculated as “pseudo R-squared” (1 – mean squared errors divided by total variance) by the “randomForest” package.
The effect of management conditions on the species richness of and the isolation frequency of non-shiitake fungi were also analyzed by GLM with a log-link function. Poisson and gamma error structure were applied to species richness and the isolation frequency, respectively. We examined these models using a likelihood ratio test (LRT). The similarity of fungal communities among logs was calculated using Bray–Curtis distance with the isolation frequency as the abundance of each species. The effect of management conditions on fungal community structures was tested by using analysis of similarity (ANOSIM) implemented in the “vegan” package in R.
Results
Relationship between mushroom yield and dominance of shiitake and other fungal species in logs
Mushroom yield ranged from 0 to 69.67 g per log (dry weight). Shiitake mycelium was detected at 6.7% to 80.0% of fixed sampling points, and the isolation frequency of shiitake (%) was significantly associated with mushroom yield (LRT: χ2 = −11.36, P = 0.006; ). The isolation frequency of shiitake was negatively correlated with the isolation frequency of all other fungal species detected on benomyl-amended media (r = −0.62, P < 0.01). The number of non-shiitake species was significantly associated with shiitake mushroom yield for both benomyl-amended (LRT: χ2 = −8.827, P = 0.004) for and non-benomyl media (LRT: χ2 = −5.26, P = 0.043). Shiitake and other fungi were not detected from a fixed sampling point together in most cases: shiitake and other fungi were simultaneously detected on benomyl-amended media at 3.8% of fixed sampling points, while the wild fungi were detected at 24.4% of fixed points. In total, 46 different OTUs were identified from 456 points (37 OTUs from the 315 fixed points) on 21 logs (). On benomyl-amended and non-benomyl media, 28 and 29 OTUs were detected, respectively. Most OTUs were detected on only one or two logs. Claident identified 31 OTUs to species or genus level, and the remaining 15 OTUs could not be identified to genus level. Of the fungi that grew on benomyl-amended medium, 17 were basidiomycetes, 10 were ascomycetes, and one was Mucorales. Of the fungi that grew on the non-benomyl medium, 10 were basidiomycetes and 19 were ascomycetes. Xylodon subflaviporus, Trametes versicolor, and Trichoderma sp. were the most frequently isolated fungal taxa (from two types of media taken together) except for shiitake.
Table 1. List of isolated fungal taxa as annotated by Claident. Number of logs from which each taxon was isolated using benomyl-amended (BS) or non-benomyl (S) media (data from fixed and additional sampling points on each log were pooled). Accession numbers, sequence lengths, and the top hit taxa in BLAST search for the ITS and LSU sequences deposited in the DDBJ are also shown
Figure 1. Relationship between the isolation frequency of shiitake mycelia and mushroom yield under three management conditions: artificial laying yard 1 (grey circles), artificial laying yard 2 (grey squares), and forest floor (black triangles)
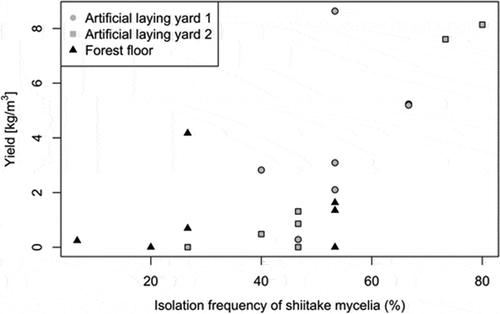
Random forest analysis showed that 11.3% of the variance in yield was explained by the occurrence of non-shiitake fungal species that grew in the benomyl-amended medium. The most important fungal species for predicting shiitake yield included X. subflaviporus and T. versicolor ()), both of which grew on benomyl-amended medium. Occurrences of these two species were negatively correlated with shiitake yield ()). In contrast, fungal species detected from the non-benomyl medium failed to explain the variation in shiitake yield (variation explained = −0.104).
Figure 2. Random forest estimates of relative importance (% of increase of mean square error) of each fungal species detected using benomyl-amended medium for predicting shiitake yield (a). Relationship between the isolation frequency of Xylodon subflaviporus (b) and Trametes versicolor (c) mycelia and mushroom yield. MSE: mean squared error
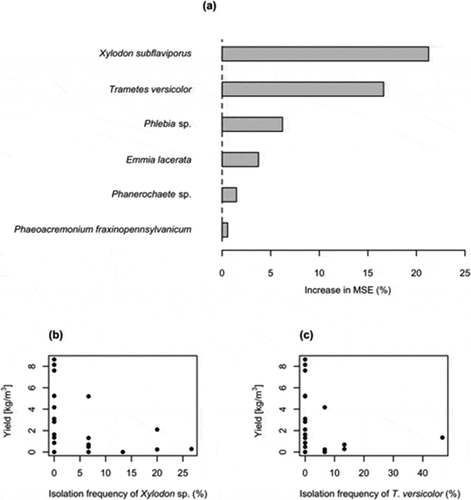
Effects of management conditions on fungal communities
The number of species (species richness) of fungal communities per log did not differ among the three management conditions, either for benomyl-amended (LRT: χ2 = −1.56, P = 0.457; )) or non-benomyl media (LRT: χ2 = −0.59, P = 0.744; )). The isolation frequency of non-shiitake fungal species detected by benomyl-amended medium significantly differed among treatments (LRT: χ2 = −5.47, P = 0.033; )), but not for non-benomyl medium (LRT: χ2 = −0.92, P = 0.236; )). Specifically, the isolation frequency of logs laid on the forest floor (38.1% on average) was higher than that of those laid at artificial laying yards (24.8% and 10.5% on average) for benomyl-amended medium ()). Fungal community composition did not differ among treatments for either benomyl-amended medium (ANOSIM: r = 0.003, P = 0.239) or non-benomyl medium (ANOSIM: r = −0.08, P = 0.942).
Discussion
In this study, we quantified the distribution of shiitake and non-shiitake mycelia in logs used for shiitake cultivation and analyzed its association with mushroom yield and management conditions during the latter period of the spawn run. The isolation frequency of shiitake mycelia explained mushroom yield and was negatively correlated with the isolation frequency of non-shiitake fungi. The occurrence pattern of fungi detected on benomyl-amended medium explained some of the variations in shiitake production, and white-rot fungi such as X. subflaviporus and T. versicolor were negatively associated with mushroom yield. In addition, the species number of non-shiitake fungi was associated with shiitake mushroom yield. Although management conditions during the spawn run did not influence the species richness or composition of fungi in logs, the isolation frequency of non-shiitake fungi detected via the benomyl-amended medium was significantly different among the treatments.
Our results demonstrate that mycelial occupancy in logs is a useful indicator of mushroom yield. For wood-decay fungi, the volume of wood substrate occupied by mycelia should reflect the resources and nutrients available for fungal growth, thus affecting the production of fruiting bodies. The mycelia of some wood-decay fungi are known to prevent colonization by mycelia of other fungi (Boddy Citation2000; Heilmann-Clausen and Boddy Citation2005). In our experiment, various fungal species, including wood-rot fungi (basidiomycetes, in particular), were detected in parts of logs not colonized by shiitake mycelia. This exclusive occurrence of shiitake and other basidiomycete fungi implies that during the spawn run, competing fungi colonize and establish territory in logs, and the growth of wild fungi can prevent the growth of shiitake mycelia. Exclusive growth of mycelia and its effect on shiitake production in logs have been confirmed in the fungal species Graphostroma platystoma and Hypoxylon truncatum under experimental conditions (e.g., Tsunoda et al. Citation1996, Citation1999; Tsunoda and Taniguchi Citation2015). In this study, we showed a negative association between multiple fungal species and shiitake production and identified specific fungal species associated with decreased mushroom yield in outdoor log cultivation, using a method similar to that used in commercial shiitake cultivation.
This study revealed that various non-shiitake species are present in logs used in shiitake cultivation, including some that have never been reported in shiitake cultivation. Likely, our combination of isolation and molecular identification allowed us to identify these species. However, most of the frequently isolated fungi (e.g., X. subflaviporus, T. versicolor, Trichoderma sp.) or their relatives have already been recorded as antagonistic fungi in shiitake cultivation (Furukawa and Nobuchi Citation1986). White-rot fungi such as X. subflaviporus and T. versicolor showed a relatively strong negative association with shiitake production, implying that resource competition is an important factor for shiitake mushroom production, at least under our experimental conditions. It should be noted that the ITS sequence identified as X. subflaviporus in this study differed (<97.7%) from that of Xylodon flaviporus previously recorded in Japan and was identical (>99.6%) to X. subflaviporus recently described from China (Chen et al. Citation2018). It is possible that previous records of X. flaviporus (“anatake” in Japanese) from shiitake cultivation actually included the two different species. However, additional investigation, including examination of fruiting bodies, would be needed for accurate identification.
Trichoderma sp. was the OTU most frequently detected in non-benomyl media, but no association with shiitake yield was found. Trichoderma is known to have a strong negative effect on shiitake production in later stages (>3 years after inoculation) of cultivation (Tokimoto Citation1985). Thus, it is possible that Trichoderma sp. did not have a conspicuous effect on shiitake yield at the early stage of cultivation in our study. Another explanation is that the Trichoderma occurrence examined after the harvest in our study did not reflect that during shiitake mycelial growth, because of the rapid mycelial growth (Kim et al. Citation2012) and high temporal turnover of Trichoderma. Most of the other non-shiitake fungi were detected less frequently, and their impact on shiitake production remains unknown, but they are potential competitors with shiitake.
Management conditions had some influence on the fungal community in logs. Two factors influence the fungal community structure in logs: the species pool and growth conditions. Many wood-inhabiting fungi colonize logs via wind dispersal of spores. Therefore, the species composition of spores in the atmosphere would affect the community structure of wood-inhabiting fungi. Indeed, spores in the atmosphere are known to vary depending on the surrounding landscape at a local scale (Edman et al. Citation2004; Peay and Bruns Citation2014). In addition, thermal and water conditions at laying yards may affect fungal establishment and mycelial growth (Furukawa and Nobuchi Citation1986). The concordant species composition of fungi among the three treatments in our study implies that the species pool of colonizing fungi does not differ among them; the higher frequency of detection of non-shiitake mycelia in the forest laying yard may reflect growth conditions more suitable for competing fungi than for shiitake. Because the three laying yards used in this study are near each other, fungal species number and composition might not differ among the logs placed in the three laying yards. In contrast, differences in the isolation frequency of wild fungal mycelia may reflect the influence of local conditions on the growth rate of competing fungi. However, the short treatment period in our experiment should be noted: the logs were placed at different conditions only during the latter period of the spawn run. In commercial outdoor cultivation of shiitake, logs can be exposed to various conditions during the whole period of cultivation from log felling to final harvesting. In addition, further studies with multiple sites and/or time points are needed to generalize the effect of management conditions on shiitake production.
Acknowledgements
We thank H. Kurokawa for her support and valuable suggestions.
Disclosure statement
We have no potential conflict of interest.
Additional information
Funding
References
- Boddy L. 2000. Interspecific combative interactions between wood-decaying basidiomycetes. FEMS Microbiol Ecol. 31:185–194. doi:10.1111/j.1574-6941.2000.tb00683.x.
- Breiman L. 2001. Random forests. Mach Learn. 45:5–32. doi:10.1023/A:1010933404324.
- Chen CC, Wu SH, Chen CY. 2018. Xylodon subflaviporus sp. nov. (Hymenochaetales, Basidiomycota) from East Asia. Mycoscience. 59:343–352. doi:10.1016/j.myc.2017.12.004.
- Edman M, Gustafsson M, Stenlid J, Jonsson BG, Ericson L. 2004. Spore composition of wood-decaying fungi: importance of landscape composition. Ecography. 27:103–111. doi:10.1111/j.0906-7590.2004.03671.x.
- Forestry Agency. 2019. [Forest products statistics]. Tokyo: Forestry Agency, Ministry of Agriculture, Forestry and Fisheries (Japan); [accessed 2020 Jan 8]. Japanese https://www.maff.go.jp/j/tokei/kouhyou/tokuyo_rinsan/
- Furukawa H, Nobuchi T. 1986. [Handbook of disease and pests of mushroom cultivation]. Japanese. Tokyo: Zenrinkyo.
- Gardes M, Bruns TD. 1993. ITS primers with enhanced specificity for basidiomycetes – application to the identification of mycorrhizae and rusts. Mol Ecol. 2:113–118. doi:10.1111/j.1365-294X.1993.tb00005.x.
- Heilmann-Clausen J, Boddy L. 2005. Inhibition and stimulation effects in communities of wood decay fungi: exudates from colonized wood influence growth by other species. Microb Ecol. 49:399–406. doi:10.1007/s00248-004-0240-2.
- Huson DH, Auch AF, Qi J, Schuster SC. 2007. MEGAN analysis of metagenomic data. Genome Res. 17:377–386. doi:10.1101/gr.5969107.
- Idei T. 1981. [Influences of environment for Hodagi, on the cultivation of Lentinus edodes]. Bull Utsunomiya Univ For. 17:75–87. Japanese.
- Japan Meteorological Agency. 2019. Climate Statistics. Tokyo: Japan Meteorological Agency. [accessed 2020 Jan 8]. https://www.jma.go.jp/jma/indexe.html.
- Kim CS, Park MS, Kim SC, Maekawa N, Yu SH. 2012. Identification of Trichoderma, a competitor of shiitake mushroom (Lentinula edodes), and competition between Lentinula edodes and Trichoderma species in Korea. Plant Pathol J. 28:137–148. doi:10.5423/PPJ.2012.28.2.137.
- Kumar S, Stecher G, Tamura K. 2016. MEGA7: molecular evolutionary genetics analysis version 7.0 for bigger datasets. Mol Biol Evol. 33:1870–1874. doi:10.1093/molbev/msw054.
- Larkin MA, Blackshields G, Brown NP, Chenna R, McGettigan PA, McWilliam H, Valentin F, Wallace IM, Wilm A, Lopez R, et al. 2007. ClustalW and ClustalX version 2.0. Bioinformatics. 23:2947–2948. doi:10.1093/bioinformatics/btm404.
- Liaw A, Wiener M. 2002. Classification and regression by randomForest. R News. 2(3):18–22.
- Niisato T. 1980. [Occurrence trend and control practice of fungal disease in shiitake cultivation]. Rep Iwate Pref For Exp Stn. 13:43–51. Japanase.
- Peay KG, Bruns TD. 2014. Spore dispersal of basidiomycete fungi at the landscape scale is driven by stochastic and deterministic processes and generates variability in plant–fungal interactions. New Phytol. 204:180–191. doi:10.1111/nph.12906.
- R Core Team. 2019. R: A language and environment for statistical computing. Vienna: R Foundation for Statistical Computing. https://www.R-project.org/.
- Seifert KA. 2009. Progress towards DNA barcoding of fungi. Mol Ecol Res. 9:83–89. doi:10.1111/j.1755-0998.2009.02635.x.
- Takabatake K. 2015. [Current trends and future prospects for mushroom production in Japan]. J Japan Wood Res Soc. 61:243–249. Japanese. doi:10.2488/jwrs.61.243.
- Tanabe AS, Toju H. 2013. Two new computational methods for universal DNA barcoding: a benchmark using barcode sequences of bacteria, archaea, animals, fungi, and land plants. PLoS One. 8:e76910. doi:10.1371/journal.pone.0076910.
- Tokimoto K. 1985. [Physiological studies on antagonism between Lentinula edodes and Trichoderma spp. in bedlogs of the former]. Rep Tottori Mycol Inst. 23:1–54. Japanese.
- Tokimoto K. 2010. [The foundation of shiitake cultivation on logs]. Mushroom Sci Biotechnol. 18:131–138. Japanese.
- Tsunoda M, Hidaka T, Ando M. 1999. [Location and timing of invasion of the Graphostroma platystoma ascospores into bed-logs for the Shiitake (Lentinula edodes) mushroom cultivation]. Mushroom Sci Biotechnol. 7:181–188. Japanese.
- Tsunoda M, Hidaka T, Taniguchi M. 1996. [Development of Graphostroma platystoma on bed-logs of shiitake (Lentinus edodes) and assessment of its injurious effects]. Trans Mycol Soc Jpn. 37:81–89. Japanese.
- Tsunoda M, Taniguchi M. 2015. [Quadrat method for measurement of injurious effects of Hypoxylon truncatum on bed logs used for shiitake (Lentinula edodes) cultivation]. Mushroom Sci Biotechnol. 23:13–15. Japanese.
- White TJ, Bruns T, Lee S, Taylor JW. 1990. Amplification and direct sequencing of fungal ribosomal RNA genes for phylogenetics. In: Innis MA, Gelfand DH, Sninsky JJ, White TJ, editors. PCR protocols: a guide to methods and applications. New York (NY): Academic Press, Inc.; p. 315–322.