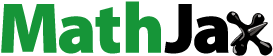
ABSTRACT
Xanthoceras sorbifolium is one of the most economically important species cultivated in northern China. To analyze the phenotypic variation within X. sorbifolium, 35 phenotypic traits among 37 elite germplasms were analyzed using nested analysis of variance, coefficient of variation (CVs), principal component analysis (PCA), correlation analysis, and cluster analysis. Large variation and rich phenotypic diversity were found among and within germplasms of X. sorbifolium. The mean of coefficient of variation for 35 traits among 37 germplasms was 25.49%. It varied from 12.80% to 63.25%, and ranked as follows: branch traits (45.51%) > inflorescence traits (31.01%) > fruit traits (24.67%) > flower traits (24.35%) > seed traits (21.82%) > leaf traits (21.45%). The mean heritability (H2) of 35 phenotypic traits was 75.94%. Significant correlations were observed within and among most branch, leaf, inflorescence, flower, fruit, and seed traits. The cumulative contribution rate of the first nine main principle components reached 80.333%. The 35 phenotypic traits were classified into two main categories and five subcategories by clustering analysis of phenotypic traits. Combined result of correlation, PCA, and clustering of phenotypic traits, 18 representative traits were selected. The 37 elite germplasms were classified into two main groups and six subgroups according to their characteristics by Ward’s method and reasonable utilization of each subgroup were suggested. These results provide useful information for genetic improvement, preservation, and evaluation of X. sorbifolium germplasms.
Introduction
Xanthoceras sorbifolium – a deciduous shrub or small tree which grows up to 2–10 m high – is the only species in the genus Xanthoceras (family Sapindaceae) that is native to China and is widely distributed in arid and semiarid regions of northern China (Yu et al. Citation2017). Its branches are brownish red, strong, glabrous, or slightly hairy, and its buds have imbricate scales. The leaves are pinnately compound with 9–19 lanceolate or subovate leaflets, acuminate apex, sharply serrate margin, and are membranous or papery. Racemes are up to 30 cm long, and inflorescences are terminal (male ones are axillary). Petals are white (less pink, red, or purple) with a purplish red or yellow base. Fruits capsules are either spherical or cylindrical with yellow or yellowish-brown color, containing 8–20 spherical, black seeds. Its flowering season is from late April to early May, and the fruiting period is from mid-May to early August. The plant usually fruits in the third year after sowing and has a lifespan of more than 200 years (Wang et al. Citation2019). It is widely adaptable to various growth conditions, such as drought, low temperature, salt, alkali, and other conditions (Fu et al. Citation2008; Zhou and Cai Citation2018). It can be used for woody edible oil and biofuel production, because the oil content in its seed kernel could be as high as 67%, of which 85–93% are unsaturated fatty acids, including 37.1–46.2% linoleic acid and 28.6–37.1% oleic acid, which are essential dietary fatty acids (Venegas-Calerón et al. Citation2017). Notably, nervonic acid, an essential nutrient for brain growth and maintenance but rarely contained in plants, can account for as much as nearly 3.04% of the seed kernel oil of X. sorbifolium (Bi et al. Citation2019). It also contains diverse bioactive compounds that are known functional ingredients of traditional Chinese medicines (Rong et al. Citation2016). Special support has been provided by the Chinese government to aid the development of X. sorbifolium cultivation. At least 5 × 105 ha of X. sorbifolium has been cultivated up to 2020 (Yu et al. Citation2017). Over the last five years, the number of X. sorbifolium registered in China has substantially increased. Until 2020, more than 40 varieties had been registered. There are still many strains and plus trees being selected.
Plant phenotypic diversity is a comprehensive manifestation of genetic diversity, environmental diversity, and a series of complex intermediate links between the phenotype and genotype, such as gene expression, individual development, and regulation (Li et al. Citation2002; Mohamed et al. Citation2009).The study of phenotypic diversity provides the most traditional and convenient way to analyze genetic diversity through phenotypic traits (Schaal et al. Citation1991). Currently, many scholars are taking advantage of relatively stable phenotypic traits to uncover genetic variation and evaluate the level of genetic diversity in forest species (Li et al. Citation2018, Citation2020; Cheng et al. Citation2020).
Despite high economic value and rich elite germplasms, little research has been done to analyze the phenotypic diversity in elite germplasms of X. sorbifolium. In this study, phenotypic traits of branch, leaf, flower, fruit, and seed of registered or selected X. sorbifolium germplasms were comprehensively and systematically measured to uncover the degree of phenotypic variation and differentiation within and among germplasms, and provide a theoretical basis for genetic improvement, preservation, and evaluation of the X. sorbifolium germplasms.
Materials and methods
Experimental site
The experiment was conducted at a X. sorbifolium germplasm conservation plot in the Yinchuan Botanical Garden in Yinchuan, Ningxia, China (106°27′E, 38°47′N). The site has continental climate with an annual mean temperature of 10.1°C, an average maximum and minimum temperature of 37.2°C in July and −27.9°C in January, respectively. The average annual precipitation and evapotranspiration are 181.2 mm and 1,882.5 mm, respectively. The soil texture is fine sand, and the pH is 9.07.
Plant materials and sample collection
The scions of the 37 elite germplasms (Table S1) of X. sorbifolium were collected from the main distribution area of X. sorbifolium. They were grafted, and plantations were established using three-year-old rootstocks in the spring of 2015. The seeds used to grow the rootstocks came from a 12-year-old tree, planted at the Yinchuan Botanical Garden.
The 37 elite germplasms comprise a diverse representation of elite strains and commercial varieties that exhibit one or more desirable characteristics, such as lush branches and leaves, big fruits, big seeds, big and colorful flowers, high content of kernel oil, high disease resistance, etc. Breeders consider these characteristics as highly relevant for the improvement of X. sorbifolium.
All germplasms were grafted in a randomized complete block design with three replications, each replication consists of 10 plants in a row. The planting densities was 1650 plants per hectare. The germplasms were drip-irrigated and drip-fertilized. The control of disease, pests, and weeds were performed uniformly. Pruning was done in every spring by the same experienced worker in a similar manner for all germplasms.
Sample collection
An individual plant with a typical growth, with no pests or diseases, was randomly selected from the plot, i.e. three plants per germplasm. For each individual plant, 10 inflorescences and 30 flowers from the middle and upper canopy were randomly selected in the middle of the blooming period in late April. Five annual branches, 15 compound leaves, and 15 leaflets from the middle and upper canopy were randomly selected in late July. All the fruits were collected at maturity in early August, and 10 fruits and 15 seeds were randomly selected based on the sample quartiles after drying. All samples were collected and numbered according to the individual plants. Measurements for branches, compound leaves, leaflets, inflorescences, and flowers, were performed on fresh samples, while the measurements of fruits and seeds were performed after natural drying.
Measurement of phenotypic traits
A total of 35 traits were measured or calculated using an electronic balance and an electronic digital caliper for the 37 elite germplasms. The 35 traits were as follows: three branch traits including annual branch length (ABL/mm), annual branch diameter (ABD/mm) and compound leaf number (CLN/No.); six leaf traits including, rachis length (RL/mm), rachis width (RW/mm), leaflet number (LN/No.), leaflet length (LL/mm), leaflet width (LW/mm), and leaflet shape index (LSI/%); five inflorescence traits including inflorescence length (IL/mm), inflorescence width (IW/mm), inflorescence axis diameter (ID/mm), inflorescence shape index (ISI/%), and flower number (FN/No.); seven flower traits including flower length (FL/mm), corolla diameter (CD/mm), peduncle length (PL/mm), peduncle diameter (PD/mm), petal length (PL1/mm), petal width (PW/mm), and petal shape index (PSI/%); seven fruit traits including fruit weight (FW/g), single-fruit seed number (SFSN/No.), single-fruit seed weight (SFSW/g), fruit transverse diameter (FTD/mm), fruit longitudinal diameter (FLD/mm), fruit shape index (FSI/%), and seed percentage (SP/%); and seven seed traits including seed transverse diameter (STD/mm), seed longitudinal diameter (SLD/mm), seed shape index (SSI/%), seed weight (SW/g), kernel weight (KW/g), kernel percentage (KP/%), and seed 100-grain weight (SHGW/g). Inflorescence, petal, leaflet, fruit, and seed indexes were calculated by dividing the longitudinal diameter by the transverse diameter in each case. Seed and kernel percentage were calculated by dividing the seed by the fruit weight and the kernel by the seed weight, respectively. The study was conducted through 2019–2020, over two consecutive years.
Statistical analyses
Nested variance analysis was performed using SPSS 20.0. The linear model used was , where Yijk is the performance of observation k of individual tree j of germplasm i, μ is the general average value, τi is the effective value of the ith germplasm, δj(i) is the effective value of jth individual of the ith germplasm, and εk(ij) is the error in the ijkth observed value (Li et al. Citation2018). The broad sense heritability (H2) (sometimes termed repeatability (R)) (Holland et al. Citation2003; Piepho and Möhring Citation2007), was calculated using the formula
, where σ2t/s is the variance component among germplasms, σ2s is the variance component within germplasms, σ2e is error variance, and k is the number of measurements in a tree (White and HodgeCitation1989). The coefficient of variation (CV) was calculated as
, where S is the standard deviation, and
is the average (Das and Divakara Citation2011). Correlations between pairs of phenotypic traits were analyzed using Pearson correlation analysis (Yang et al. Citation2009; Li et al. Citation2015). Principal component analysis (PCA) was applied to evaluate the underlying dimensionality of the variables, and the mean of individual tree of each phenotypic trait standardized from the z-score were used for the PCA analysis. Hierarchical cluster analysis of germplasm and phenotypic traits were performed by Ward’s method using the squared Euclidean distance to calculate the cluster distance. And the mean of germplasm values of each phenotypic trait standardized from the z-score were used for cluster analysis. Statistical analyses were performed using SPSS 20.0.
Results
The diversity of 35 phenotypic traits among 37 germplasms
The mean, minimum and maximum values, standard deviations (SD), and coefficient of variation (CVs) of 35 phenotypic traits among 37 germplasms were shown in . Significant variation was observed. The mean CV of all traits was 25.49%, varying from 12.80% to 63.25%. Traits with the largest CVs were ABL (63.25%), PD (53.06%), and ID (42.57%), with the ranges 1.90–66.50 mm, 0.10–1.20 mm, and 0.99–10.20 mm, respectively. The traits with the smallest CVs were SSI (12.80%), SLD (13.54%), and SP (13.83%), with the ranges 0.62–1.60, 7.99–19.59 mm, and 11.98%-69.85%, respectively. The mean CV for branch, leaf, inflorescence, flower, fruit, and seed traits ranked as follows: branch traits (45.51%) > inflorescence traits (31.01%) > fruit traits (24.67%) > flower traits (24.35%) > seed traits (21.82%) > leaf traits (21.45%).
Table 1. Analysis of diversity of 35 phenotypic traits among 37 X.sorbifolium germplasms
Phenotypic variation of 35 phenotypic traits among 37 germplasms
Nested analysis of variance revealed wide variation in phenotypic traits of X. sorbifolium among and within germplasms (). The F value among germplasms ranged from 4.750 to 385.547 (p < 0.01), and within germplasms, it ranged from 1.307 to 19.667 (p < 0.01). Extremely significant differences were exhibited in phenotypic traits, except for LN, PD, and SSI.
Table 2. Nested Variation analysis of 35 phenotypic traits among 37 X.sorbifolium germplasms
Broad sense heritability of phenotypic traits
The percentage of variance components and broad sense heritability were listed in . The average percentage of variance components among germplasms was 49.563%, greater than that within germplasms (10.117%). The broad sense heritability (H2) ranged from 18.43% to 95.65%, with an average of 75.94%, The mean H2 values for branch, leaf, inflorescence, flower, fruit, and seed traits were 57.68%, 78.60%, 68.96%, 82.53%, 75.17%, and 77.67%, respectively.
Table 3. Variance component and broad sense heritability of 35 phenotypic traits
Correlation between phenotypic traits
Highly significant correlations were observed among the 35 phenotypic traits (Table S2). Branch traits were significantly positively correlated with most inflorescence and flower traits, and a few leaf, fruit, and seed traits. Few leaf traits were significantly positively correlated with a few branch, leaf, inflorescence, fruit, and seed traits. Whereas, CLN was significantly negatively correlated with FN and SP, and RL was significantly negatively correlated with KW, KP, and SHGW. LN was significantly negatively correlated with most fruit and seed traits.
Most inflorescence traits were significantly positively correlated with each other and with most flower and branch traits, but not correlated with fruit and seed traits except for ID, which was significantly positively correlated with FTD, FLD, FSI, SLD, SSI, and KW. Most flower traits were significantly correlated with each other and with most inflorescence and branch traits and a few leaf traits. Most flower traits were not correlated with fruit and seed traits, but FN had a significant negative correlation with FW, SFSN, SFSW, FLD, SW, KW, and SHGW.
There were extremely significant positive correlations within and among fruit and seed traits. FLD, FSI, and KW were significantly correlated with 23, 22, and 18 traits, respectively.
PCA of phenotypic traits
Principal component analysis (PCA) of 35 phenotypic traits in 37 germplasms () revealed nine main principal components with eigenvalues larger than 1, and the cumulative contribution rate reached 80.333%, which suggests that most of the information in the original factors could be retained. The contribution rate from PC1 to PC9 was 25.075%, 16.345%, 9.997%, 7.221%, 6.122%, 4.800%, 4.143%, 3.633%, and 2.998%, respectively. According to the absolute values of eigenvectors, PC1 reflects mainly the fruit and seed traits, PC2 reflects mainly the flower traits, and PC3 reflects mainly the leaf traits.
Table 4. The PCA of 35 phenotypic traits among 37 X. sorbifolium germplasms
The clustering analysis of 35 phenotypic traits
The 35 phenotypic traits were classified into two main categories and five subcategories. ()).This result was in a good agreement with the results of both correlation and PCA analysis. The traits were significantly correlated with each other within the five subcategories. The subcategory I contained nine traits which were mainly related to the fruit and seed traits, consistent with PC1. Subcategory II contained five traits which were mainly related to the leaf traits, consistent with PC3. Subcategory III consisted of seven traits (including SFSN, SP, KP, PSI, LSI, FN, and RL). Subcategory IV contained six traits which were mainly related to the flower traits, consistent with PC2. Lastly, subcategory V contained eight traits which were mainly related to the branch, inflorescence, and leaf traits.
Figure 1. (a)Dendrogram of clustering analysis of 35 phenotypic traits of X. sorbifolium by Ward method. (b) Dendrogram of clustering analysis among 37 germplasms of X. sorbifolium based on 35 phenotypic traits by Ward method. (c) Dendrogram of clustering analysis among 37 germplasms of X. sorbifolium based on 18 representative traits by Ward method.
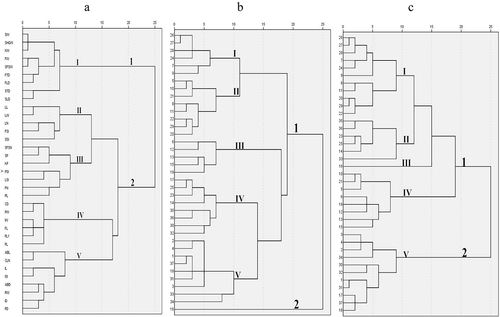
Combining the results of correlation analysis, PCA analysis, and clustering of phenotypic traits, 18 representative traits were selected, including two branch traits, ABL and ABD; three leaf traits, RL, LN, and LL; three inflorescence traits, IL, IW, and FN; four flower traits, FL, PL, PL1, and PW; three fruit traits, FW, SFSN, and SFSW; and three seed traits, SW, KW and SSI. The selected criteria of the 18 traits were as follows: according the eigenvalues from PC1 to PC9, at least two traits of each branch, leaf, inflorescence, flower, fruit, and seed, which can reflect their main characteristics were selected; then according to the clustering analysis of phenotypic traits, similar traits in the same subgroup that have more correlation with other traits were selected.
The clustering analysis of 37 elite germplasms
Cluster analysis with 35 traits () and 18 representative traits ()) were performed simultaneously. The 37 elite germplasms were classified into two main groups ()). The mean value of phenotypic traits of different groups were shown in Table S3. The first group included thirty-five germplasms divided into subgroups I, II, III, IV, and V. Subgroup I included six germplasms (Hrhb, 5-B2-1, fhg, 5-C1-1, N920, and smwgg) with smaller values for all traits. Subgroup II included eight germplasms, namely N916, Gs, Bxg, N923, Gl, 5-C3-2, Fhg, and Cfg, and was characterized by bigger inflorescence and flower traits but smaller or medium branch, leaf, fruit, and seed traits. Subgroup III comprised of five germplasms (i.e. N917, Yx02, Jhg, Smthg, and Smjfg), which had the characteristics of biggest inflorescence and flower traits, and bigger branch, leaf, fruit and seed traits. Subgroup IV comprised of eight germplasms (i.e. Zs4, Jhz, Chg, Smjzg, Nsjt21, Nsjt29, Jhwm, and Jgz8), had the characteristics of bigger branch and leaf traits, smallest inflorescence and flower traits, and medium fruit and seed traits. Subgroup V included nine germplasms (Mg3, Mg1, Kkx, Nsjt32, Zs9, Jgz4, Mg2, Jd3, and Jw1), which had the largest fruit and seeds. The second group included only one germplasm, Smcbg, which had double flowers and was fruitless.
The result of cluster analysis of 35 phenotypic traits ()) has a good agreement with the result of cluster analysis of 18 representative traits ()). When the distance was 10, 19/37 (51%) germplasms groupings were consistent, and this figure increased to 28/37 (76%) when the distance was 5.
Discussion
Phenotypic variation in X. sorbifolium germplasms
Variations in phenotypic traits are based on the variations and interactions between plant genotype and the environment (Santos et al. Citation2012). In this study, the genetic diversity of 35 phenotypic traits, including branch, leaf, inflorescence, flower, fruit, and seed, which were investigated together comprehensively and systematically for the first time, could accurately evaluate the phenotypic diversity of X. sorbifolium germplasms. The coefficient of variation (CV) can measure the degree of dispersion of observed values. The greater the CV value, the higher the degree of dispersion of observed values, and the richer the phenotypic diversity (Li et al. Citation2018). In this study, the CVs for branch, leaf, inflorescence, flower, fruit, and seed traits were at the medium level. The mean CV of leaf traits was 21.45%, greater than the previously reported values of 16.18% (Qi et al. Citation2012), 19.21% (Bai et al. Citation2019), and 17.65% (Ma et al. Citation2019). The mean CV of inflorescence and flower traits was 27.13%, smaller than the previously reported value of 32.64% (Lu et al. Citation2011). The mean CV of fruit traits was 24.65%, smaller than 40.18% (Bai et al. Citation2019), 43.6% (Ma et al. Citation2019), and 28.72% (Chai et al. Citation2013) but larger than the 6.14% (Wang et al. Citation2018) reported previously. The mean CV of seed traits was 21.82%, smaller than 29.19% (Bai et al. Citation2019) but larger than the 10.02% (Wang et al. Citation2018), 14.9% (Ma et al. Citation2019), and 13.57% (Chai et al. Citation2013) previously reported.
Twenty-nine phenotypic traits have moderate to high (> 60%) broad sense heritability (H2), suggesting that these traits are controlled more strongly by genotype, thus it will be beneficial to use these traits with higher H2 value when selecting germplasms (Liang et al. Citation2018).
Correlation between phenotypic traits
Correlation coefficient reveals the degree of correlation between different traits and is thus useful for the selection of multiple characters (Xia et al. Citation2016). Significant correlations were observed within most branch, leaf, inflorescence, flower, fruit, and seed traits, and the results were similar to those in the previous reports (Chai et al. Citation2013; Huang Citation2018; Wang et al. Citation2018; Ma et al. Citation2019; Bai et al. Citation2019; Zhang et al. Citation2019). Significant correlations were also observed among most branch, inflorescence, flower, and leaf traits and among fruit and seed traits. Few branch, inflorescence, flower, and leaf traits including ABD, ID, FL, and CD were positively correlated with some fruit and seed traits. Therefore, the ABD, ID, FL, and CD can be used as the basis for judging the FLD, SLD, etc. The leaf-to-fruit ratio is always a hot topic in economic forestry research and influences the quality and yield of fruits (Jin et al. Citation2016; Sun et al. Citation2019). In this study, LN was negatively correlated with fruit and seed traits, indicating that excessive vegetative growth affects reproductive growth. Some measures should be taken to control the leaf-to-fruit ratio in order to get the optimal value. FN was also negatively correlated with most fruit and seed traits because high proportion of male flowers lead to the consumption of more nutrients, affecting the development of fruit and seed traits.
PCA of phenotypic traits
PCA is an important tool because it reduces a large number of variables into a small number of common or major components that effectively summarize a large portion of variation in a complex dataset (Aït-Sahalia and Xiu Citation2019). The method is very helpful for the selection of multiple traits and in determining the most suitable traits that mostly contribute to the variation in the selected materials (Nardo et al. Citation2005). In this study, the cumulative contribution rate of the first nine principle components reached 80.333%, slightly higher than the results of 74.693% (Bai et al. Citation2019) and 70.260% (Guo et al. Citation2012), and similar to the result of 86.053% (Ma et al. Citation2019) reported previously. The result of cluster analysis of 35 phenotypic traits have a good agreement with the result of the 18 representative traits, indicating that it is feasible and effective to simplify and select the phenotypic traits through PCA analysis and clustering of phenotypic traits. This result can improve the efficiency of evaluation and breeding of X. sorbifolium.
Cluster analysis of X. sorbifolium germplasms
The 37 germplasms were classified into two main groups and six subgroups. This is helpful for their reasonable utilization in the future. Subgroup IV had larger leaf so the germplasms were more suitable for leaf based development, such as leaf teas of X. sorbifolium. Subgroups II and III had larger flowers and inflorescences, so they were more suitable for landscaping and ornamental applications. Subgroup V, with the largest fruits and seeds, showed especially high FW, SFSN, SFSW, FTD, KP, SW, KW KP, and SHGW values, and these traits were highly positively correlated with the fruit and seed yields (Chai et al. Citation2013; Ma et al. Citation2019; Zhang et al. Citation2019; Bai et al. Citation2019). Therefore, the germplasms of subgroup V are suitable for fruit-based applications. The characteristics and classifications of the germplasms agree well with the previous reports, such as that the germplasm Smjfg is a suitable landscaping cultivar as reported by Wang (Wang et al. Citation2020). Cui reported that Zs9 has characteristics like strong tree vigor, long branch, and large fruit (Cui et al. Citation2020).
Conclusion
Large variation and high phenotypic diversity were found among the germplasms of X. sorbifolium. The degree of variation was as follows: branch traits > inflorescence traits > fruit traits > flower traits > leaf traits > seed traits. Significant correlations were observed within and among the phenotypic traits. The cumulative contribution rate of the first nine principle components reached 80.333%, and 18 representative traits were selected by combining the results of correlation, PCA, and clustering of phenotypic traits. The 37 germplasms were classified into two main groups and six subgroups. This study contributes to effective evidence-based conservation and management strategies and provides useful information for genetic improvement and evaluation of germplasms of X. sorbifolium. This research, when combined with molecular marker, would provide a strong base for unravelling the genetic diversity and would also provide valuable phenotypic descriptions for GWAS study on X. sorbifolium in future.
Supplemental Material
Download Zip (24.7 KB)Disclosure statement
No potential conflict of interest was reported by the author(s).
Supplementary material
Supplemental data for this article can be accessed here
Additional information
Funding
References
- Aït-Sahalia Y, Xiu DC. 2019. Principal component analysis of high-frequency data. J Am Stat Assoc. 114:287–303. doi:10.1080/01621459.2017.1401542.
- Bai XL, Jin H, Zou JX, Zhu T, Jin CY, Cheng WQ, Li XL. 2019. Correlation analysis and principal component analysis on phenotypic characteristics in Xanthoceras sorbifolia. Non-wood for Res. 37(1):68–73. Chinese.
- Bi QX, Zhao Y, Cui YF, Wang LB. 2019. Genome survey sequencing and genetic background characterization of yellow horn based on next‑generation sequencing. Mol Biol Rep. 46(4):4303–4312. doi:10.1007/s11033-019-04884-7.
- Chai CS, Lu J, Cai GJ, Wang SY, Qi JL, Wang ZT, Xue R. 2013. Fruit phenotypic diversity and variation of Xanthoceras sorbifolia artificial population. For Res. 26(2):181–191. Chinese.
- Cheng H, Xu YF, Wang WX, Zhu J, Jia RD, Yang SH, Zhao X, Ge H. 2020. Phenotypic diversity of Paphiopedilum micranthum populations from main distribution areas in China. Acta Hortic Sinica. 47(6):1098–1110. Chinese.
- Cui YF, Cui DS, Li HL, Bi QX, Yu HY, Cui TP, Xj L, Wang LB. 2020. New Xanthoceras sorbifolium Cultivars ‘Zhongshi 4 ’and ‘Zhongshi 9. Acta Hortic Sinica. 47(S2):3115–3116. Chinese.
- Das R, Divakara BN. 2011. Variability and divergence in Pongamia pinnata for further use in tree improvement. J For Res. 22(2):193–200. doi:10.1007/s11676-011-0149-9.
- Fu YJ, Zu YG, Wang LL, Zhang NJ, Liu W, Li SM, Zhang S. 2008. Determination of fatty acid methyl esters in biodiesel produced from Yellow Horn oil by LC. Chromatographia. 67:9–14. doi:10.1365/s10337-007-0471-8.
- Guo JZ, Zhang M, Fei ZX, Zhang LL, Jiang XD, Yang M. 2012. Principal component analysis and cluster analysis of quantitative characters of Xanthoceras sobifolia. J North For Univ. 27(2):67–79. Chinese.
- Holland JB, Nyquist WE, Cervantes-Martínez CT. 2003. Estimating and interpreting heritability for plant breeding: an update. Plant Breed Rev. 22:9–112.
- Huang YZ. 2018. Variation of the fruit and seed phenotypic traits and the selection of the comprehensive evaluation of different mating combinations in X. sorbifolium [Dissertation]. Beijing University.
- Jin FL, Zhang FW, Yue X, Li M, Ao XX. 2016. Correlation between leaf size and fruit quality of Kiwi. Agric Sci Technol. 17(11):2469–2472, 2488.
- Li B, Gu WC, Lu BM. 2002. A study on phenotypic diversity of seeds and cones characteristics in Pinus bungeana. Nat Popul Biodivers Sci. 10(2):181–188. Chinese.
- Li HC, Gan XH, Zhang ZP, Zhang CX, Song L. 2015. Effects of altitudes and the DBH of seed trees on biological characteristics of Tetracentron sinense (Tetracentraceae) seeds. Plant Divers Resour. 37(2):177–183.
- Li YG, Liu XH, Ma JW, Zhang XM, Xu LA. 2018. Phenotypic variation in Phoebe bournei populations preserved in the primary distribution area. J For Res. 29(1):35–44. doi:10.1007/s11676-017-0409-4.
- Li Y, Li S, Lu XH, Wang QQ, Han HY, Zhang XM, Ma YH, Gan XH. 2020. Leaf phenotypic variation of endangered plant Tetracentron sinense Oliv and influence of geographical and climatic factors. J For Res. doi:10.1007/s11676-020-01124-8.
- Liang DY, Ding CJ, Zhao GH, Leng WW, Zhang M, Zhao XY, Qu GZ. 2018. Variation and selection analysis of Pinus koraiensis clones in northeast China. J For Res. 29(3):611–622. doi:10.1007/s11676-017-0471-y.
- Lu J, Chai CS, Cai GJ, Wang SY, Qi J, Wang ZT, Xue R. 2011. Phenotypic diversity of flowers of Xanthoceras sorbifolia Bunge. in DingXi area of Gansu. For Resour Manage. 10(6):49–53. Chinese.
- Ma X, Jian JY, Li M. 2019. Analysis on growth and fruiting characteristics of Xanthoceras sorbifolia from 24 provenances in Xinjiang. Non-wood for Res. 37(1):61–67. Chinese.
- Mohamed MS, Mohammed EH, Nadia W, Aziz O, Abdelali B, Abdelmajid H. 2009. Fruit and seed diversity of domesticated carob (Ceratonia siliqua L.) in Morocco. Sci Hortic. 123(1):110–116. doi:10.1016/j.scienta.2009.07.009.
- Nardo M, Saisana M, Saltelli A, Tarantola S. 2005. Tools for composite indicators building. Eur Comission Ispra. 15:19–20.
- Piepho HP, Möhring J. 2007. Computing heritability and selection response from unbalanced plant breeding trials. Genetics. 177:1881–1888. doi:10.1534/genetics.107.074229.
- Qi JL, Chai CS, Lu J, Wang ZT, Xue R, Wang SY, Cai GJ. 2012. Branch and leaf phenotypic diversity of artificial Xanthoceras sorbifolia population in Gansu. For Resour Manage. 8(4):66–69.Chinese.
- Rong W, Sun Z, Li Q, Liu R, Zhang T, Wang T, Yang W, Li Z, Bi K. 2016. Characterization and simultaneous quantification of seven triterpenoid saponins in different parts of Xanthoceras sorbifolia Bunge by HPLC-ESI-TOF. Anal Methods. 8:2176–2184. doi:10.1039/C5AY02813B.
- Santos RC, Pires JL, Correa RX. 2012. Morphological characterization of leaf, flower, fruit and seed traits among Brazilian Theobroma L. species. Genet Resour Crop Evol. 59:327–345. doi:10.1007/s10722-011-9685-6.
- Schaal BA, Leverich WJ, Rogstad SH. 1991. A comparison of methods for assessing genetic variation in plant conservation biology. In: Falk DA, Holsinger KE, editors. Genetics and conservation of rare plants. New York: Oxford University Press; p. 123–124.
- Sun X, Shan SM, Xu YL, Liu CM, Li YL. 2019. Effect fomparison of leaf-berry ratio of ‘Cabernet Franc’ berry quality. Northern Horticulture 9 . (8):53–58. Chinese.
- Venegas-Calerón M, Ruíz-Méndez MV, Martínez-Force E. 2017. Characterization of Xanthoceras sorbifolium Bunge seeds: lipids, proteins and saponins content. Ind Crops Prod. 109:192–198. doi:10.1016/j.indcrop.2017.08.022.
- Wang X, Zheng YQ, Su SC, Ao Y. 2019. Discovery and profiling of microRNAs at the critical period of sex differentiation in Xanthoceras sorbifolium Bunge. Forests. 10:1141. doi:10.3390/f10121141.
- Wang YL, Jia QX, Li Y. 2020. A new landscaping Xanthoceras sorbifolium cultivar ‘Senmiao Jinfengguan’. Acta Hortic Sinica. 47(12):2463–2464. Chinese.
- Wang YL, Lyu D, Liu XD, Li XY, Zhao GS, Liu JH. 2018. Analysis on variation of phenotypic traits of Xanthoceras sorbifolia fruit and seed of five crown fruits provenances. Non-wood For Res. 36(3):38–47.Chinese.
- White TL, Hodge GR. 1989. Predicting breeding values with applications in forest tree improvement. The Netherlands: Kluwer Academic Publishers; p. 90.
- Xia H, Zhao GH, Zhang LS, Sun XY, Yin SP, Liang DY, Li Y, Zheng M, Zhao XY. 2016. Genetic and variation analyses of growth traits of half-sib Larix olgensis families in northeastern China. Euphytica. 212:387–397. doi:10.1007/s10681-016-1765-4.
- Yang XY, Fu MY, Xie JZ, Li ZC. 2009. Geographic variation and provenance selection for bamboo wood properties in Bambusa chungii. J For Res. 20(3):261–267. doi:10.1007/s11676-009-0045-8.
- Yu HY, Fan SQ, Bi QX, Wang SX, Hu XY, Chen MY, Wang LB. 2017. Seed morphology, oil content and fatty acid composition variability assessment in yellow horn (Xanthoceras sorbifolium Bunge) germplasm for optimum biodiesel production. Ind Crops Prod. 97:425–430. doi:10.1016/j.indcrop.2016.12.054.
- Zhang Y, Ao Y, Liu JF, Zhao LL, Zhang YM. 2019. Variation pattern of fruit and seed characters and superior individual selection of Xanthoceras sorbifolium. J North For Univ. 47(9):1–5. Chinese.
- Zhou QY, Cai Q. 2018. The superoxide dismutase genes might be required for appropriate development of the ovule after fertilization in Xanthoceras sorbifolium. Plant Cell Rep. 37:727–739. doi:10.1007/s00299-018-2263-z.