ABSTRACT
Climate change increases air and soil temperatures and the frequency of extreme weather events such as high temperatures, droughts, and flooding. These changes negatively impact forest ecosystems and the provision of ecosystem services such as timber supply and carbon sequestration. Fine roots and associated mycorrhizae play an essential role in the functioning of forest ecosystems because they absorb water and nutrients from the soil and owing to their short lifespan, significant amounts of carbon and nutrients are transferred through them into the soil. Here, we reviewed the existing literature on the responses of tree fine roots and root systems to increasing soil temperature and changes in soil water availability and waterlogging. We also discussed how the negative responses of fine roots to environmental changes can be mitigated in forest management operations by selecting tree species and provenances, growing mixed tree species stands, carrying out continuous-cover forestry, and avoiding rutting and soil compaction when using forest vehicles. Our main focus is on the root systems of boreal and temperate forests. Knowledge of root responses to changing environmental conditions and different management practices, which could be used to mitigate negative effects, is still very limited and requires more research and development before they can be introduced in practice.
Introduction
Forest ecosystems provide many important services, including carbon (C) sequestration and the supply of timber and fuelwood. Forests contribute significantly to global C (Pan et al. Citation2011) and hydrological cycles (Creed and van Noordwijk Citation2018). Forests fix C from the atmosphere and store significant amounts of C in biomass and soil. The roots and crowns of trees and understory vegetation capture large amounts of water that is released by evapotranspiration from the canopies. Globally, ~30% of all forests are managed primarily for the production of wood and non-wood forest products (FAO Citation2020). In Europe, the share is 53%, and in Asia, large forest areas are also designated for soil and water protection, and in America, for multiple uses (FAO Citation2020). In forests managed primarily for wood production, cuttings, regeneration, drainage, and fertilization operations are regularly performed to improve forest growth and yield. The functioning of root systems is significantly affected by forest management operations and changes in environmental factors such as soil temperature, moisture, and aeration. The challenges caused by climate change can be considered in forest management operations to mitigate negative effects.
This article aimed to review the existing literature on the responses of fine roots and root systems to changing environmental conditions to form a knowledge basis for the management of forests to maintain or improve the functioning of roots and whole trees in future climates. Roots play an essential role in the functioning of forest ecosystems and the global C cycle (McCormack et al. Citation2015; Adamczyk et al. Citation2019). Root systems consist of stumps, coarse roots, transport fine roots, and absorptive fine roots, including mycorrhizae (McCormack et al. Citation2015). Stumps, coarse roots, and transport fine roots are needed to stabilize trees with soil reinforcement (Schwarz et al. Citation2010) and to conduct water, nutrients, and photosynthates between the above- and below-ground parts of trees. Absorptive fine roots, which are composed of lower-order roots defined as first- or second-order roots in the branch-order-based classification and are located in the most distal parts of the root system, are responsible for water and nutrient uptake from the soil (McCormack et al. Citation2015). Due to the short lifespan of fine roots, particularly absorptive fine roots, significant amounts of C and nutrients are transferred to the soil. Globally, the production and turnover of fine roots, including both absorptive and transport fine roots, represent 22% of terrestrial net primary production (McCormack et al. Citation2015). Lower-order absorptive roots are generally more sensitive to stress than higher-order roots, that is, transport fine roots (Doi et al. Citation2020; Repo et al. Citation2020). Absorptive fine-root function is linked to various measurable root traits (e.g. Freschet et al. Citation2021, Citation2021; Wang et al. Citation2021). For example, root diameter and specific root length are closely related to root lifespan and respiration, which contribute significantly to C cycle in forests (Weemstra et al. Citation2020). Fine-root biomass, production, and mortality are traits that can be used to evaluate forest responses to climate change.
Water uptake by forest vegetation reduces soil moisture, groundwater recharge, and runoff to watercourses in most forested regions (Creed and van Noordwijk Citation2018). The functioning of forest ecosystems is affected by varying and changing climatic conditions, that is changes in atmospheric CO2 concentration, air and soil temperatures, and the amount of precipitation (IPCC Citation2022). The increase in mean annual air temperature will continue in the boreal and temperate forest regions, whereas the changes in the amount of precipitation are likely to vary both spatially and temporally (IPCC Citation2022). The mean annual precipitation is projected to increase in the boreal region and mostly in winter (IPCC Citation2022). Precipitation in winter, whether as rain or snow, strongly affects soil temperature and moisture and thereby also to root systems (Repo et al. Citation2014). Snow cover isolates soil and may prevent soil from freezing. It protects roots from freezing injury, frost heaving damages and the impaired water uptake of roots in cold soil in spring and early summer when evapotranspiration demand is high. In general, increases in the mean air temperature, atmospheric CO2 concentration, and water availability have positive impacts on forest production in high-latitude forests (IPCC Citation2022). In many temperate forests, precipitation will decrease, and together with increasing temperature, will lead to long dry periods and reduced forest growth (IPCC Citation2022).
In addition to the gradual long-term changes in air temperature and the amount of precipitation, extreme weather events have become more frequent, long-lasting, and intensive in all regions (IPCC Citation2022). These extreme weather events cause stresses to the forests, which are first projected to the rhizosphere and through the roots to the functioning of aboveground organs and the entire forest ecosystem. Forests may either tolerate and recover from the impacts of these harmful conditions or, at worst, experience forest die-off (IPCC Citation2022).
In this article, we first briefly review what is known about fine-root responses to increasing soil temperature, changes in soil water availability, and waterlogging. We then present how negative responses can be mitigated through different management operations. We highlight that for the growth of trees and the functioning of forest ecosystems, consideration of the interactions between roots, mycorrhizas, and the above-ground parts is also essential. Our main focus is on root systems in temperate and boreal forests.
Fine-root responses to changing climate
Increasing air and soil temperatures
An increase in the mean annual air temperature, and consequently, an increase in soil temperature, affects various fine-root traits. Tree fine-root biomass, growth, water and nutrient uptake, and root respiration increase, whereas C allocation to roots decreases when there are no limitations on other growth factors (Pregitzer et al. Citation2000; Wang et al. Citation2021). Furthermore, fine-root turnover and mortality increase, and fine-root lifespan decreases, but this is not always clear (Leppälammi-Kujansuu et al. Citation2014; Wang et al. Citation2021). The effects of warming on fine roots are dependent on the season, temperature range, and duration and magnitude of the temperature change (Wang et al. Citation2021). Biomass, growth, and mortality responses may also be delayed, or may gradually reverse from positive to negative (Repo et al. Citation2014; Wang et al. Citation2021).
In all forest biomes, the majority of roots are located in the surface soil horizons (Jackson et al. Citation1996; Schenk and Jackson Citation2002) (). Here, they are exposed to greater daily and seasonal temperature fluctuations than those in deeper soil horizons (Pregitzer et al. Citation2000). Warming increases soil temperatures, and the effects are more positive for roots growing in deeper (>20 cm) soil horizons than for those growing near the surface. High temperatures in surface soil horizons may even become detrimental and shift fine-root biomass allocation deeper into the soil (Wang et al. Citation2021). Higher root production at deeper soil depths in the future may also be a consequence of the increased CO2 concentration in the atmosphere, which increases C availability for root growth and resource demand from the soil, and consequently decreases resource availability in the surface soil horizons owing to high root competition (Iversen Citation2010; Germon et al. Citation2020). In the permafrost forest, soil surface will thaw deeper in the future climate, which will increase the biomass and depth distribution of fine roots (Noguchi et al. Citation2016). Generally, tree fine-root response studies cover only the upper part of the full rooting depth (Finér et al. Citation2011), therefore we need to further focus on fine-root responses to increasing temperatures in deeper soil layers (Germon et al. Citation2020; Wang et al. Citation2021).
Figure 1. A fallen Norway spruce in boreal forest. The root system was mostly located within 30-50 cm from the soil surface. Photo: L. Finér.
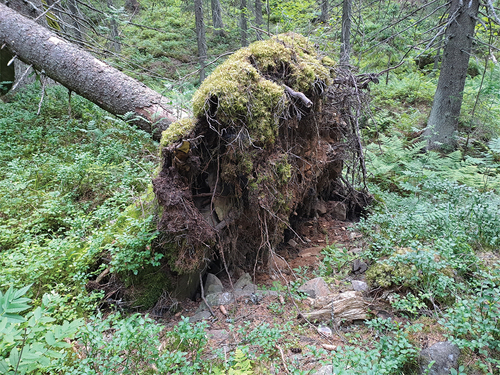
In high-latitude and high-altitude forests, the soil surface can freeze during winter, especially if there is no insulating snow cover (Helama et al. Citation2011). This can increase fine-root mortality (Groffman et al. Citation2001; Repo et al. Citation2014; Domisch et al. Citation2017). The fine roots of the boreal tree species develop freezing tolerance, but their tolerance is lower than that of the needles (Ryyppö et al. Citation1998; Wu et al. Citation2023). For example, in one study, fine roots of Scots pine (Pinus sylvestris L) seedlings developed a freezing tolerance of −5°C, while needles could tolerate −20°C or lower temperatures (Ryyppö et al. Citation1998). In the future, soil may remain unfrozen at sites that are exposed to soil freezing in the current climate, thus decreasing the susceptibility to root mortality. Changes in snow cover may confuse this concept, because decreased snow cover may lead to more soil freezing in areas where temperatures below zero may still exist in winter. The positive effect of warming on fine-root biomass has been found to increase with latitude under conditions where root growth is limited by low soil temperatures (Wang et al. Citation2021).
Roots have periodicity in growth and mortality, which is closely related to soil temperature variation, although there are also other factors, such as above-ground phenology, that determine the seasonality of root growth (Lyr and Hoffmann Citation1967; Pregitzer et al. Citation2000). Fine roots of boreal and temperate conifer (Picea abies, Pinus sylvestris, P cembra) and deciduous tree species (Alnus viridis, A. glutinosa, Betula pendula) are observed to start growing in spring when the mean daily soil temperature reaches 6–8°C (Vapaavuori et al. Citation1992; Alvarez-Uria and Körner Citation2007), and conditions are optimal at 17–20°C (e.g. Domisch et al. Citation2001). In the current climate, temperatures seldom reach such high levels in boreal forest soils (Kubin and Kemppainen Citation1994). It is probable that in the future, root growth will start earlier in spring and continue longer in autumn, resulting in higher overall fine-root biomass production in boreal forests. The positive response of fine-root biomass to experimental warming has decreased with increasing mean annual temperature (Wang et al. Citation2021). This suggests that fine-root responses to warming are stronger in boreal than in more southern environments.
In general, native tree species are adapted to the prevailing climatic conditions and are in temperate and boreal regions exposed to large seasonal and between-year air temperature variations. However, the survival of species in significantly warmer climates depends on their phenotypic plasticity and genetic capacity to adapt to new conditions (Savolainen et al. Citation2007). Climate change is already shifting vegetation zones towards the poles and upwards in the mountains (Gottfried et al. Citation2012; Lenoir and Svenning Citation2015). However, the role of fine roots in the adaptation process remains poorly understood.
Drought and waterlogging
Future climate conditions will expose tree roots to drought and waterlogging more frequently in many regions. Fine-root growth decreases in dry and in waterlogged soils, where limited water or oxygen availability may increase root mortality (Glenz et al. Citation2006; Brunner et al. Citation2015; Repo et al. Citation2016, Citation2020). In waterlogged soils, not only the lack of oxygen but also the accumulation of toxic metabolites (aldehydes, organic acids, ethanol) and high CO2 and methane concentrations are detrimental to roots (Glenz et al. Citation2006; Domisch et al. Citation2020). Different tree species vary in their tolerance to water availability stress, depending on season, developmental stage, and the possibility to acclimate anatomically, morphologically, or metabolically to dry or anoxic conditions (Glenz et al. Citation2006; Niinemets and Valladares Citation2006). The most common response of trees to these stresses is to reduce fine-root biomass and shoot-to-root biomass ratio (Brunner et al. Citation2015; Repo et al. Citation2016, Citation2020). The morphological and chemical traits of fine roots are only slightly affected, indicating that tree roots have strategies to coordinate the metabolic and structural demands required to acclimate and maintain physiological and morphological functions under drought conditions (Brunner et al. Citation2015).
Trees may adjust water and oxygen availability in the soil by altering their rooting depth. In areas where the soil is frequently dry on the surface horizon, trees grow roots deeper into more moist soil horizons, whereas, in areas where the water table is close to the soil surface, root growth is concentrated in the oxygenated upper soil horizons (Glenz et al. Citation2006; Fan et al. Citation2017; Fujita et al. Citation2021). However, the ability to grow roots at different soil depths to access water is species-specific (Nardini et al. Citation2016). Liu and Biondi (Citation2021) showed that aspen allocates its water uptake fine roots to surface soil horizons, whereas conifers at the same sites grow their roots in deeper soil horizons to access water. This makes aspen more vulnerable to drought damage than conifers.
In the boreal zone, precipitation is projected to increase especially during the dormant period, which may increase snowfall, thaw-freeze events, and the risk of waterlogging in soil. Heavy and long-lasting rainfall increases the frequency of flooding in many forested regions. Flooding can cause soil hypoxia. The sensitivity of fine roots to hypoxia varies depending on the degree (depth of the water table) and timing of the waterlogging and its duration (Glenz et al. Citation2006; Sikström and Hökkä Citation2016; Roitto et al. Citation2019; Repo et al. Citation2020). The most harmful situation is long-lasting waterlogging during the growing season, when also high soil temperatures decrease the solubility of oxygen in water at the same time when oxygen demand of roots is high (Glenz et al. Citation2006; Repo et al. Citation2016, Citation2020). Adult trees tolerate hypoxia better than seedlings, and angiosperms, which have aerenchyma tissues (e.g. Salix sp.), are more tolerant than gymnosperms; however, these differences have not been fully tested in the field (Glenz et al. Citation2006). The tolerance of species to multiple stresses is not common; species that are tolerant to drought are often not tolerant to waterlogging, and vice versa (Niinemets and Valladares Citation2006). The drought tolerance of trees also varies with age; seedlings are less tolerant to drought than saplings and adult trees (Niinemets and Valladares Citation2006).
Managing forests for roots in future climate
Forest management activities have the potential to affect the temperature and moisture conditions in forests and mitigate the negative effects of climate change on forests and root systems. This can be attained by selecting management systems between even-aged or continuous cover forests, between mixed and single-species forests, reducing tree stand density by thinning operations and increasing soil drainage in waterlogged conditions (see, e.g., Pretzch Citation2005; Keenan Citation2015). Current forestry is highly mechanized, and the avoidance of the negative effects of vehicles on fine roots, and overall, on forests, will be dependent on the skills and planning tools of managers. In forest management, it is challenging to consider long rotation periods of forest stands. During long rotation periods, climate will change, and forests will be exposed to the consequences of numerous extreme weather events. It is difficult to select the best management chains for the uncertain future. Adaptive forest management actions can be aimed at reducing tree and rhizosphere vulnerability to extreme weather events or at increasing the resilience and capacity of forests to respond to progressive climate change and regime shift of tree species (Keenan Citation2015). In the following sections, we discuss some forest management options that we believe can be used to improve the adaptation of trees and forest ecosystems to the future climate through functioning of the rhizosphere.
Selection of tree species
Native plant species are, in general, adapted to local conditions, where they are exposed to large seasonal and annual variations (Savolainen et al. Citation2007; Leimu et al. Citation2008; Hereford Citation2009). The observed shift of vegetation zones northward and upward in the mountains indicates that species move to climatic conditions they are adapted to, or they genetically adapt to the changing climatic conditions, e.g. by changing their growth periodicity and drought or cold tolerance (e.g. Alberto et al. Citation2013; Aitken and Bemmels Citation2015). Changes in climatic conditions are mostly gradual, but still too rapid, and plant species should be able to migrate 1000 m or more per year to track changing environmental conditions (Malcolm et al. Citation2002). Past migration rates were much lower than that, ~100 m per year. The migration rates of tree species are lower than those of herbaceous plants because of their low reproductive capacity and high maturity age. It is not only gradual changes in mean annual air temperatures or precipitation but also changes in the occurrence of extreme weather events that affect species resilience and survival in future climates. Tree die-offs due to increased temperature and/or water stress have been documented globally in different forest ecosystems (Allen et al. Citation2010, Citation2015).
Forest managers should consider climate change when selecting tree species for breeding, afforestation, or thinning operations. The local provenances of tree species spreading over large areas with large population sizes, good reproductive capacity, and phenotypic plasticity are expected to have good tolerance to changing climatic conditions (Leimu et al. Citation2008; Alberto et al. Citation2013; Leites and Benito Garzón Citation2023). Therefore, they may be proper choices for use in near-future forests. However, this conclusion, which is based on studies without a focus on roots or their associations with mycorrhizas, may be too generalized when their adaptations are considered and need to be further studied. Adaptation may also be improved by selecting tree species with high genetic diversity, including genotypes from more southern locations, which are adapted to near-future climatic conditions, and by selecting tree species from local genotypes that tolerate extreme weather conditions, such as high and low temperatures or drought (Aitken and Bemmels Citation2015; Leites and Benito Garzón Citation2023).
Populations of tree species that grow along wide climatic gradients adjust their root systems to various environmental conditions (Zadworny et al. Citation2016; Tenkanen et al. Citation2021). However, it is important to remember that so far transfers are in general recommended only across short latitudinal distances to avoid abiotic and biotic risks (Aitken and Bemmels Citation2015). Especially, trees growing close to their northern margins are adapted to local harsh conditions, but their genetic adaptation capacity may be limited (Aitken et al. Citation2008; Alberto et al. Citation2013) When selecting species and provenance for forest plantations, adaptation at the seedling stage is critical because adult trees tolerate stress better than seedlings (Aitken and Bemmels Citation2015). The establishment and growth of tree seedlings are affected by the colonization of fine roots by specific arbuscular or ectomycorrhizal fungi (Jones et al. Citation2003; Wu et al. Citation2019; Seiwa et al. Citation2020), and the opportunity for appropriate symbiosis between fine roots and mycorrhizae could determine the survival of seedlings in the new environment. More systematic and site-specific research including also focus on root system responses is needed to assist foresters to migrate tree species plantations towards the future climate. In North America, an interesting framework called Desired Regeneration through Assisted Migration (DREAM) was recently established to provide a scientific basis for successful migration of tree species for forestry (Royo et al. Citation2023). The DREAM framework could also offer a good opportunity to study below-ground responses to assist tree species migration.
Controlling tree stand density
In forest management, the reduction of tree stand density varies from operations where all trees are removed at clear-cutting to operations where stand density is reduced by thinning to decrease competition and increase the availability of light and soil resources for the seedlings/remaining trees. In addition to these two methods, forest managers use various other management systems that affect tree stand density to different degrees, such as seed trees, shelterwood, and gap and strip cutting methods. In thinned stands, trees have space to develop more extensive root systems over time, which may improve their tolerance to stressful conditions. Clear-cutting changes soil temperature, moisture, and chemical environment, which affect the species composition of mycorrhizal fungal communities associated with fine roots (Kranabetter and Kroeger Citation2001; Jones et al. Citation2003). This compositional change can induce an increase, decrease, or no change in seedling growth (Jones et al. Citation2003), therefore more research is needed to apply these findings in practice.
In dense stands, fine roots take up significant amounts of water from the soil and decrease soil moisture levels (e.g. Verstraeten et al. Citation2005), which may amplify the negative impacts of dry conditions. The reduction in stem density caused by heavy thinning may decrease drought stress (Sohn et al. Citation2016). However, under hot and dry conditions, clear-cuts should be avoided because water evapotranspiration of forest canopy has a cooling effect of several degrees (Hesslerová et al. Citation2013), which, together with the shading effect of the crowns, creates a forest microclimate with lower and less extreme temperature conditions than those outside the crown cover (De Frenne et al. Citation2013, Citation2021; Zellweger et al. Citation2020).
Clear-cutting makes temperature conditions more extreme, especially the high summer temperatures that will increase, and probably more so in the future climate (Zellweger et al. Citation2020; De Frenne et al. Citation2021). Depending on site conditions, high temperatures and low precipitation may have negative effects on seedling growth. In the boreal zone, where temperatures currently limit the growth of seedlings, soil scarification is performed to create microsites above the soil surface that provide better temperature conditions for seedling growth than undisturbed microsites (Kubin and Kemppainen Citation1994). The temperature increase at these microsites may correspond to a 100 km shift to the south of the site. In the future, there may be a risk that the temperature and moisture conditions will become too extreme for growth and survival of roots in the surface soil horizons of clear-cuts, causing problems in establishing forest plantations even in the boreal regions with permafrost.
There are sites where thinning and clear-cutting can increase soil moisture and raise the water table to levels at which the roots begin to suffer from hypoxia. This is the case for peatlands and wet mineral soils that cover large areas of boreal regions. Millions of hectares of peatlands were drained in the twentieth century, mostly in Fennoscandia, Russia, and the Baltic countries, to increase forest growth (Päivänen and Hånell Citation2012). To maintain good drainage in these forests, the ditches need to be cleaned at 20–40 year intervals and clear-cut to maintain proper soil oxygen content for the growth of fine roots (). The frequency of ditch cleaning can be reduced by avoiding clear-cutting and maintaining continuous tree cover, which can keep soil water table low enough for good aeration in soil by crown evapotranspiration and interception (Sikström and Hökkä Citation2016; Leppä et al. Citation2020).
Figure 2. Old drainage area in central Finland. Ditches have been recently cleaned. There is a sedimentation pond constructed to trap suspended solids and reduce their outflow to watercourses. Photo: L. Finér.
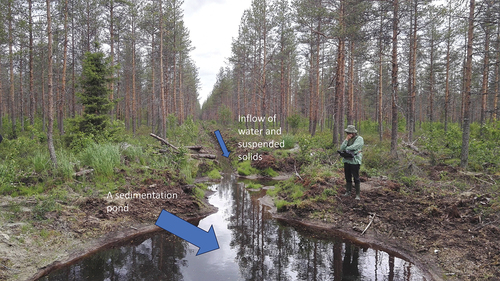
In the future, continuous cover forest management systems can maintain microclimates that are more stable and favorable for fine-root growth than management systems that include clear cuttings during the regeneration phase of the forest (). However, continuous cover management systems reduce the possibility of selecting tree species, utilizing the potential of tree breeding, and favoring mixed tree species stands, since regeneration usually relies on the seed production of the species already existing in the stands. In many cases, early successional light-demanding species do not regenerate under closed canopies. This may lead to stands composed of late-successional species, which are generally less adapted to water stress and higher temperatures than are early-successional species (Niinemets and Valladares Citation2006; Kolström et al. Citation2011). To reduce the risk of continuous cover forestry, both early and late successional species should be retained in the forest during management operations, if feasible.
Mixed tree species vs single tree species stands
Natural and semi-natural forests are generally composed of different tree species, whereas plantation forests are typically composed of a single tree species. In Europe, approximately 67% of forests are composed of two or more tree species, and the remaining 33% contain only one tree species, mainly conifers (Forest Europe Citation2020) (). Mixed forest stands are regarded as more tolerant to drought than single-species stands, although species identity, site, and stand factors cause variation in this relationship (Grossiord et al. Citation2014; Sousa‐Silva et al. Citation2018; Grossiord Citation2019; Pardos et al. Citation2021). The mechanisms related to roots explaining the better performance of mixed forests are the partitioning of water resources owing to the occupancy of roots of different tree species in different soil horizons, facilitation between species owing to the uplifting of water by deep-rooted species to the species in which roots grow in the surface soil horizons, and the presence of highly water-stress-tolerant species in mixtures (Grossiord Citation2019). On the other hand, the enhanced growth observed in mixed stands may increase root water uptake and evapotranspiration, especially in stands with high basal areas, thereby increasing susceptibility to drought stress (Grossiord Citation2019). Tree species mixtures are commonly thought to increase drought tolerance, especially in drought-prone environments, e.g. by partitioning roots and thereby their water uptake in different soil layers, by actively redistributing water in soil by roots and by increasing a change to contain deep-rooted and anisohydric species (Grossiord Citation2019; Pardos et al. Citation2021). In addition to altered soil water and temperature conditions, nutrient availability should also be considered in mixtures of different tree species. In conifer forests, significant hardwood content enhances the availability of soil inorganic nitrogen, by facilitating leaf litter decomposition and soil nitrogen mineralization (Masuda et al. Citation2022).
Figure 4. Mixed forest stands. Left, temperate forest in Poland (species Picea abies (L.) karst., Pinus sylvestris L., Betula pendula Roth., Quercus robur L.); right, boreal forest in Finland (species: Picea abies (L.) Karst., Pinus sylvestris L., Betula pendula Roth./B. pubesecens Ehrh). Photos: L. Finér.
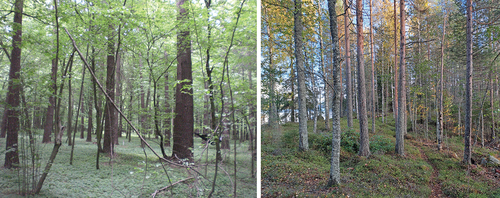
In the future, continuous-cover forests with a mixed species composition are likely to be more resistant to low water availability and other environmental stresses than single-species stands (Pardos et al. Citation2021). In European forests, mixtures of conifer-broadleaved species could be favored because they are more resistant to drought than mixtures of deciduous species (Pardos et al. Citation2021). When selecting tree species for mixed forest stands, the natural resistance of tree species to drought and their ability to take water from different soil horizons must be considered (Liu and Biondi Citation2021). More research and developmental work in terms of root systems are required to establish and manage proper mixed tree species stands for wood production or other ecosystem services because of species differences in light demand and growth rhythms as well as their susceptibility to biotic damage, such as browsing by cervids at a young age (Huuskonen et al. Citation2021). We also need to know more about the fine-root responses in tree species mixtures.
Use of vehicles in forest operations
Modern forest management operations are highly mechanized. Powerful ground-based heavy vehicles are used to harvest forests, transport timber, and prepare soil for planting. Heavy vehicles can cause long-term rutting and soil compaction in their tracks, covering part of the operation site (). Forest soils in tropical and temperate zones are more susceptible to negative effects than soils in dry and cold areas (Nazari et al. Citation2023). In the future, higher precipitation and shorter periods of frozen soil will increase the risk of soil compaction at northern latitudes. The review by Cambi et al. (Citation2015) and meta-analyses by Mariotti et al. (Citation2020), Nazari et al. (Citation2021, Citation2023), and Latterini et al. (Citation2023) comprehensively analyzed the results of the existing literature on the impacts of heavy vehicles on forest soils and ways to reduce the negative impacts. In the following sections, we refer to these from the perspective of roots.
Figure 5. Left, soil rutting and compaction caused by a forwarder. Right, a forwarder equipped with bogies piling logs at a clear-cut area. Bogies around the tires reduce pressure to soil surface and diminish soil damages. Photo: L. Finér.
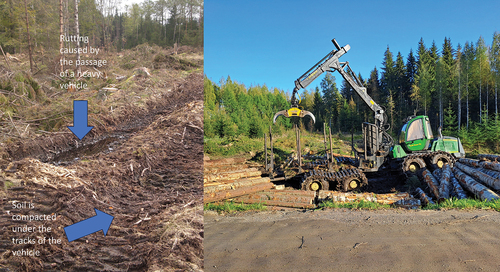
Soil compaction reduces water and oxygen conductivity and infiltration in forest soil, increases waterlogging in flat areas, decreases soil air space, cuts roots, increases the penetration resistance of soil, and causes erosion of slopes (Cambi et al. Citation2015, Nazari et al. Citation2021), all of which have negative impacts on the root and shoot growth of seedlings (Cambi et al. Citation2015; Mariotti et al. Citation2020; Latterini et al. Citation2023). These impacts on roots are stronger at surface soil horizons than deeper in the soil, and they decrease the rooting depth of planted seedlings (Mariotti et al. Citation2020). In most soils, compaction reduces water and oxygen availability, decreases root growth, and increases plant mortality (Cambi et al. Citation2015). Soil disturbance by heavy vehicles can be positive only in very coarse-textured soils, where the decrease in water infiltration and hydraulic conductivity may increase water availability to roots, and to some extent, also in soils with thick organic layers, where the mixing of organic horizons with mineral soil may improve water and nutrient availability (Cambi et al. Citation2015). Recovery from soil compaction may take years or even decades, depending on the soil texture, organic matter content, and environmental conditions (Cambi et al. Citation2015; Latterini et al. Citation2023). Recovery is faster in the surface soil horizons than in the deeper soil, which also affects the root depth distribution.
In the future, compaction and rutting damages will increase, especially in areas where soils are wet and do not freeze in winter and in areas with soils that have low bulk densities. For example, in organic and fine-textured mineral soils. Several approaches have been proposed to avoid or reduce soil compaction and rutting and, consequently, soil and root damage (Cambi et al. Citation2015; Nazari et al. Citation2021, Citation2023; Latterini et al. Citation2023). These include the use of lighter vehicles and bogie tracks instead of tires (), decreasing traffic passages in time and space, application of logging residues on logging tracks, timing of operations to dry seasons or times when soils are frozen, and planning vehicle tracks to locations with good bearing capacity. Driver education is also essential for reducing damage.
Knowledge of the susceptibility of operation sites to rutting and soil compaction forms the basis for planning measures to avoid negative impacts. Maps are used as decision support tools to indicate spatial and temporal variations in the bearing capacity of soils at the operation site; this is operational in Nordic countries to help plan transportation roads to locations where they cause less damage and timing the operations to periods of dry or frozen soil (Kankare et al. Citation2019; Salmivaara et al. Citation2017, Citation2020, https://www.metsakeskus.fi/korjuukelpoisuuskartat). Maps are useful tools for drivers when performing operations.
Conclusions
Global warming, together with the increasing frequency of extreme weather events (high and low temperatures, drought, and flooding), will challenge the functioning of root systems in the future. Based on the current knowledge, forest managers can take actions to mitigate the negative effects on fine roots by using local, drought/waterlogging-tolerant tree species and tree genotypes, which roots systems can adapt to drought or waterlogging, selecting tree genotypes whose annual cycle is synchronized with the new seasonal variation of climatic factors, favoring mixed tree species stands, favoring deciduous tree species, carrying out continuous cover forestry, avoiding soil compaction and rutting, and developing decision support tools and training experts for the future. Researchers should provide more knowledge on the responses of the fine roots and mycorrhizas of tree species to changing environmental conditions and work together with forest managers to develop forest management practices for future forests.
Acknowledgements
We thank the Japanese Society of Root Research for providing us with the opportunity to write this manuscript.
Disclosure statement
No potential conflict of interest was reported by the author(s).
Additional information
Funding
References
- Adamczyk B, Sietiö OM, Straková P, Prommer J, Wild B, Hagner M, Pihlatie M, Fritze H, Richter A, Heinonsalo J. 2019. Plant roots increase both decomposition and stable organic matter formation in boreal forest soil. Nat Commun. 10(1):3982. doi: 10.1038/s41467-019-11993-1. PMID: 31484931; PMCID: PMC6726645.
- Aitken SN, Bemmels JB. 2015. Time to get moving: assisted gene flow of forest trees. Evol Appl. 9(1):271–290. doi: 10.1111/eva.12293.
- Aitken SN, Yeaman S, Holliday JA, Wang TL, Curtis-McLane S. 2008. Adaptation, migration or extirpation: climate change outcomes for tree populations. Evol Appl. 1(1):95–111. doi: 10.1111/j.1752-4571.2007.00013.x.
- Alberto FJ, Aitken SN, Alia R, Gonzalez-Martinez SC, Hänninen H, Kremer A, Lefevre F, Lenormand T, Yeaman S, Whetten R, et al. 2013. Potential for evolutionary responses to climate change – evidence from tree populations. Glob Chang Biol. 19(6):1645–1661. doi: 10.1111/gcb.12181.
- Allen CD, Breshears DD, McDowell NG. 2015. On underestimation of global vulnerability to tree mortality and forest die-off from hotter drought in the anthropocene. Ecosphere. 6(8):1–55. doi: 10.1890/ES15-00203.1.
- Allen CD, Macalady AK, Chenchouni H, Bachelet D, McDowell N, Vennetier M, Kitzberger T, Rigling A, Breshears DD, Hogg EH, et al. 2010. A global overview of drought and heat-induced tree mortality reveals emerging climate change risks for forests. For Ecol Manage. 259(4):660–684. doi: 10.1016/j.foreco.2009.09.001.
- Alvarez-Uria P, Körner C. 2007. Low temperature limits of root growth in deciduous and evergreen temperate tree species. Func Ecol. 21(2):211–218. doi: 10.1111/j.1365-2435.2007.01231.x.
- Brunner I, Herzog C, Dawes MA, Arend M, Sperisen C. 2015. How tree roots respond to drought. Front Plant Sci. 6:547. doi: 10.3389/fpls.2015.00547.
- Cambi M, Certini G, Neri F, Marchi E. 2015. The impact of heavy traffic on forest soils: a review. For Ecol Manage. 338:124–138. doi: 10.1016/j.foreco.2014.11.022.
- De Frenne P, Rodríguez-Sánchez F, Coomes DA, Baeten L, Verstraeten G, Vellend M, Bernhardt-Römermann M, Brown CD, Brunet J, Cornells J, et al. 2013. Microclimate moderates plant responses to macroclimate warming. Proc Natl Acad Sci. 110(46):18561–18565. doi: 10.1073/pnas.1311190110.
- De Frenne P, Roger J, Lenoir MH, Luoto M, Scheffers B, Zellweger F, Aalto J, Ashcroft MB, Christiansen DM, Decocq G, et al. 2021. Forest microclimates and climate change: importance, drivers and future research agenda. Glob Chang Biol. 27(11):2279–2297. doi: 10.1111/gcb.15569ff.ffhal-03293490.
- Doi R, Tanikawa T, Wada R, Hirano Y. 2020. Morphological traits of Chamaecyparis obtusa fine roots are sensitive to soil acid buffering capacity. Plant Soil. 452(1–2):73–85. doi: 10.1007/s11104-020-04561-w.
- Domisch T, Finér L, Lehto T. 2001. Effects of soil temperature on biomass and carbohydrate allocation in scots pine (Pinus sylvestris) seedlings at the beginning of the growing season. Tree Physiol. 21(7):465–472. doi: 10.1093/treephys/21.7.465.
- Domisch T, Martz F, Repo T, Rautio P. 2017. Winter survival of scots pine seedlings under different snow conditions. Tree Physiol. 38(4):602–616. doi: 10.1093/treephys/tpx111.
- Domisch T, Qian J, Sondej I, Martz F, Lehto T, Piirainen S, Finér L, Silvennoinen R, Repo T. 2020. Here comes the flood! Stress effects of continuous and interval waterlogging periods during the growing season on Scots pine saplings. Tree Physiol. 40:869–885. doi: 10.1093/treephys/tpaa036.
- Fan Y, Miguez-Macho G, Jobbágy EG, Jackson RB, Otero-Casal C. 2017. Hydrologic regulation of plant rooting depth. Proc Natl Acad Sci. 114(40):10572–10577. doi: 10.1073/pnas.1712381114.
- FAO. 2020. Global forest resources assessment 2020: main report. Rome. 10.4060/ca9825en.
- Finér L, Ohashi M, Noguchi K, Hirano Y. 2011. Fine root production and turnover in forest ecosystems in relation to stand and environmental characteristics. For Ecol Manage. 262(11):2008–2023. doi: 10.1016/j.foreco.2011.08.042.
- Forest Europe. 2020. State of European forests 2020. p. 394. SoEF_2020.pdf (foresteurope.org).
- Freschet GT, Pagès L, Iversen C, Comas L, Rewald B, Roumet C, Klimesova J, Zadworny M, Poorter H, Postma JA. 2021. A starting guide to root ecology strengthening ecological concepts and standardizing root classification, sampling, processing and trait measurements. New Phytol. 232(3):973–1122. doi: 10.1111/nph.17572.
- Freschet GT, Roumet C, Comas LH, Weemstra M, Bengough AG, Rewald B, Bardgett RD, de Deyn GB, Johnson D, Klimesova J, et al. 2021. Root traits as drivers of plant and ecosystem functioning: current understanding, pitfalls and future research needs. New Phytol. 232(3):1123–1158. doi: 10.1111/nph.17072.
- Fujita S, Noguchi K, Tange T. 2021. Different waterlogging depths affect spatial distribution of fine root growth for Pinus thunbergii seedlings. Front Plant Sci. 12:614–764. doi: 10.3389/fpls.2021.614764.
- Germon A, Laclau J-P, Robin A, Jourdan C. 2020. Tamm Review: deep fine roots in forest ecosystems: why dig deeper? For Ecol Manage. 466:118135. doi: 10.1016/j.foreco.2020.118135.
- Glenz C, Schlaepfer R, Iorgulescu I, Kienast F. 2006. Flooding tolerance of central European tree and shrub species. Rev For Ecol Manage. 235(1–3):1–13. doi: 10.1016/j.foreco.2006.05.065.
- Gottfried M, Pauli H, Futschik A, Akhalkatsi M, Barabcok P, Benito Alonso JL, Goldea G, Dick J, Erschbaumer B, Fernández Calzado MR. 2012. Continent-wide response of mountain vegetation to climate change. Nat Clim Chang. 2(2):111–115. doi: 10.1038/nclimate1329.
- Groffman PM, Driscoll CT, Fahey TJ, Hardy JP, Fitzhugh RD, Tierney GL. 2001. Colder soils in a warmer world: a snow manipulation study in a northern hardwood forest ecosystem. Biogeochemistry. 56:135–150. doi: 10.1023/A:1013039830323.
- Grossiord C. 2019. Having the right neighbors: how tree species diversity modulates drought impacts on forests. New Phytol. 228(1):42–49. doi: 10.1111/nph.15667.
- Grossiord C, Granier A, Ratcliffe S, Bouriaud O, Bruelheide H, Chećko E, Forresterg DI, Dawud SM, Finér L, Pollastrini M. 2014. Tree diversity does not always improve resistance of forest ecosystems to drought. Proc Natl Acad Sci. 111(41):14812–14815. doi: 10.1073/pnas.1411970111.
- Helama L, Tuomenvirta H, Venäläinen A. 2011. Boreal and subarctic soils under climatic change. Glob Planet Chang. 79(1–2):37–47. doi: 10.1016/j.gloplacha.2011.08.001.
- Hereford J. 2009. A quantitative survey of local adaptation and fitness trade-offs. Am Nat. 173(5):579–588. doi: 10.1086/597611.
- Hesslerová P, Pokorný J, Brom J, Rejšková–Procházková A. 2013. Daily dynamics of radiation surface temperature of different land cover types in a temperate cultural landscape: consequences for the local climate. Ecol Eng. 54:145–154. doi: 10.1016/j.ecoleng.2013.01.036.
- Huuskonen S, Domisch T, Finér L, Hantula J, Hynynen J, Matala M, Miina J, Neuvonen S, Nevalainen S, Niemistö P. 2021. What is the potential for replacing monocultures with mixed-species stands to enhance ecosystem services in boreal forests in Fennoscandia? For Ecol Manage. 479:118558. doi: 10.1016/j.foreco.2020.118558.
- Creed IF, van Noordwijk M, editors. 2018. Forest and water on a changing planet – vulnerability, adaptation and governance opportunities. A global assessment report. In: IUFRO world series Vol. 38, Vienna: International Union of Forest Research Organizations (IUFRO); p. 192.
- IPCC. 2022 Pörtner H-O, Roberts D, Tignor M, Poloczanska E, Mintenbec K, Alegrí A, Craig M, Langsdorf S, Löschke S, Möller V, editors. Climate change 2022: impacts, adaptation and vulnerability. Contribution of working group II to the sixth assessment report of the intergovernmental panel on climate change. Cambridge, (UK) and New York (NY) (USA): Cambridge University Press. doi: 10.1017/9781009325844.
- Iversen CM. 2010. Digging deeper: fine-root responses to rising atmospheric CO 2 concentration in forested ecosystems. New Phytol. 186(2):346–357. doi: 10.1111/j.1469-8137.2009.03122.x.
- Jackson RB, Canadell J, Ehleringer JR, Mooney H, Sala OE, Schulze ED. 1996. A global analysis of root distributions for terrestrial biomes. Oecologia. 108(3):389–411. doi: 10.1007/BF00333714.
- Jones MD, Durall DM, Cairney JWG. 2003. Ectomycorrhizal fungal communities in young forest stand regenerating after clearcut logging. New Phytol. 157(3):399–422. doi: 10.1046/j.1469-8137.2003.00698.x.
- Kankare V, Luoma V, Saarinen N, Peuhkurinen J, Holopainen M, Vastaranta M. 2019. Assessing feasibility of the forest trafficability map for avoiding rutting – a case study. Silva Fenn. 53(3):10197. doi: 10.14214/sf.10197.
- Keenan RJ. 2015. Climate change impacts and adaptation in forest management: a review. Ann For Sci. 72(2):145–167. doi: 10.1007/s13595-014-0446-5.
- Kolström M, Lindner M, Vilén T, Maroschek M, Seidl R, Lexer MJ, Netherer S, Kremer A, Delzon S, Barbati A, et al. 2011. Reviewing the science and implementation of climate change adaptation measures in European forestry. Forests. 2(4):961–982. doi: 10.3390/f2040961.
- Kranabetter J, Kroeger P. 2001. Ectomycorrhizal mushroom response to partial cutting in a western hemlock - western redcedar forest. Can J For Res. 31(6):978–987. doi: 10.1139/cjfr-31-6-978.Kubin.
- Kubin E, Kemppainen L. 1994. Effects of soil preparation of boreal spruce forest on air and soil temperature conditions in forest regeneration areas. Acta For Fenn. 244:56. http://hdl.handle.net/10138/27260.
- Latterini F, Dyderski MK, Horodecki P, Rawlik M, Stefanoni W, Högbom L, Venanzi R, Picchio R, Jagodzin´ki AM. 2023. A meta-analysis of the effects of ground-based extraction technologies on fine root in forests. Land Degrad Dev. 35(1):9. doi: 10.1002/ldr.4902.
- Leimu R, Fischer M, Buckling A. 2008. A meta-analysis of local adaptation in plants. PLOS ONE. 3(12):e4010. doi: 10.1371/journal.pone.0004010.
- Leites L, Benito Garzón M. 2023. Forest tree species adaptation to climate across biomes: building on the legacy of ecological genetics to anticipate responses to climate change. Glob Chang Biol. 29(17):4711–4730. doi: 10.1111/gcb.16711.
- Lenoir J, Svenning JC. 2015. Climate-related range shifts – a global multidimensional synthesis and new research directions. Ecography. 38(1):15–28. doi: 10.1111/ecog.00967.
- Leppä K, Hökkä H, Laiho R, Launiainen S, Lehtonen A, Mäkipää R, Peltoniemi M, Saarinen M, Sarkkola S, Nieminen M. 2020. Selection cuttings as a tool to control water table level in boreal drained peatland forests. Front Earth Sci. 8:576510. doi: 10.3389/feart.2020.576510.
- Leppälammi-Kujansuu J, Salemaa M, Kleja DB, Linder S, Helmisaari H–S. 2014. Fine root turnover and litter production of Norway spruce in a long-term temperature and nutrient manipulation experiment. Plant Soil. 374(1–2):73–88. doi: 10.1007/s11104-013-1853-3.
- Liu X, Biondi F. 2021. Inter-specific transpiration differences between aspen, spruce, and pine in a sky-island ecosystem of the North American Great Basin. For Ecol Manage. 491:119157. doi: 10.1016/j.foreco.2021.119157.
- Lyr H, Hoffmann G. 1967. Growth rates and growth periodicity of tree roots. In: Romberger J Mikola P, editors. Int Rev For Res Vol. 2, 181–236. 10.1016/B978-1-4831-9976-4.50011-X.
- Malcolm JR, Markham A, Neilson RP, Garaci M. 2002. Estimated migration rates under scenarios of global climate change. J Biogeogr. 29(7):835–849. doi: 10.1046/j.1365-2699.2002.00702.x.
- Mariotti B, Hoshika Y, Cambi M, Marra E, Feng Z, Paoletti E, Marchi E. 2020. Vehicle-induced compaction of forest soil affects plant morphological and physiological attributes: a meta-analysis. For Ecol Manage. 462:118004. doi: 10.1016/j.foreco.2020.118004.
- Masuda C, Kanno H, Masaka K, Morikawa Y, Suzuki M, Tada C, Hayashi S, Seiwa K. 2022. Hardwood mixtures facilitate leaf litter decomposition and soil nitrogen mineralization in conifer plantations. For Ecol Manage. 507:120006. doi: 10.1016/j.foreco.2021.120006.
- McCormack ML, Dickie IA, Eissenstat DM, Fahey TJ, Fernandez CW, Guo DL, Helmisaari H–S, Hobbie EA, Iversen CM, Jackson RB, et al. 2015. Redefining fine roots improves understanding of below-ground contributions to terrestrial biosphere processes. New Phytol. 207:505–518. doi: 10.1111/nph.13363.
- Nardini A, Casolo V, Dal Borgo A, Savi T, Stenni B, Bertoncin P, Zini L, McDowell NG. 2016. Rooting depth, water relations and non-structural carbohydrate dynamics in three woody angiosperms differently affected by an extreme summer drought. Plant Cell Environ. 39(3):618–627. doi: 10.1111/pce.12646.
- Nazari M, Arthur E, Lamandé M, Keller M, Bilyera N, Bickel S. 2023. A metaanalysis of soil susceptibility to MachineryInduced compaction in forest ecosystems across global climatic zones. Curr For Rep. 9(5):370–381. doi: 10.1007/s40725-023-00197-y.
- Nazari M, Eteghadipour M, Zarebanadkouki M, Ghorbani M, Dippold MA, Bilyera N, Zamanian K. 2021. Impacts of logging-associated compaction on forest soils: a meta-analysis. Front For Glob Change. 4:780074. doi: 10.3389/ffgc.2021.780074.
- Niinemets Ü, Valladares F. 2006. Tolerance to shade, drought, and waterlogging of temperate northern hemisphere trees and shrubs. Ecol Monogr. 76(4):521–547. doi: 10.1890/0012-9615(2006)076[0521:TTSDAW]2.0.CO;2.
- Noguchi K, Matsuura Y, Sparrow S, Hinzman LD. 2016. Fine root biomass in two black spruce stands in interior Alaska: effects of different permafrost conditions. Trees. 30(2):441–449. doi: 10.1007/s00468-015-1226-z.
- Päivänen J, Hånell B. 2012. Peatland ecology and forestry – a sound approach. 267. University of Helsinki Department of Forest Science Publications 3.
- Pan Y, Birdsey RA, Fang J, Houghton R, Kauppi PE, Kurz WA, Phillips OL, Shvidenko A, Lewis SL, Canadell JG, et al. 2011. Aug 19. A large and persistent carbon sink in the world’s forests. Science. 333(6045):988–993. doi: 10.1126/science.1201609.
- Pardos M, Del Río M, Pretzsch H, Jactel H, Bielak K, Bravo F, Brazaitis G, Defossez E, Engel M, Godvod K, et al. 2021. The greater resilience of mixed forests to drought mainly depends on their composition: analysis along a climate gradient across Europe. For Ecol Manage. 481:118687. doi: 10.1016/j.foreco.2020.118687.
- Pregitzer KS, King JS, Burton AJ, Brown SE. 2000. Responses of tree fine roots to temperature. Res Rev New Phytol. 147(1):105–115. doi: 10.1046/j.1469-8137.2000.00689.x.
- Pretzch H. 2005. Diversity and productivity in forests: evidence from long-term experimental plots. In: Scherer-Lorenze M, Körner C Schulze E-D, editors. Forest diversity and function: temperate and boreal systems. Ecological studies Vol. 176, Berlin Heidelberg: Springer-Verlag. doi: 10.1007/3-540-26599-6_3.
- Repo T, Domisch T, Kilpeläinen J, Piirainen S, Silvennoinen R, Lehto T. 2020. Dynamics of fine-root production and mortality of scots pine in waterlogged peat soil during the growing season. Can J For Res. 50(5):510–518. doi: 10.1139/cjfr-2019-0163.
- Repo T, Launiainen S, Lehto T, Sutinen S, Ruhanen H, Heiskanen J, Laurén A, Silvennoinen R, Vapaavuori E, Finér L. 2016. The responses of scots pine seedlings to waterlogging during the growing season. Can J For Res. 46(12):1439–1450. doi: 10.1139/cjfr-2015-0447.
- Repo T, Sirkiä S, Heinonen J, Lavigné A, Roitto M, Koljonen E, Sutinen S, Finér L. 2014. Effects of frozen soil on growth and longevity of fine roots of Norway spruce. For Ecol Manage. 313:112–122. doi: 10.1016/j.foreco.2013.11.002.
- Roitto M, Sutinen S, Wang A, Domisch T, Lehto T, Repo T. 2019. Waterlogging and soil freezing during dormancy affected root and shoot phenology and growth of scots pine saplings. Tree Physiol. 39(5):805–818. doi: 10.1093/treephys/tpz003.
- Royo AA, Raymond P, Kern CC, Adams BT, Bronson D, Champagne E, Dumais D, Gustafson E, Marquardt PE, McGraw AM, et al. 2023. Desired regeneration through assisted migration (DREAM): implementing a research framework for climate-adaptive silviculture. For Ecol Manage. 546:121298. doi: 10.1016/j.foreco.2023.121298.
- Ryyppö A, Repo T, Vapaavuori E. 1998. Development of freezing tolerance in roots and shoots of scots pine seedlings at nonfreezing temperatures. Can J For Res. 28(4):557–565. doi: 10.1139/x98-022.
- Salmivaara A, Launiainen S, Perttunen J, Nevalainen P, Pohjankukka J, Ala-Ilomäki J, Sirén M, Laurén A, Tuominen S, Uusitalo J, et al. 2020. Towards dynamic forest trafficability prediction using open spatial data, hydrological modelling and sensor technology. Forestry. 13(5):662–674. doi: 10.1093/forestry/cpaa010.
- Salmivaara A, Launiainen S, Tuominen S, Ala-Ilomäki J, Finér L. 2017. Topographic wetness index for Finland 2016 natural resources institute Finland, Etsin research data finder, CSC (distributor). [accessed 2024 Jan]. http://urn.fi/urn:nbn:fi:csc-kata20170511113233803176.
- Savolainen O, Pyhäjärvi T, Knürr T. 2007. Gene flow and local adaptation in trees. Annu Rev Ecol Evol Syst. 38(1):595–619. doi: 10.1146/annurev.ecolsys.38.091206.095646.
- Schenk HJ, Jackson RB. 2002. The global biogeography of roots. Ecol Monogr. 72(3):311–328. doi: 10.1890/0012-9615(2002)072[0311:TGBOR]2.0.CO;2.
- Schwarz M, Lehmann P, Or D. 2010. Quantifying lateral root reinforcement in steep slopes – from a bundle of roots to tree stands. Earth Surf Process Landf. 35(3):354–367. doi: 10.1002/esp.1927.
- Seiwa K, Negishi Y, Eto Y, Hishita M, Masaka K, Fukasawa Y, Matsukura K, Suzuki M. 2020. Successful seedling establishment of arbuscular mycorrhizal-compared to ectomycorrhizal-associated hardwoods in arbuscular cedar plantations. For Ecol Manage. 468:118155. doi: 10.1016/j.foreco.2020.118155.
- Sikström U, Hökkä H. 2016. Interactions between soil water conditions and forest stands in boreal forests with implications for ditch network maintenance. Silva Fenn. 50(1):1416. doi: 10.14214/sf.1416.
- Sohn JA, Saha S, Bauhus J. 2016. Potential of forest thinning to mitigate drought stress: a meta-analysis. For Ecol Manage. 380:261–273. doi: 10.1016/j.foreco.2016.07.046.
- Sousa‐Silva R, Verheyen K, Ponett Q, Bay E, Sioen G, Titeux H, Van de Peer T, Van Meerbeek K, Muys B. 2018. Tree diversity mitigates defoliation after a drought‐induced tipping point. Glob Chang Biol. 24(9):4304–4315. doi: 10.1111/gcb.14326.
- Tenkanen A, Suprun S, Oksanen E, Keinänen M, Keski-Saari S, Kontunen-Soppela S. 2021. Strategy by latitude? Higher photosynthetic capacity and root mass fraction in northern than southern silver birch (Betula pendula Roth) in uniform growing conditions. Tree Physiol. 41(6):974–991. doi: 10.1093/treephys/tpaa148.
- Vapaavuori EM, Rikala R, Ryyppö A. 1992. Effects of root temperature on growth and photosynthesis in conifer seedlings during shoot elongation. Tree Physiol. 10(3):217–230. doi: 10.1093/treephys/10.3.217.
- Verstraeten WW, Muys B, Feyen J, Veroustraete F, Minnaert M, Meiresonne L, de Schrijve A. 2005. Comparative analysis of the actual evapotranspiration of Flemish forest and cropland, using the soil water balance model WAVE. Hydrol Earth Syst Sci. 9(3):225–241. doi: 10.5194/hess-9-225-2005.
- Wang J, de Frenne C, McCormack M, Yang L, Tian D, Luo Y, Hou E, Yan T, Li Z, Bu W, et al. 2021. Fine-root functional trait responses to experimental warming: a global meta-analysis. New Phytol. 230(5):1856–1867. doi: 10.1111/nph.17279.
- Weemstra M, Kiorapostolou N, van Ruijven J, Mommer L, de Vries J, Sterck F. 2020. The role of fine-root mass, specific root length and life span in tree performance: a whole-tree exploration. Func Ecol. 34(3):575–585. doi: 10.1111/1365-2435.13520.
- Wu D, Pulkkinen P, Pappinen A, Neyko I, Zhang G, Di B, Heinonen J, Repo T. 2023. Frost hardiness of Finnish plus tree progenies of scots pine from seed orchards in Finland and Ukraine. Eur J For Res. 142(6):1467–1477. doi: 10.1007/s10342-023-01606-4.
- Wu Q-S, He J-D, Srivastava AK, Zou YN, Kuča K. 2019. Mycorrhizas enhance drought tolerance of citrus by altering root fatty acid compositions and their saturation levels. Tree Physiol. 39(7):1149–1158. doi: 10.1093/treephys/tpz039.
- Zadworny M, McCormack ML, Mucha J, Reich BP, Oleksyn J. 2016. Scots pine fine roots adjust along a 2000-km latitudinal climatic gradient. New Phytol. 212(2):389–399. doi: 10.1111/nph.14048.
- Zellweger F, de Frenne P, Lenoir J, Vangansbeke P, Verheyen K, Bernhardt-Römermann M, Baeten L, Hédl R, Berki I, Brunet J. 2020. Forest microclimate dynamics drive plant responses to warming. Science. 368(6492):772–775. doi: 10.1126/science.aba6880ff.ffhal-02998429.