Abstract
Tropical areas exhibit torrential rains, and when the rate of soil drainage is slower than the rate of precipitation, rapid flooding can result which can lead to germination failure. The objective of this study was to evaluate the tolerance of three bean cultivars (IPR-139, Pérola and IPR-Tiziu) to submersion in water for different time periods (0, 1, 2, 4, 8 and 16 h). Seeds were evaluated for moisture content, electrical conductivity, leached ethanol, germination percentage and vigour classification, length and dry weight of roots and shoots, proline content and superoxide dismutase (SOD) activity (an antioxidant enzyme). Increasing the submersion time resulted in decreased germination, vigour and growth, with increased electrical conductivity and leaching of ethanol. Just 1 h of submersion was enough for early differences to be evident among cultivars. The SOD activity was near constant during submersion in all cultivars. Overall, IPR-139 was the most susceptible to submersion, and IPR-Tiziu was the most tolerant cultivar. The tolerance of IRP-Tiziu was related to the high proline content in dry seeds and proline consumption during the submersion period.
Brazil is a continental country with a highly diverse climate. In the summer, heavy rainfall may occur over a large part of the Brazilian territory, and under some situations, crops may suffer from short periods of flooding during seed sowing to seedling emergence. Flooding is characterised by oxygen deprivation, even in soils without drainage problems, resulting in a limited supply of oxygen for roots, seeds, micro-organisms and chemical processes, leading to a less efficient metabolic pathway (anaerobiosis) and to a rapid depletion of carbohydrate reserves (Banach et al., Citation2009; Bailey-Serres & Voesenek, Citation2008).
Although most terrestrial plants can tolerate light flooding, there are a small number of species which can grow and reproduce under severe flooding; for example, some tropical plants or those which occupy coastal marshes and rice. Other crops such as wheat exhibit modest tolerance to occasional flooding (Jackson et al., Citation2009). However, the flooding tolerance of the crop Phaseolus, of which there are over four species in agricultural production, has not been well studied except for some research that has been conducted on the seeds of Phaseolus vulgaris (Custódio et al., Citation2002, 2009).
Many seeds experience anaerobic conditions during phase II of water uptake (i.e. following imbibition but preceding germination) which is characterised by the accumulation of ethanol and lactic acid (i.e. the anaerobic pathway products). When tissue disruption occurs due to radicle elongation, these fermentation products are degraded by alcohol and lactate dehydrogenase as conditions become more aerobic. These enzymes are frequently present in dry seeds but their activity increases by 10-fold or more in germinating seeds (Bewley et al., Citation2013). When germination is completed or when conditions become more aerobic, the enzymes activities and their products are negligible. This natural period of anaerobiosis can extend from a few hours to several days and may be prolonged by immersing seeds in water, which is characterised by the leaching of ethanol into the surrounding environment (Bewley et al., Citation2013).
Under anaerobic conditions, the primary products formed in seedlings are ethanol and two enzymes, alcohol dehydrogenase (ADH, EC 1.1.1.1) and pyruvate decarboxylase (PDC; EC 4.1.1.1), which are involved in the biosynthesis of ethanol (Bewley et al., Citation2013). A metabolic adaptation to flooding may also include the production of enzymes of the lactic and alanine fermentation pathways (Parent et al., Citation2008). Furthermore, in lettuce seedlings, anoxia-induced protein synthesis, decreased ADH activity and decreased availability of ATP were associated with low flooding tolerance (Kato-Noguchi, Citation2001).
Another consequence of anaerobiosis is the production of reactive oxygen species (ROS; for review see Bailey-Serres et al., 2012). Hypoxia over a period of eight days increased the activities of the ROS-scavenging enzymes superoxide dismutase (SOD), ascorbate peroxidase (APX) and glutathione reductase (GSR) activities in tolerant genotypes of 25-day-old Vigna, suggesting the removal of excess ROS associated with flooding stress (Sairam et al., Citation2011). Furthermore, the ROS-scavenger enzymes glutathione-S-transferase (GST), cytosolic APX and a putative GST were inhibited in rice coleoptiles under anaerobic conditions (Sadiq et al., Citation2011). Similar results have been reported with APX for soybean under hypoxic conditions (Hashiguchi et al., Citation2009; Shi et al., Citation2008). However, using a membrane marker of peroxidative damage by ROS, rice seedlings were examined during submersion in water, in the dark, to check whether the damage was a phenomenon that occurred after submersion or when seedlings were once again in contact with oxygen. Damage occurred during submersion and was not associated with prolonged anoxia. In seedlings that were partially deprived of oxygen and then placed into contact with oxygen again, peroxidation was suppressed as an effective control of the ROS production which was not evident during submersion (Santosa et al., Citation2007).
Proline is a multifunctional amino acid that is commonly involved in stress responses and has been found to have antioxidant activity in addition to being a source of energy or carbon backbones and an important osmolyte (Szabados & Savoure, Citation2010). In Aloe vera L. plants growing in soil with a nutritive solution for 60 days, the formation of aerenchyma (characterised by programmed cell death) and the simultaneous proline accumulation (Verbruggen & Hermans, Citation2008; He et al., Citation2012) occurred in response to the excess of water.
The limited studies on Vigna and Phaseolus have revealed that these genera do not tolerate flooding in the early developmental stages, but differences between cultivars have been observed (Singh & Singh, Citation2011). The Cajanus genus has shown high susceptibility to flooding during germination and in the early and later seedling developmental stages, and this information is used to select tolerant genotypes (Choudhary et al., Citation2011). There are also varietal differences in seed survival after flooding (Saka & Izawa, Citation1999; Lenssen et al., Citation1999), and it is possible to discriminate among batches of the same variety because more vigorous seeds are more tolerant to submersion conditions (Custódio et al., Citation2002, 2009).
Considering: (i) the importance of common bean as a staple food in Brazil and the susceptibility of this crop to flooding, especially in the early growth stages, (ii) that bean cultivars may differ in proline concentration, even in dry seeds produced under the same conditions (Machado-Neto et al., Citation2004) and (iii) the important role of proline as an energy source (Szabados & Savoure, Citation2010), it can be hypothesised that seeds with a high proline content may respond differently to low oxygen during germination under flooding than seeds with a lower proline content. The objective of this study was to evaluate the seed tolerance of bean cultivars to submersion in water for different periods of time.
Material and methods
Seed material
Seeds from the common bean (Phaseolus vulgaris L.) from two different colour groups were used in this study. Two were from the carioca (IPR-139 and Pérola) and one was from the black group (IPR-Tiziu). These cultivars were chosen because they are modern cultivars, seeds were freely available and they have different proline contents in the dry seed. After assessing three batches of each cultivar for germination and vigour, one of each of the following was selected: IPR-139 (from a seed producer), Pérola (from DSMM - CATI – Avaré/SP) and IPR-Tiziu (from the company Selegrãos – Santo Anastácio/SP). These batches exhibited the same pattern of initial germination, which allowed for comparison among cultivars and water submersion periods.
Seeds were stored in a climate-controlled room at 20 ± 3 °C and 60% relative humidity until the tests were initiated. Evaluations for initial quality establishment were moisture content determination, germination test, classification of seedling vigour, length and dry mass of shoots and roots, electrical conductivity after 24 h of submersion and proline content of dry seeds.
Submersion
Seed submersion in water, with no gas control, was conducted over periods of 1, 2, 4, 8 and 16 h (the control was not submerged) with 10 groups of 50 seeds per time period and per cultivar, at 25 °C in a growing chamber in the dark. These treatments were carried out before germination. Seeds were weighed before being submerged in a 5-cm water column (75 mL). After each time period, the electrical conductivity was measured, the water was drained, and seeds evaluated for moisture content (two replicates of 50 seeds), and germination test, vigour classification, length and dry weight of shoot and root, in four replicates of 50 seeds. One group of 50 seeds was used to determine proline content and SOD activity in three replicates. The same number of seeds and replicates were used for the determination of ethanol in water and in the tissues. Both the imbibition solution and drained seeds were conditioned in polypropylene tubes and stored at –20 °C. The submersion treatment was conducted twice to confirm repeatability.
Moisture content determination
This was conducted gravimetrically using two replicates of 50 seeds per cultivar in an oven without air movement at 105 ± 3 °C for 24 h (Brasil, Citation2009).
Electrical conductivity
Conductivity was determined using a meter (HI 2300, Hanna Instruments, USA). This was achieved using four replicates of 50 seeds per cultivar that were weighed and placed in plastic containers with a volume of 150 mL. The containers were filled with 75 mL of deionised water and kept in an incubator at 25 °C during the studied submersion periods and for the initial evaluation after 24 h of submersion. A plastic container that was filled only with water was also evaluated as the control. After the submersion periods, the electrical conductivity of the solution was determined and was subtracted from the water conductivity and divided by the sample weight. The results are expressed as μS cm−1 g−1 of seed fresh weight (Vieira & Krzyzanowski, Citation1999).
Ethanol determination
The method used was gas chromatography (GC, VARIAN 3800) with a flame ionisation detector equipped and a headspace (Markelov, HS 9000) using a Carbowax 20 M column (30 m length, .25 mm internal diameter I.D. with .25-μm film thickness). The temperature of the column and injector was 120 °C and 230 °C, respectively. Nitrogen was used as a carrier gas in a flux of 25 mL min−1. The samples were prepared in 20-mL flasks containing n-propanol (16 g L−1) as an internal standard and 1 mL of the sample. The vials were sealed and kept in an auto-sampler for the alcoholic components volatilisation at 80 °C and 200μL of the gas were injected in the GC and the run proceeded in 2 min. Five seeds were ground in 10 mL of deionised water for each replicate. One mL per repetition was used, and the results are expressed as mg g−1 of dried seed.
Germination test and vigour classification
These tests were conducted using four replicates of 50 seeds, which were sown in paper towel rolls embedded with distilled water (2.5 times the weight of the paper) and placed in an incubator at 25 °C (24 h dark). Control seeds (not submerged for any time) and treated seeds (seeds that were submerged for different times) were germinated in the same way. Germination counts were performed at five and seven days after sowing. On the seventh day, seedlings were classified as strong (morphologically perfect) and normal (Brasil, Citation2009), abnormal and weak (with minor defects) or dead. Germination was measured as the percentage of normal and abnormal seedlings relative to the number of seeds tested. For vigour classification, only the percentage of normal seedlings was considered (Nakagawa, Citation1999).
Seedling length and dry weight measurements
Four replicates of 10 seeds were germinated as in the germination test to assess shoot, root, total seedling length and dry masses. After seven days, the seedlings were measured, divided into roots and shoots (without cotyledons) and dried at 60 °C for 72 h for the determination of dry mass (Nakagawa, Citation1999). The total dry mass was calculated as the sum of the dry mass of shoot and root.
Proline content
Three replicates of 800 mg of seeds were analysed following the method of Machado-Neto et al. (Citation2004) using a spectrophotometer at a wavelength of 520 nm. The proline content was plotted against a calibration curve and expressed as μg g−1 of dried tissue.
Superoxide dismutase activity (SOD, EC.1.15.11)
One gram of seeds was weighed and ground in liquid nitrogen and then homogenised in 10 ml of 100 mM phosphate buffer (pH 7.8, 4 °C), containing .4% polyvinylpolypyrrolidone, 2 mM dithiothreitol, .1 mM ethylenediaminetetraacetic acid (EDTA) and centrifuged at 12,000 g for 20 min. The supernatant was collected and stored at –80 °C until further use. SOD activity was evaluated as described by Moriya et al. (Citation2015), by adding 100 mM phosphate buffer (pH 7.8) to 50 μL of extract containing 13 mM methionine and 63 μM nitro blue tetrazolium (NBT), with 13 μM riboflavin as a modification. The tubes were incubated at 25 °C for 15 min under fluorescent lamps, after which the absorbance was measured at 560 nm. Tubes containing the same medium but not subjected to light were used as a control. One unit of SOD (mg protein)−1 was defined as the enzyme activity that is able to inhibit the photoreduction of NBT to blue formazan by 50%. SOD data were normalised by the protein content, following the method of Bradford (Citation1976)..
Statistical design
The experiment was conducted using a completely randomised statistical design with four replicates per treatment. The treatments were arranged in a (3 × 6) factorial design that was composed of three cultivars (IPR-139, Pérola and IPR-Tiziu) and six submersion periods. The percentage results were transformed using arcsine (x.100−1)−1/2. The data were analysed using an ANOVA F test (p < .05), and the means were compared using Tukey’s test (p < .05). Polynomial regressions were used for the quantitative data. The significant mathematical models with the highest determination coefficient (R2) were considered. Data were analysed using SISVAR software (Ferreira, Citation2011). Moisture content was not statistically analysed because these data followed the tolerance level for established differences between samples (Brasil, Citation2009). Ethanol determination, proline content and SOD activity were analysed using the mean ± standard deviation.
Results and discussion
Initial vigour traits before water submersion
Tolerance to water submersion may be influenced by seed vigour (Custódio et al., Citation2002, 2009), and even small differences in seed vigour may translate into larger differences in seedling performance (Marcos Filho, Citation2005). Therefore, it is important to verify varietal differences and to use seeds with the same initial quality, as was the case here. The initial evaluation of each cultivar batch (Table ) showed equal results for germination (G%), length and root dry mass (RDM). Seedling vigour classification (CV) showed that Pérola cultivar was inferior to IPR-39 and IPR-Tiziu; however, length, shoot and total dry mass showed the inverse trend.
Table 1. Germination (G), Vigour Classification (VC), Proline, Root length (RL), Shoot length (SL), Total length (TL), Root dry mass (RDM), shoot dry mass (SDM), total dry mass (TDM), electrical conductivity (CE) and moisture content (MC) from the initial evaluation of bean seed from the cultivars IPR-139, Pérola and IPR-Tiziu.
Seeds of IPR-139, Pérola and IPR-Tiziu had initial moisture contents of 12.2, 9.5 and 12.4%, respectively (Table ). The drier the seed, the more likely imbibition is to be damaging which can result in a higher leaching of electrolytes. Although there were differences in the initial moisture content of the seeds of IPR-139 and Pérola cultivars, both showed higher electrolyte leakage in the electric conductivity test (Table ).
Seed–water relations and electrolyte leakage during submersion
After each submersion period, the determination of the seed moisture content showed that all three cultivars rapidly imbibed water during the first four hours, followed by a slow rate of water uptake. After four hours submersion, IPR-139, Pérola and IPR-Tiziu had 52, 47 and 49% seed moisture content, respectively. Pérola was the slowest cultivar to imbibe water. There was no evidence of the primary root during the submersion periods, showing that there was no progression of visible germination, i.e. elongation and cell division in the radicle as a function of the anaerobic process caused by the submersion. After 16 h of submersion, all cultivars had seed moisture contents of between 56 and 57% (Figure (A)).
Figure 1. Moisture content (A), electrical conductivity of imbibition water (B) and ethanol determination in imbibition water (C) of three bean cultivars seeds submitted to different submersion period. (B) Adjusted equations: y (IPR-139) = R² = .8877**; y (Pérola) =
R² = .8937**; y (IPR-Tiziu) =
R² = .9201**. Significant equation: *(p < .05); **(p < .01). In the same period of submersion equal letters are not statistically different by the Tukey’s test at .05 of probability; the absence of a letter indicates no significance. (C) Error bars are the standard deviation.
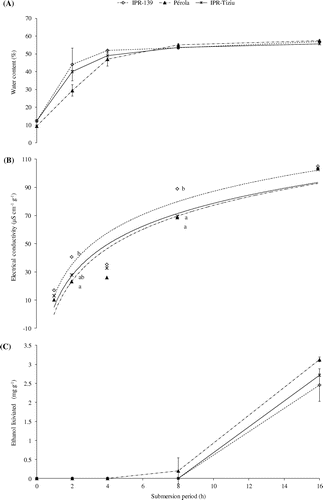
Water is essential for cell metabolism during germination because of its role in enzymatic activity, for the solubilisation and transport of reserves and as a reagent itself. At the start of imbibition, the movement of water into the seed occurs down a water potential gradient (Bewley et al., Citation2013). The different rates of hydration of the seeds may be attributed to variations in the coat permeability, as bright and dark seeds have lower rates of hydration. In this study, the dark-coated seeds of the IPR-Tziu cultivar were not the slowest to imbibe water (Figure (A)). Another factor that influences the rate of hydration is the seed chemical composition; the higher the concentration of protein and sugar contents (hydrophilic substances), the faster the seed will absorb water (Marcos Filho, Citation2005). This may explain the water uptake of IPR-Tziu which had the highest concentration of proline of the three cultivars.
The electrical conductivity, which was evaluated after each submersion period, showed a logarithmic increase and was most rapid within the initial four hours. No statistical differences were observed between the cultivars at one, four and 16 h of submersion. IPR-139 exhibited the highest conductivity and differed from Pérola by two hours of submersion; by eight hours IPR-139 maintained the highest conductivity (Figure (B)). Santosa et al. (Citation2007) concluded that a lack of oxygen, resulting from submersion, causes peroxidative damage to the cell membranes and this can occur after only a short exposure to a lack of oxygen. Here, two hours was sufficient to see differences between the cultivars and prolonged submersion resulted in increased electrolyte leakage. The elevated conductivity may also be attributed to the condition of the cellular membranes and the capability to reorganise the membranes as imbibition progresses (Marcos Filho, Citation2005). In this case, the cultivar with a low initial moisture content did not exhibit the highest leaching, indicating that the initial differences in water content (Table ) were not related to higher electrolyte leakage during submersion (Figure (B)).
Seed and seedling vigour traits during submersion
After just one hour of submersion, the germination of IPR-139 decreased but there was no change in germination of IPR-Tiziu and Pérola during the first four hours of submersion. By 8 and 16 h of submersion, IPR-Tiziu exhibited higher germinability than the other cultivars (Table ) but this was not related to a lower electrical conductivity (Figure (B)). The higher performance of IPR-Tiziu after long-term submersion was also evident in the vigour classification (Table ), with fewer plants damaged by eight and 16 h submersion conditions (Table ). Also reflecting the germination behaviour, Pérola had a higher vigour classification during shorter term submersion (first four hours). Overall, the vigour classification showed a linear decrease of 3.64, 4.49 and 3.44%, based on the adjusted equations based on the appropriated regressions, for each hour of submersion for the IPR-139, Pérola and IPR-Tiziu cultivars, respectively (Table ).
Table 2. Germination and vigour classification of three bean cultivars seeds subjected to different submersion time periods.
The length (Table ) and dry weight (Table ) of the root, shoot and total value of the seedlings decreased as the submersion time in the water increased. The root length differed between the three cultivars only at two and four hours of submersion, where IPR-Tiziu and Pérola had longer roots than IPR-139 at two hours submersion, and Pérola had longer roots than the other cultivars at four hours submersion (Table ). The root dry mass of IPR-139 was always lowest (Table ). Similarly, differences in the shoot length between the cultivars were only evident at certain submersion times, in this case at one (Pérola was superior to IPR-Tiziu) and four hours submersion (Pérola was superior to the two other cultivars), as shown in Table . When the whole seedling was evaluated, Pérola maintained the longest seedlings at four hours submersion in comparison to the other cultivars (Table ). After submersion for one and two hours, Pérola and IPR-Tiziu had the highest total and shoot dry mass and after four hours Pérola was superior to the other two cultivars (Table ). In summary, IRP-139 always had the poorest vigour of the three cultivars and this was matched by equally poor germination during submersion. In contrast, the germination of Pérola was particularly high during the first four hours and this was associated with good vigour characteristics. However, by 16 h of submersion, all cultivars had similar length and mass measurements, suggesting that the strong germination of IPR-Tiziu at this time may be related to other characteristics. The ability to survive temporary oxygen deprivation is attributed to controlling energy use (Bailey-Serres & Voesenek, Citation2010; van Dongen et al., 2009). Anoxia has been related to protein synthesis inhibition, decrease in the ADH activity in the availability of ATP (Kato-Noguchi, Citation2001), the activation of pyruvate dehydrogenase, HSPs (heat shock proteins), CBL5 (calcineurin B-like-protein) and USP (universal stress protein) (Sadiq et al., Citation2011) and further increases in antioxidant enzyme activity during and after the stress release caused by flooding (Sairam et al., Citation2011). Therefore, biochemical components were analysed in the bean seeds and examined in relation to their performance under submersion.
Table 3. Root, shoot and total length of bean seedling from three cultivars subjected to different submersion time periods.
Table 4. Root, shoot and total dry mass of three bean cultivars seedlings subjected to different submersion time periods.
Biochemical traits during submersion
The leaching of ethanol into the imbibition water occurred after eight hours of submersion in the Pérola cultivar, and by 16 h for the other cultivars, with Pérola leaking a higher amount (Figure (C)). Nevertheless, ethanol was not detected in the seed tissues suggesting an induction of the fermentative pathway in the seed as a response to submergence (Bailey-Serres & Voesenek, Citation2008; Parent et al., Citation2008).
From the initial tests, IPR-Tiziu contained almost 420 μg of proline per gram of dry mass, 10 times higher than the other cultivars. Over 16 h submersion, IPR-Tiziu showed a decrease in the proline content, whereas Pérola increased but IPR-139 did not change (Figure (A)). Proline is a carbon source or sink during respiration (Szabados & Savouré, Citation2010) because proline can sustain the production of NADH / NADPH that is necessary to keep the cycle running with almost no oxygen (Kishor et al., Citation2005). Proline is also an osmolyte in seedlings and plants (Verbruggen & Hermans, Citation2008; He et al., Citation2012) and has antioxidant capacity (Szabados & Savouré, Citation2010).
Figure 2. Proline content (A) and SOD activity (B) of three bean cultivars subjected to different submersion period. Error bars are the standard deviation of three repetitions by cultivar and submersion period.
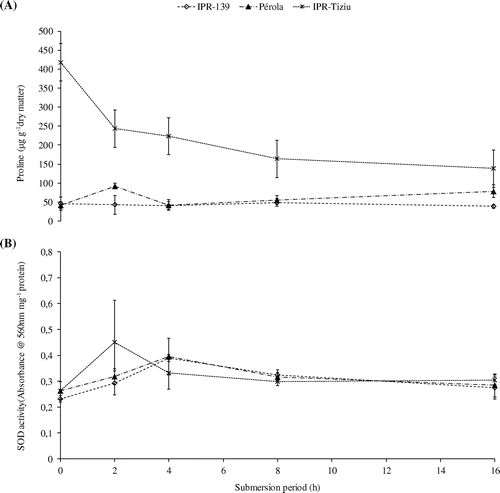
Antioxidants are an important defence to stressors including low oxygen environments. In 25-day-old Vigna, hypoxia induced an increase in the SOD activity, the APX and the GSR in tolerant genotypes (Sairam et al., Citation2011). In rice coleoptiles under aerobic or anaerobic conditions, the ROS-scavenging enzymes GST, APX cytosolic and putative GST were inhibited (Sadiq et al., Citation2011), and this response was interpreted as a form of signalling in response to the increasing ROS concentration in anoxic environments (Fukao & Bailey-Serres, Citation2004). In soybean under hypoxic conditions, there was also a reduction in the APX activity (Shi et al., Citation2008; Hashiguchi et al., Citation2009). Here, we examined SOD activity and found that values decreased in IPR-Tiziu after two hours of submersion and returned to the initial values after 8 or 16 h (Figure (B)). The other two cultivars showed an increased activity after four hours, returning to the original level after eight or 16 h. According to Kranner et al. (Citation2010) the SOD activity is often bell-shaped during stress, i.e. the activity is low when the stress is not so severe or in excess, and increases between these two points. In this study, the SOD activity pattern was a curve that was skewed to the right for all cultivars, but the peak of the activity was noticeable for IPR-Tiziu. In addition to proline concentration, SOD activity may be an early indicator of the tolerance among the cultivars during seed submersion.
Conclusion
Bean seeds are highly susceptible to submersion period in the tropical areas during the sowing season, what can lead to a complete fail in crop establishment. Tolerance to this stress should be linked directly to the available energy in the seed. This work hypothesised that proline could be an alternative route to overcome the energy depletion due to seed submersion using a model with three cultivars with different proline contents in the dry seed to test this.
The loss in germination and vigour was directly proportional to the seed submersion time. The one-hour submersion period was sufficient for germination differences to be exhibited among the cultivars. The consumption of proline during submersion enabled flooding tolerance by IPR-Tiziu, as this cultivar was less susceptible to damage and consequently more tolerant to submersion.
For the first time, it is shown that proline is an alternative to energy depletion caused by submersion of bean seeds. Further studies with more cultivars and landraces must be done to confirm these findings and the effectiveness of proline determination to help in select breeding parents and offspring lines as a characteristic of tolerance to flooding.
Disclosure statement
There were no conflicts of interest.
References
- Bailey-Serres, J., & Voesenek, L. A. C. J. (2008). Flooding stress: acclimations and genetic diversity. Annual Review of Plant Biology, 59, 313–339.10.1146/annurev.arplant.59.032607.092752
- Bailey-Serres, J., & Voesenek, L. A. C. J. (2010). Life in the balance: a signaling network controlling survival of flooding. Current Opinion in Plant Biology, 13, 489–494.10.1016/j.pbi.2010.08.002
- Bailey-Serres, J. Lee, S.C. and Brinton, E. (2012). Waterproofing crops: Effective flooding survival strategies. Plant Physiology, 160, 1698–1709.10.1104/pp.112.208173
- Banach, K., Banach, A. M., Lamers, L. P. M., Kroon, H., Bennicelli, R. P., Smits, A. J. M., & Visser, E. J. W. (2009). Differences in flooding tolerance between species from two wetland habitats with contrasting hydrology: Implications for vegetation development in future floodwater retention areas. Annals of Botany, 103, 341–351.
- Bewley, J. D., Bradford, K. J., Hilhorst, H. W. M., & Nonogaki, H. (2013). Seeds: Physiology of development, germination and dormancy. New York, NY: Springer.10.1007/978-1-4614-4693-4
- Bradford, M. M. (1976). A rapid and sensitive method for the quantitation of microgram quantities of protein utilising the principle of protein-dye binding. Anaytical Biochemistry, 72, 248–254.
- Brasil. (2009). Rules for Seed Analysis. Brasília: Ministério da Agricultura.
- Choudhary, A. K., Sultana, R., Pratap, A., Nadarajan, N., & Jha, U. C. (2011). Breeding for abiotic stresses in pigeonpea. Journal of Food Legumes, 24, 165–174.
- Custódio, C. C., et al. (2002). Effects in the germination and vigour of water submersion of bean seeds. Revista Brasileira de Sementes, 24, 49–54.10.1590/S0101-31222002000100009
- Custódio, C. C., Machado-Neto, N. B., Ito, H. M., & Vivan, M. R. (2009). Water submersion of bean seeds in the vigour evaluation. Revista Brasileirade Ciencias Agrarias, 4, 261–266.
- Ferreira, D. F. (2011). SISVAR: A computer statistical analysis system. Ciência e Agrotecnologia, 35, 1039–1042.
- Fukao, T., & Bailey-Serres, J. (2004). Plant responses to hypoxia – is survival a balancing act? Trends in Plant Science, 9, 449–456.10.1016/j.tplants.2004.07.005
- Hashiguchi, A., Sakata, K., & Komatsu, S. (2009). Proteome analysis of early-stage soybean seedlings under flooding stress. Journal of Proteome Research, 8, 2058–2069.10.1021/pr801051m
- He, Y., Zhang, J., Ma, H., Tu, Y., Chen, J., Chen, F., & Xue, X. (2012). Aerenchyma formation and increased accumulation of free proline in roots of xerophytic Aloe vera L. cultured in nutrient solutions. Journal of Medicinal Plants Research, 6, 243–252.
- Jackson, M. B., Ishizawa, K., & Ito, O. (2009). Evolution and mechanisms of plant tolerance to flooding stress. Annals of Botany, 103, 137–142.
- Kato-Noguchi, H. (2001). Anoxia tolerance and alcohol dehydrogenase activity in lettuce seedlings. Plant Growth Regulation, 33, 199–203.10.1023/A:1017558523090
- Kishor, P. K., Sangam, S., Amrutha, R. N., Laxmi, P. S., Naidu, K. R., Rao, K. R. S. S., & Sreenivasulu, N. (2005). Regulation of proline biosynthesis, degradation, uptake and transport in higher plants: Its implications in plant growth and abiotic stress tolerance. Current Science, 88, 424–438.
- Kranner, I., Minibayeva, F. V., Beckett, R. P., & Seal, C. E. (2010). What is stress? Concepts, definitions and applications in seed science. New Phytologist, 188, 655–673.10.1111/j.1469-8137.2010.03461.x.
- Lenssen, J. P. M., Menting, F. B. J., van der Putten, H. W., & Blom, C. W. P. M. (1999). Control of plant species richness and zonation of functional groups along a freshwater flooding gradient. Oikos, 86, 523–534.10.2307/3546656
- Machado-Neto, N. B., Custodio, C. C., Gatti, A. B., Priolli, M. R., & Cardoso, V. J. M. (2004). Proline: Use as an indicator of temperature stress in bean seeds. Cropp Breeding and Applied Biotechnology, 4, 330–337.10.12702/1984-7033
- Marcos Filho, J. (2005). Seed physiology of cultivated plants. Fealq: Piracicaba.
- Moriya, L. M., et al. (2015). Seed vigour better to be assessed by physiological markers rather than expression of antioxidant enzymes in the common bean (Phaseolus vulgaris L.). Australian Journal of Crop Science, 9, 30–40.
- Nakagawa, J. (1999). Vigour tests based in seedling development. In F. C. Krzyzanowski, et al. (Eds.), Vigor de sementes: Conceitos e testes Abrates: Londrina pp. 2.1–2.24.
- Parent, C., Capelli, N., Beger, A., Crevecoeur, M., & Dat, J. (2008). An overview of plant responses to soil waterlogging. Plant Stress, 2, 20–27.
- Sadiq, I., Fanucchi, F., Paparelli, E., Alpi, E., Bachi, A., Alpi, A., & Perata, P. (2011). Proteomic identification of differentially expressed proteins in the anoxic rice coleoptile. Journal of Plant Physiology, 168, 2234–2243.10.1016/j.jplph.2011.07.009
- Sairam, R. K., Dharmar, K., Lekshmy, S., & Chinnusamy, V. (2011). Expression of antioxidant defense genes in mung bean (Vigna radiata L.) roots under water-logging is associated with hypoxia tolerance. Acta Physiologiae Plantarum, 33, 735–744.10.1007/s11738-010-0598-3
- Saka, N., & Izawa, T. (1999). Varietal differences in the survival rate of sprouting rice seed (Oryza sativa L.) under highly reduced soil conditions. Plant Production Science, 2, 136–137.10.1626/pps.2.136
- Santosa, I. E., Ram, P. C., Boamfa, E. I., & Laarhoven, L. J. J. (2007). Patterns of peroxidative ethane emission from submerged rice seedlings indicate that damage from reactive oxygen species takes place during submergence and is not necessarily a post-anoxic phenomenon. Planta, 226, 193–202.10.1007/s00425-006-0457-z
- Shi, F., Yamamoto, R., Shimamura, S., Hiraga, S., Nakayama, N., Nakamura, T., & Komatsu, S. (2008). Cytosolic ascorbate peroxidase 2 (cAPX 2) is involved in the soybean response to flooding. Phytochemistry, 69, 1295–1303.10.1016/j.phytochem.2008.01.007
- Singh, D. P., & Singh, B. B. (2011). Breeding for tolerance to abiotic stresses in mungbean. Journal of Food Legumes, 24, 83–90.
- Szabados, L., & Savouré, A. (2010). Proline: A multifunctional amino acid. Trends in Plant Science, 15, 89–97.10.1016/j.tplants.2009.11.009
- van Dongen, J. T., Frohlich, A., Ramirez-Aguilar, S. J., Schauer, N., Fernie, A. R., Erban, A., … Geigenberger, P. (2009). Transcript and metabolite profiling of the adaptive response to mild decreases in oxygen concentration in the roots of Arabidopsis plants. Annals of Botany, 103, 269–280.
- Verbruggen, N., & Hermans, C. (2008). Proline accumulation in plants: A review. Amino Acids, 35, 753–759.10.1007/s00726-008-0061-6
- Vieira, R. D., & Krzyzanowski, F. C. (1999). Electrical conductivity test. In F. C. Krzyzanowski, et al. (Eds.), Vigor de sementes: Conceitos e testes (pp. 1–26). Londrina: Abrates.